Abstract
Background. Traditionally, radiation therapy plans are optimized without consideration of chemotherapy. Here, we model the risk of radiation pneumonitis (RP) in the presence of a possible interaction between chemotherapy and radiation dose distribution. Material and methods. Three alternative treatment plans are compared in 18 non-small cell lung cancer patients previously treated with helical tomotherapy; the tomotherapy plan, an intensity modulated proton therapy plan (IMPT) and a three dimensional conformal radiotherapy (3D-CRT) plan. All plans are optimized without consideration of the chemotherapy effect. The effect of chemotherapy is modeled as an independent cell killing process using a uniform chemotherapy equivalent radiation dose (CERD) added to the entire organ at risk. We estimate the risk of grade 3 or higher RP (G3RP) using the critical volume model. Results. The mean risk of clinical G3RP at zero CERD is 5% for tomotherapy (range: 1–18 %) and 14% for 3D-CRT (range 2–49%). When the CERD exceeds 9 Gy, however, the risk of RP with the tomotherapy plans become higher than the 3D-CRT plans. The IMPT plans are less toxic both at zero CERD (mean 2%, range 1–5%) and at CERD = 10 Gy (mean 7%, range 1–28%). Tomotherapy yields a lower risk of RP than 3D-CRT for 17/18 patients at zero CERD, but only for 7/18 patients at CERD = 10 Gy. IMPT gives the lowest risk of all plans for 17/18 patients at zero CERD and for all patients with CERD = 10 Gy. Conclusions. The low dose bath from highly conformal photon techniques may become relevant for lung toxicity when radiation is combined with cytotoxic chemotherapy as shown here. Proton therapy allows highly conformal delivery while minimizing the low dose bath potentially interacting with chemotherapy. Thus, intensive drug-radiation combinations could be an interesting indication for selecting patients for proton therapy. It is likely that the IMRT plans would perform better if the CERD was accounted for during optimization, but more clinical data is required to facilitate evidence-based plan optimization in the multi-modality setting.
Advanced imaging, treatment planning and delivery techniques have enabled highly conformal treatments, reducing dramatically the volume of normal tissue irradiated to high doses. However, these techniques redistribute dose so volumes receiving relatively low doses are increased. It is unclear exactly how these low dose volumes will influence the risk of toxicity. Moreover, modern cancer treatment often combines of radiotherapy and systemic treatment, and we lack modeling tools to analyze the possible interactions between these modalities.
As an example of this, among the first 46 patients treated with helical tomotherapy in an in-house single-arm phase I/II dose-per-fraction escalation trial, the incidence of grade 2 and 3 radiation pneumonitis (RP) was only 13% and 0%, respectively, despite treating large volume disease to very high equivalent doses [Citation1]. In contrast, Song et al. reported 7/37 cases (19%) with grade 3 or greater RP in 37 patients also treated with helical IMRT, but 24/37 patients had received concurrent and 13 had received neoadjuvant chemotherapy, illustrating the impact of chemotherapy [Citation2].
Recently, we demonstrated that if cytotoxic therapy acts as an independent cell killing process, the use of multimodality therapy will not only change the toxicity of treatment in a critical volume model, but also affect the relative merits of competing dose plans [Citation3]. Specifically, a simple dose plan without the low dose bath associated with intensity modulated photon RT (IMRT) may become less toxic than a more complex intensity modulated dose plan when a certain level of chemotherapy-related normal tissue damage is exceeded [Citation3].
Proton therapy allows delivery of highly conformal RT while minimizing the integral dose to non-target tissues [Citation4]. Consequently, proton therapy could potentially reduce the possible interaction between the low dose bath of IMRT and chemotherapy. Here, this proposition is examined in a modeling study comparing the predicted risk of radiation pneumonitis associated with proton therapy plans versus helical photon IMRT and 3D-CRT plans in the presence of chemotherapy thus extending our previous study of this effect [Citation3].
Material and methods
Eighteen patients with non-small cell lung cancer (NSCLC) previously treated at the University of Wisconsin were selected for this modeling study [Citation1]. These patients were the initial enrollees of a Phase I/II hypofractionation, dose-escalation study designed for patients who were not surgical candidates secondary to either medical comorbidities or advanced disease. Of the 18 patients, stage ranged from IIB to IV, with the majority being stage IIIA (six patients) and stage IIIB (seven patients).
Radiotherapy was delivered via helical tomotherapy and limited to the primary site and clinically proven or radiographically suspicious nodal regions. Elective nodal irradiation was prohibited. Planning imaging included a thin slice treatment planning CT and a 4D-CT. A custom-made double vacuum based immobilization system was utilized to reduce and normalize respiratory motion. The 4D-CT was acquired to identify the motion-defined envelope of the gross tumor volume (GTV). PET imaging was also utilized to define the extent of the GTV. A 6 mm margin was then added to the motion envelope to account for microscopic extension and set-up error, forming the PTV.
In addition to the clinically used tomotherapy plans, two plans were optimized for this modeling study: a 3D-CRT plan and a spot scanning intensity modulated proton therapy (IMPT) plan. The 3D-CRT and the tomotherapy plans are described in detail in [Citation3]. The IMPT plans were generated using Eclipse version 8.6 and the Varian Proton Convolution Superposition algorithm (version 8.6.15) with the generic beam data of the PT2 Varian Proton Therapy System. The machine has a span of proton energies of 70–250 MeV and a range shifter was introduced when appropriate. The IMPT plan was generated assuming freedom to irradiate from 360 degrees, but irradiation from anterior angles where the respiratory motion can be expected to change the effective path length to the target was avoided. Typically, two to three beam directions were used for the plans. All plans were normalized to deliver exactly 60 Gy to 95% of the target volume.
After planning, the dose volume histograms were converted into 2 Gy equivalent doses using an α/β ratio of 4 Gy for the lung (for the endpoint of radiation pneumonitis) and 10 Gy for the tumor as described in detail in [Citation5]. The critical volume model was used to estimate the risk of radiation pneumonitis associated with a given dose distribution [6,7]. In short, the critical volume model assumes that the organ at risk consists of a number of independent functional subunits (FSUs). The probability of damaging the FSU is estimated by a sigmoid function of the local dose. The volume of damaged FSUs can then be calculated from the dose distribution. Finally, the volume of damaged lung and the risk of clinical overt RP is linked by a second sigmoid function. In the present work, the tolerance of the FSUs was assumed to be 20 Gy with an infinite steepness of the local dose-response relationship – hence the volume of the lung exposed to 20 Gy or more is assumed to be damaged and the volume of lung exposed to a lower dose is assumed to retain function after RT. The normal tissue complication probability, NTCP, is estimated from the volume of damaged lung, fdam, using the logistic function:
where β0 and β1 are fitting parameters. We use β0 = −5.2 and β1 = 13.3 based on the fitted function linking fdam to clinical grade 3 RP in a previous publication [Citation8]. Note that the fitting parameters, β0 and β1, define the position and steepness of the sigmoid link between the damaged volume and the risk of clinical RP, but that the link function is monotonous. As a consequence, the ranking of dose plans is determined by the value of fdam alone; independent of the values of β0 and β1. The modeling is further described in [Citation3]. When calculating the risk of RP, the organ at risk was defined as both lungs minus the PTV and the effect of chemotherapy was modeled as an independent cell killing process by adding a chemotherapy equivalent radiation dose (CERD) in 2-Gy fractions to all voxels in the organ at risk.
The mean NTCP for the 18 patients was calculated for each plan with CERD varied from 0 Gy (no effect of chemotherapy) to 20 Gy (chemotherapy dose equal to FSU tolerance dose). The uncertainty of the mean values was estimated by bootstrap resampling whereby 5000 simulated groups of 18 patients in each were randomly drawn from the original population with replacement. The Monte Carlo 68% confidence interval of the mean (approximately corresponding to ±1 S.D.) was then estimated from the 5000 samples as the 16th to 84th percentile of the simulated means. Statistical comparisons between plans were performed as two-tailed Wilcoxon signed rank sum tests in SPSS version 15.0.
Results
shows the main dose-volume metrics for the three plan types for CERD = 0 and 10 Gy along with the mean and range of the NTCP estimates. displays a representative example of the intensity modulated plans in a single patient. Note that the tomotherapy plan is highly conformal, with only the target receiving the prescribed 60 Gy. The tomotherapy plan, however, is also associated with a large volume of the lung exposed to low doses. In contrast, the IMPT plan is equally conformal to the target but is not associated with the extensive low dose volume as the contralateral lung distal to the target seen from the direction of the beam receives no appreciable dose.
Figure 1. An illustrative case of the intensity modulated photon plan (left) and proton plan (right). The Tomotherapy plan provides very high conformality at the expense of large low dose volumes of the lung. The proton IMPT plan, however, provides conformal target coverage with very little exposure of the residual lung. The bold purple line is the target volume prescribed to 60 Gy.
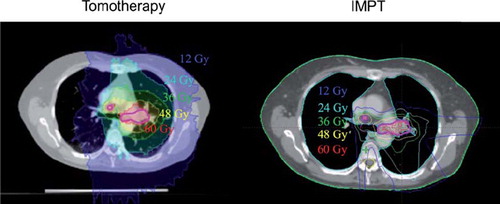
Table I. Mean and range of dose-volume metrics for the three types of dose plans and the mean and range of the NTCP as calculated with the critical volume model with D50 = 20 Gy and k = infinity. CERD, chemotherapy equivalent radiation dose; MLD, mean lung dose; Vx, volume of lung receiving x Gy. All values are corrected for fractionation with α/β = 4 Gy for the residual lung. Note that V20 is monotonically correlated with NTCP. Also, V10 with CERD = 0 Gy is equal to V20 at CERD = 10 Gy.
shows the resulting NTCP as calculated from the critical volume model for the three studied dose plans as a function of CERD. Note, that without chemotherapy, i.e. at zero CERD, the tomotherapy plans are superior to the 3D-CRT plans, whereas the proton plans are the best of all three plans. However, However, as CERD increases, the low dose associated with the tomotherapy plans become penalized, as shown in our previous study [Citation3]. This results in a steep increase in NTCP as with increasing CERD. In contrast, the 3D-CRT curve is flatter. Intuitively, this reflects that only volumes exposed to less than 20 Gy can change from undamaged to damaged in the critical volume model as CERD increases. The 3D-CRT plans only expose limited volumes to such low doses and are therefore relatively insensitive to reasonable values of CERD. Similarly, the proton plans are not associated with any appreciable low dose volume, and only the fraction of the lung between the beam entrance and the target are exposed to low doses. Consequently, the favorable ranking of the proton therapy dose plans is preserved as CERD increases.
Figure 2. The estimated risk of radiation pneumonitis versus chemotherapy equivalent dose using the critical volume model. The solid lines indicate average risk and the dotted lines indicate the 68% confidence interval of the mean as derived by bootstrap resampling.
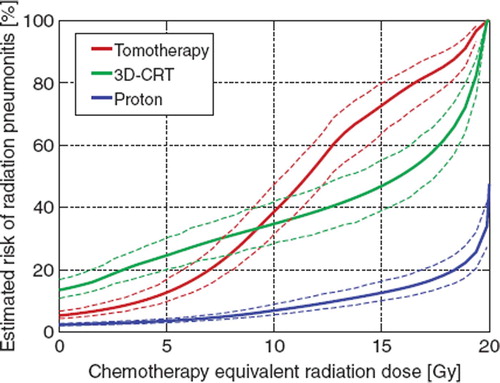
Tomotherapy gives a smaller risk of RP than 3D-CRT for 17/18 patients at zero CERD. When CERD exceeds 9 Gy, however 3D-CRT is on average less toxic. With CERD = 10Gy, 3D-CRT gives a smaller risk of RP for 11/18 patients when compared to tomotherapy. Proton therapy yields the lowest risk of all plans for 17/18 patients at zero CERD (maximum sparing of RP risk compared to best photon plan: 9 percent points, p = 0.001) and for all patients with CERD = 10 Gy (maximum sparing of RP risk: 26 percent points, p < 0.001).
Discussion
A simple additive model was chosen here to represent the effect of combining chemotherapy and radiation. Alternative models could be proposed, for example models where chemotherapy is assumed to modify the local effect of radiation dose, i.e. (local) radiation sensitization. While increased toxicity of chemoradiation compared to RT alone has been documented in flurry of studies, there are very few published studies with data allowing discrimination between different models of drug-radiation interactions. One study reported dose-response curves for subcutaneous fibrosis after postmatectomy radiotherapy over a range of doses with and without cyclophosphamide, methotrexate and 5-fluorouracil (CMF). Different models of the effect of chemotherapy were considered. There was no evidence for repair inhibition after RT+CMF. Attempts to model the increased risk of normal-tissue injury by means of a dose-modifying effect of CMF produced a significantly poorer fit to the data than a model with an additive effect of chemotherapy [Citation9]. Although generalizations to other normal tissues and chemotherapy schedules are problematic there is to the best of our knowledge no relevant analysis for RP. We therefore chose the simplest possible assumption of additive, independent effects. It should be noted, however, that the value of CERD = 10 Gy, where plan ranking changes, is consistent with reported clinical data on RP incidence after radiation plus adjuvant chemotherapy [Citation1].
Proton therapy is more sensitive to anatomic changes and motion effects than photon therapy. Consequently, more generous margins CTV to PTV margins are often used for proton therapy [Citation10,Citation11]. In the present study, we applied the same margins for photon and proton therapy, which may influence the comparison of NTCP, especially at zero CERD. As the normal lung was defined as lung tissue outside the PTV, we used the same PTV margin for both plans. Alternatively, one could have defined the normal lung as the lung outside the photon PTV and then have added the additional expanded margin for the proton plan. While this would have increased the volume of lung exposed to high doses in the proton plan, the absence of the low dose bath means that the relative insensitivity to CERD will be preserved. Furthermore, we note that state-of-the-art motion management can minimize the problem of motion, also for protons [Citation12], although several issues still make respiratory gating and tracking particularly difficult with protons [Citation13].
If reliable chemo-radiation dose-effect models were available, IMRT plans could be optimized with the drug-radiation effect taken explicitly into account. Thereby, photon IMRT plans could likely compare favorably with 3D-CRT, or at least do equally well as the optimizer would ultimately drive the IMRT plan towards the 3D-CRT plan if the latter was really optimal. Unfortunately, the lack of accurate clinical parameter estimates means that the model proposed here and in [Citation3] should be considered hypothesis generating and cannot be used for clinical plan optimization. The present study is intended as an explorative, proof-of-principle study.
In conclusion, interaction between radiation dose distribution and administration of chemotherapy might influence the relative merits of competing dose plans and optimizing the radiation plan separately cannot be assumed to produce the best possible treatment plan in a multimodality setting. Using proton therapy, however, could potentially minimize the undesired interaction between the modalities with respect to normal tissue tolerance by avoiding the low dose bath associated with modern, conformal photon therapy.
Acknowledgements
ISV is supported by The Lundbeck Foundation Center for Interventional Research in Radiation Oncology (CIRRO) and The Danish Council for Strategic Research. SMB acknowledges support from the National Cancer Institute grant no. 2P30 CA 014520-34.
Declaration of interest: Mackie, TR has ownership interest in Tomotherapy Inc, Mehta, MP has served as a consultant for Tomotherapy and holds stock options in Tomotherapy.
References
- Adkison JB, Khuntia D, Bentzen SM, . Dose escalated, hypofractionated radiotherapy using helical tomotherapy for inoperable non-small cell lung cancer: Preliminary results of a risk-stratified phase I dose escalation study. Technol Cancer Res Treat 2008;7:441–7.
- Song CH, Pyo H, Moon SH, Kim TH, Kim DW, Cho KH. Treatment-related pneumonitis and acute esophagitis in non-small-cell lung cancer patients treated with chemotherapy and helical tomotherapy. Int J Radiat Oncol Biol Phys 2010;78:651–8.
- Vogelius IS, Westerly DC, Cannon GM, Mackie TR, Mehta MP, Sugie C, Bentzen SM. Intensity-modulated radiotherapy might increase pneumonitis risk relative to three-dimensional conformal radiotherapy in patients receiving combined chemotherapy and radiotherapy: A modeling study of dose dumping. Int J Radiat Oncol Biol Phys In Press, Corrected Proof, Available online 7 April 2011, ISSN 0360-3016, DOI: 10.1016/j.ijrobp.2010.12.073.(http://www.sciencedirect.com/science/article/B6T7X-52JWFGR-B/2/73278d0755c82c2304ee811cdbb234d4).
- Nystrom H. The role of protons in modern and biologically-guided radiotherapy. Acta Oncol 2010;49:1124–31.
- Vogelius IS, Westerly DC, Cannon GM, Bentzen SM. Hypofractionation does not increase radiation pneumonitis risk with modern conformal radiation delivery techniques. Acta Oncol 2010;49:1052–7.
- Jackson A, Kutcher GJ, Yorke ED. Probability of radiation-induced complications for normal tissues with parallel architecture subject to non-uniform irradiation. Med Phys 1993; 20:613–25.
- Niemierko A, Goitein M. Modeling of normal tissue response to radiation: The critical volume model. Int J Radiat Oncol Biol Phys 1993;25:135–45.
- Yorke ED, Jackson A, Rosenzweig KE, Braban L, Leibel SA, Ling CC. Correlation of dosimetric factors and radiation pneumonitis for non-small-cell lung cancer patients in a recently completed dose escalation study. Int J Radiat Oncol Biol Phys 2005;63:672–82.
- Bentzen SM, Overgaard M, Thames HD, Christensen JJ, Overgaard J. Early and late normal-tissue injury after postmastectomy radiotherapy alone or combined with chemotherapy. Int J Radiat Biol 1989;56:711–5.
- Moyers MF, Miller DW, Bush DA, Slater JD. Methodologies and tools for proton beam design for lung tumors. Int J Radiat Oncol Biol Phys 2001;49:1429–38.
- Engelsman M, Kooy HM. Target volume dose considerations in proton beam treatment planning for lung tumors. Med Phys 2005;32:3549–57.
- af Rosenschold PM, Aznar MC, Nygaard DE, . A treatment planning study of the potential of geometrical tracking for intensity modulated proton therapy of lung cancer. Acta Oncol 2010;49:1141–8.
- Knopf A, Bert C, Heath E, . Special report: Workshop on 4D-treatment planning in actively scanned particle therapy – recommendations, technical challenges, and future research directions. Med Phys 2010;37:4608–14.