Abstract
Purpose. The purpose of this study was to compare treatment plans generated using fixed beam Intensity Modulated photon Radiation Therapy (IMRT), inversely optimized arc therapy (RapidArc(R), RA) with spot-scanned Intensity Modulated Proton Therapy (IMPT) for high-grade glioma patients. Plans were compared with respect to target coverage and sparing of organs at risk (OARs), with special attention to the possibility of hippocampus sparing. Method. Fifteen consecutive patients diagnosed with grade III and IV glioma were selected for this study. The target and OARs were delineated based on computed tomography (CT), FDG-positron emission tomography (PET) and T1-, T2-weigted, and Diffusion Tensor Imaging (DTI) magnetic resonance imaging (MRI) and fiber-tracking. In this study, a 6 MV photon beam on a linear accelerator with a multileaf collimator (MLC) with 2.5 mm leaves and a spot-scanning proton therapy machine were used. Two RA fields, using both a coplanar (clinical standard) and a non-coplanar, setup was compared to the IMRT and IMPT techniques. Three and three to four non-coplanar fields where used in the spot-scanned IMPT and IMRT plans, respectively. The same set of planning dose-volume optimizer objective values were used for the four techniques. The highest planning priority was given to the brainstem (maximum 54 Gy) followed by the PTV (prescription 60 Gy); the hippocampi, eyes, inner ears, brain and chiasm were given lower priority. Doses were recorded for the plans to targets and OARs and compared to our clinical standard technique using the Wilcoxon signed rank test. Result. The PTV coverage was significantly more conform for IMPT than the coplanar RA technique, while RA plans tended to be more conform than the IMRT plans, as measured by the standard deviation of the PTV dose. In the cases where the tumor was confined in one cerebral hemisphere (eight patients), the non-coplanar RA and IMPT techniques yielded borderline significantly lower doses to the contralateral hippocampus compared to the standard (22% and 97% average reduction for non-coplanar RA and IMPT, respectively). The IMPT technique allowed for the largest healthy tissue sparing of the techniques in terms of whole brain doses and to the fiber tracts. The maximum doses to the chiasm and brainstem were comparable for all techniques. Conclusion. The IMPT technique produced the most conform plans. For tumors located in the one of the cerebral hemispheres, the non-coplanar RA and the IMPT techniques were able to reduce doses to the contralateral hippocampus. The IMPT technique offered the largest sparing of the brain and fiber tracts. RA techniques tended to produce more conform target doses than IMRT.
Malignant glioma have a characteristically aggressive growth pattern including necrotic foci, proliferating blood vessels and migrating tumor cells [Citation1]. The patients have a poor prognosis with median survival times between 4 to 18 months following surgery plus chemoradiation, and depending on prognostic factors such as age and Karnofsky score [Citation2–4]. The median survival for glioblastoma patients (WHO grade IV) have remained more or less constant in the past three decades, despite a considerable technological advancement in radiation therapy imaging, computer-aided planning and delivery. However, a modest improvement of the median survival was reported by Stupp et al. [Citation5] with the introduction of concomitant chemo- and radiation therapy, with a minority of patients surviving beyond three to five years. A reduction of long-term neurotoxicity using intensity modulated radiation therapy (IMRT) was seen in a retrospective analysis [Citation6]. WHO grade III glioma may have longer survival times depending on histological subtype [Citation7], than patients with glioma grade IV. We also note with interest that some evidence has emerged suggesting that the subventricular zone may need to be given a certain dose, hypothetically in order to sterilize tumor stem cells [Citation8].
With a small group of long-term surviving glioma patients the reduction of long-term treatment-related side effects and improving quality of the remaining life becomes increasingly important. A number of treatment planning studies have shown the superiority of the IMRT technique to conventional radiation therapy techniques; i.e. three-dimensional conformal radiation therapy (3D-CRT) [Citation6,Citation9–12]. Recently, the IMRT technique was compared to inversely optimized rotational therapy; i.e. the RapidArc® (RA) technique [Citation13,Citation14]. In these studies, it was found that RA compared favorably to IMRT with respect to sparing of organs at risk (OARs) and in the reduction of the number of monitor units. It was also noted that it is of interest to study the effect of using non-coplanar RA fields.
In this study, we compare the radiation therapy techniques IMRT, coplanar and non-coplanar RA, and Intensity Modulated Proton Therapy (IMPT) for patients with malignant glioma. The clinical implementation of the RA technique was pioneered at our institution [Citation15–18]. Our hypothesis is that the IMPT and RA techniques will offer significant sparing of organs at risk compared to fixed beam IMRT. We are particularly interested in the potential of reducing doses to the hippocampi, which have been demonstrated to be of importance in the neurocognitive dysfunction for patients receiving cranial irradiation [Citation19,Citation20].
Methods
Patients and scan data
Fifteen consecutive patients treated for high-grade glioma (WHO grades III and IV) at our institution were selected for this study. An aqua-plastic mask system attached to a carbon-fiber frame was used as fixation. The patients were scanned using positron emission tomography using (18F)-fluorodeoxyglucose (FDG-PET) and computed tomography (CT) in the same session. In addition, T1- and T2-weighted magnetic resonance imaging (MRI) scans were performed with Gadovist® contrast (Bayer HealthCare AG, Germany). Five of the 15 patients were also scanned using MRI diffusion tensor imaging (DTI), which was used for three-dimensional (3D) mapping of white matter fiber tracts (tractography) [Citation21]. All scans were fused in the iPlan treatment planning system (version 4.1, BrainLab AG, Germany).
Organs at risk were delineated using the automation tools available in the iPlan system, including the eyes, lenses, brainstem, hippocampus, optic chiasm, optical nerves, inner ear, and fiber tracts objects based on the tractography mapping. The delineations of the OARs were subsequently preliminary corrected by a radiographer, and then finally reviewed, corrected and approved by the clinical radiation oncologist in charge of the treatment. No Planning Risk Volumes (PRV) were used for the OARs in this study. Fiber tracking was performed using the iPlan treatment planning software, and the fibers were subsequently transformed 3D fiber objects volumes in the iPlan system.
The Gross Tumor Volume (GTV) was defined based primarily on the contrast CT and MRI scans, while the FDG-PET and fiber tracts were only qualitatively evaluated. The Clinical Target Volume (CTV) equaled the GTV plus 2 cm margin in 3D (though respecting bony anatomy), and the Planning Target Volume (PTV) equaled the CTV plus 2 mm margin in 3D.
Treatment planning
Four inversely optimized treatment planning strategies were applied: coplanar RapidArc® (RA), non-coplanar RA, non-coplanar fixed-beam Intensity Modulated photon Radiation Therapy (IMRT) and spot-scanned Intensity Modulated Proton Therapy (IMPT). Two arc fields and three to four non-coplanar static fields were used for the RA and IMRT techniques, respectively. The collimator was set at 45° and 315° complimentary angles for the RA fields and at 2° for the IMRT fields. The sliding window IMRT technique was used. Three non-coplanar fields where used in the proton plans. For each patient, the same set of planning objectives (i.e. priorities) were used for the four techniques in order to maintain the same level of plan modulation. The PTV was prescribed 60 Gy, but the brainstem was given higher priority than the PTV in the optimization; the maximum dose was limited to 54 Gy centrally for the brainstem (higher point doses were allowed). The doses to the other OARs were minimized, but were given lower priority than the PTV. The list of priorities were as follows: brainstem 250 (maximum (max) 54 Gy), body 200 (max 62 Gy), PTV 175 (max 61 Gy), PTV 175 (minimum (min) 59 Gy), hippocampus 75 (or 5 if inside the PTV; max 10 Gy), eyes 90 (max 2 Gy), chiasm 75 (max 54 Gy) and the Normal Tissue Objective (NTO) at 150.
A 6 MV photon beam with a high-definition Multi-Leaf Collimator (MLC) (Novalis Tx) machine implemented in the Eclipse (ver. 8.9, Varian Medical Systems, US) treatment planning system was used in all instances, except for the proton plans, where the PT2 Proton Therapy System was used.
For the proton plans, the radiation absorbed dose was multiplied by a factor of 1.1 to obtain the radiobiological equivalence of a cobalt-60 source; often denoted as “cobalt-equivalent Gray” (denoted as CGy, GyE or Gy-Eq). A non-coplanar technique was used in this study, and using an isocentric field setup. The proton therapy machine has a span of proton kinetic energies of 70–250 MeV. Considering that many of the targets had a proximal depth of less than the 4.1 g/cm2 that corresponds 70 MeV, we therefore introduced a water-equivalent 57 mm range shifter in the beam in case of need. Use of the range shifter affects the energy straggling. Dosimetrically, the range shifter will slightly increase the lateral spread of the beam and result in a blurring of the beam spots [Citation22]. A range shifter of 57 mm was the only choice available in the system. The IMPT plans were optimized on a 5 mm spot size grid in the direction of the beam axis as well as in the orthogonal plane, see [Citation23] for details.
Minimum, average and maximum doses to the PTV, and OARs; hippocampus (dex/sin), eyes (dex/sin), optic chiasm, fiber objects, and brainstem were recorded in this study. The standard deviation of the dose to the PTV was calculated as a measure of PTV dose conformity, as suggested by Yoon et al. [Citation24].
Statistics
The clinical co-planar RA technique was compared to non-coplanar RA and IMPT, that represents potentially new techniques for clinical use, and to the previously used IMRT technique. Specifically, the standard deviation of the dose to the PTV, the average dose to the whole brain, the average dose to the contra-lateral hippocampus (in case of a target in one cerebral hemisphere) and the highest of the average doses to the eyes were compared for the set of plans. A Wilcoxon signed rank test was used. A two-tailed 0.05 significance level was used. The Bonferroni correction was applied to account for repeated testing (12 tests in total) and, consequently, a two-tailed p-value of p < 0.0042 was considered significant. Analyse-it ver. 2.22 were used for the statistical analysis (and compared with SPSS ver. 15.0).
Results
An example patient MRI with contrast is shown in , illustrating the use of multiple image data sets in the delineation of glioma patients. While contrast enhanced T1 weighted MRI primarily localize areas with leaking vasculature and increased blood volume, FDG-PET visualize metabolic activity in viable tumor tissue. In , a transversal CT slice example is shown with fiber tract objects overlaid centered on the tumor in the left cerebral hemisphere as well as a tractography image.
Figure 1. Transversal images through the level of the basal ganglia of a patient with glioblastoma multiforme. The T1 weighted MR after contrast injection (left image) show a central area in the left temporal lobe with contrast enhancement. The metabolic activity in temperature scale using FDG-PET (right image). The tumor region – the large red region with the highest uptake – extends far beyond the MR contrast enhanced area in all directions, as shown in the fused images (centre image).
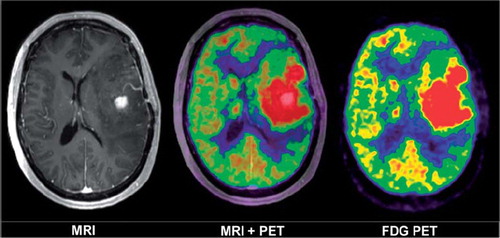
Figure 2. Left: transversal CT image with overlaid tractography structure in gold. The hippocampi and brainstem are displayed in purple and green, respectively. Right: a 3D fiber tract structure seen anteriorly with eyes in red and green for the same patient. Fibers are displayed in red, green and blue in the images. The color coding is by convention red for commissural (transversal) tracts, blue for fibers running superoinferiorly and green for fibers running in ventralodorsaly. The fibers were disrupted and displaced by the tumor, as indicated by the lack of symmetry in both images.
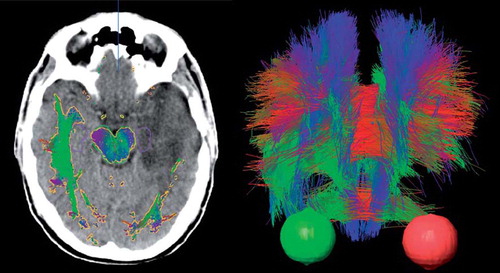
The highest of the average doses to the eyes were significantly lower for both the IMRT (2.1 Gy, p < 0.0001) and IMPT (0.2 Gy, p < 0.0001) plans compared to the standard coplanar RA technique (3.9 Gy), which was achieved using carefully selected beam directions. The maximum doses to the chiasm and brainstem were comparable for all techniques; the average of organ at risk doses for the 15 patients are presented in .
Figure 3. Doses to the organs at risk specifically evaluated in this study. Average doses for the 15 patients (Fiber Objects were averaged for 5 patients) are presented.
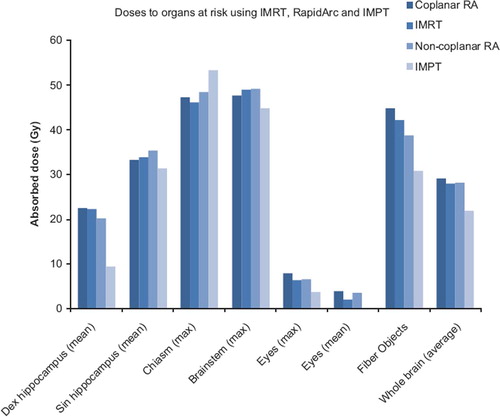
The PTV coverage was significantly more conform for the for IMPT (p < 0.0001) than the standard two field coplanar RA technique. The standard technique tended to have a more conform target coverage than IMRT (not significant; p = 0.034) and was comparable to non-coplanar RA technique (see ). In case of a tumor located in one of the cerebral hemispheres (eight patients), the non-coplanar RA and IMPT techniques yielded a borderline significantly (both at p = 0.0078) lower dose to the contralateral hippocampus compared to the standard (22% and 97% average reduction for non-coplanar RA and IMPT, respectively).
Figure 4. The ranges of the standard deviations of the absorbed doses to the Planning Target Volumes for the Intensity Modulated photon Radiation Therapy (IMRT), coplanar RapidArc (RA), non-coplanar RA and Intensity Modulated Proton Therapy (IMPT) techniques are shown as lines in the diagram. The extent of the boxes represent the range of the mid third of the standard deviations for each radiation therapy technique.
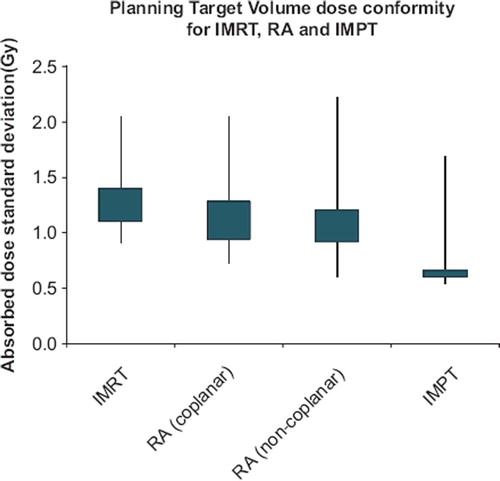
The IMPT technique allowed for the largest healthy tissue sparing of the techniques in terms of whole brain doses; this is illustrated in a set of example dose distribution for one patient, shown in . The average dose to the whole brain (including the target volume) was significantly lower for IMPT than co-planar RA (p < 0.0001); the three photon techniques were all comparable in this respect.
Discussion
In this treatment planning study we show that our current practice was dosimetrically comparable to the previous, i.e. co-planar RA fields compared to IMRT, but allows for a simpler setup of the patients without involving a rotation of the treatment couch. A further dosimetric improvement from the current practice of coplanar RA fields was possible in selected cases with the tumor located in one cerebral hemisphere. Here, the non-coplanar RA technique leads to a somewhat reduced doses to the contralateral hippocampus.
Previous works have shown a dosimetric advantage in terms of maximum doses to organs at risk as well as target conformality using advanced photon therapy techniques such as IMRT [Citation25]. In addition, previous planning studies the IMRT technique were shown it to be equal or superior to RA in terms of target coverage [Citation26,Citation27]. In this study, however, the coplanar RA plans tended to have a superior PTV coverage compared to the IMRT plans, though not at the statistically significant level (p = 0.034) when applying the Bonferroni correction. This was perhaps due to the differences in the number of IMRT and RA fields used in this vs. previous studies; e.g. we used two RA fields and only three to four IMRT fields in this study, which matched our clinical practice, compared to a single RA field in previous works [Citation26,Citation27]. Also, planning priorities and/or software versions appear to be significantly dissimilar.
In the present work we show that further improvements from the photon therapy techniques can be made, both in terms of target coverage and in terms of sparing of most organs at risk, but particularly the contralateral hippocampus, for the whole brain and the fiber tracts, by using IMPT. The risk of radiation induced necrosis increases with the irradiated brain volume [Citation28]. To our knowledge, dose-response data for fiber tracts are presently limited to a single study involving the pyramidal tract and radiosurgery [Citation29]. Tractography scan data was recently shown to correlate with neurocognitive decline in children treated with crainospinal irradiation [Citation30]. Neurocognitive decline causing deteriorating quality of life within the time-frame of one to four months has been observed in the palliative radiation treatment of the whole brain, i.e. for doses of 30 Gy. This early neurocognitive decline is reflected primarily by loss of verbal function and short-term memory recall [Citation31]. The preservation of quality of life for glioma patients was recently suggested to be the main focus for the design of coming clinical trials [Citation32]. IMPT may be considered for the patient group given the potential to spare eloquent centers, as demonstrated herein.
The PTV margin was small in this study (2 mm) and based on the daily image guidance performed in the clinical radiation treatments using the ExacTrac imaging and 6D position corrections using the Robotics couch systems (BrainLab AG). The PTV margin was the same in both the photon and proton therapy plans, though in reality the uncertainties related to the proton range might have justified a slightly larger margin for IMPT.
In the present work we selected a set of planning priorities values and used them in all plans. The planning was therefore automated and allowed no user interaction. Results will therefore be biased towards the selection of priority values, and the assumption was that the selection was clinically relevant. The method was chosen in favor of evaluating the plans actually used to treat the patients since we would then run a substantial risk that the differences found will simply be a result of planner experience and competence, planning time, and differences in priority of organs entered in the optimizer. The method of using the same priority values does not however rule out the possibility of results being influenced by differences in the interpretation of the optimizers for IMRT, RA and IMPT, as separate algorithms were implemented in and called by the same system (Eclipse). It is our opinion that the method chosen in this work will tend to demonstrate differences inherent to the techniques, which was the aim to clarify in present study. A promising but yet experimental way of handling the comparison of systems with multiple parameter input is the Pareto concept; see for example [Citation33]. The actual parameters set were not specifically including the whole brain, the fiber tracts, inner ear, etc, but the normal tissue objective operates on all tissue outside the target and thus these structures as well, at least in part. Adopting the priority list used in the planning of glioma patients in this work for other patients at other centers appear unwise, given potential differences in diagnostic imaging, target delineation, image guidance, treatment margin selection and radiation delivery equipment and protocols.
In conclusion, in this work we study the dosimetric differences in photon and proton therapy plans for malignant gliomas, imaged using CT and FDG-PET, T1 and T2 MRI, and five of 15 with DTI MRI and fiber tracking. The intensity modulated proton therapy technique produced the most conform treatment plans and provided the lowest irradiation of the whole brain and fiber tracts. For tumors located in one of the cerebral hemispheres, the non-coplanar RapidArc and the intensity modulated proton therapy techniques resulted in reduced doses to the contra-lateral hippocampus. The RapidArc techniques tended to produce more conform target doses than intensity modulated photon radiation therapy, though with slightly elevated but likely clinically insignificant doses to the eyes.
Acknowledgements
The authors acknowledge Thomas Carlslund and Mikael Olsen for IT support.
Declaration of interest: Per Munck af Rosenschöld and Svend Aage Engelholm have research and educational collaboration agreements with Varian Medical Systems, Palo Alto, US. There are no conflict of interest regarding the current study. The study was conducted from scientific interest.
References
- McLendon RE, Rich JN. Glioblastoma stem cells: A neuropathologist's view. J Oncol 2011;2011:397195.
- Salford LG, Brun A, Nirfalk S. Ten-year survival among patients with supratentorial astrocytomas grade III and IV. J Neurosurg 1988;69:506–9.
- Gilbert MR, Friedman HS, Kuttesch JF, Prados MD, Olson JJ, Reaman GH, . A phase II study of temozolomide in patients with newly diagnosed supratentorial malignant glioma before radiation therapy. Neuro Oncol 2002;4:261–7.
- Curran WJ, Jr., Scott CB, Weinstein AS, Martin LA, Nelson JS, Phillips TL, . Survival comparison of radiosurgery-eligible and -ineligible malignant glioma patients treated with hyperfractionated radiation therapy and carmustine: A report of Radiation Therapy Oncology Group 83-02. J Clin Oncol 1993;11:857–62.
- Stupp R, Mason WP, van den Bent MJ, Weller M, Fisher B, Taphoorn MJ, . Radiotherapy plus concomitant and adjuvant temozolomide for glioblastoma. N Engl J Med 2005;352:987–96.
- Narayana A, Yamada J, Berry S, Shah P, Hunt M, Gutin PH, . Intensity-modulated radiotherapy in high-grade gliomas: Clinical and dosimetric results. Int J Radiat Oncol Biol Phys 2006;64:892–7.
- Shirai K, Suzuki Y, Okamoto M, Wakatsuki M, Noda SE, Takahashi T, . Influence of histological subtype on survival after combined therapy of surgery and radiation in WHO grade 3 glioma. J Radiat Res (Tokyo) 2010;51:589–94.
- Evers P, Lee PP, DeMarco J, Agazaryan N, Sayre JW, Selch M, . Irradiation of the potential cancer stem cell niches in the adult brain improves progression-free survival of patients with malignant glioma BMC. Cancer 2010;10:384.
- Thilmann C, Zabel A, Grosser KH, Hoess A, Wannenmacher M, Debus J. Intensity-modulated radiotherapy with an integrated boost to the macroscopic tumor volume in the treatment of high-grade gliomas. Int J Cancer 2001;96:341–9.
- MacDonald SM, Ahmad S, Kachris S, Vogds BJ, DeRouen M, Gittleman AE, . Intensity modulated radiation therapy versus three-dimensional conformal radiation therapy for the treatment of high grade glioma: A dosimetric comparison. J Appl Clin Med Phys 2007;8:47–60.
- Hermanto U, Frija EK, Lii MJ, Chang EL, Mahajan A, Woo SY. Intensity-modulated radiotherapy (IMRT) and conventional three-dimensional conformal radiotherapy for high-grade gliomas: Does IMRT increase the integral dose to normal brain? Int J Radiat Oncol Biol Phys 2007;67:1135–44.
- Chan MF, Schupak K, Burman C, Chui CS, Ling CC. Comparison of intensity-modulated radiotherapy with three-dimensional conformal radiation therapy planning for glioblastoma multiforme. Med Dosim 2003;28:261–5.
- Wagner D, Christiansen H, Wolff H, Vorwerk H. Radiotherapy of malignant gliomas: Comparison of volumetric single arc technique (RapidArc), dynamic intensity-modulated technique and 3D conformal technique. Radiother Oncol 2009;93:593–6.
- Shaffer R, Nichol AM, Vollans E, Fong M, Nakano S, Moiseenko V, . A comparison of volumetric modulated arc therapy and conventional intensity-modulated radiotherapy for frontal and temporal high-grade gliomas. Int J Radiat Oncol Biol Phys 2010;76:1177–84.
- Aznar MC, Petersen PM, Logadottir A, Lindberg H, Korreman SS, Kjaer-Kristoffersen F, . Rotational radiotherapy for prostate cancer in clinical practice. Radiother Oncol 2010;97:480–4.
- Kjaer-Kristoffersen F, Ohlhues L, Medin J, Korreman S. RapidArc volumetric modulated therapy planning for prostate cancer patients. Acta Oncol 2009;48:227–32.
- Korreman S, Medin J, Kjaer-Kristoffersen F. Dosimetric verification of RapidArc treatment delivery. Acta Oncol 2009;48:185–91.
- Fredh A, Korreman S, Munck af Rosenschold P. Automated analysis of images acquired with electronic portal imaging device during delivery of quality assurance plans for inversely optimized arc therapy. Radiother Oncol 2010;94:195–8.
- Abayomi OK. Pathogenesis of irradiation-induced cognitive dysfunction. Acta Oncol 1996;35:659–63.
- Monje ML, Mizumatsu S, Fike JR, Palmer TD. Irradiation induces neural precursor-cell dysfunction. Nat Med 2002; 8:955–62.
- Sanghvi DA. Recent advances in imaging of brain tumors. Indian J Cancer 2009;46:82–7.
- Pedroni E, Scheib S, Bohringer T, Coray A, Grossmann M, Lin S, . Experimental characterization and physical modelling of the dose distribution of scanned proton pencil beams. Phys Med Biol 2005;50:541–61.
- Munck af Rosenschold P, Aznar MC, Nygaard DE, Persson GF, Korreman SS, Engelholm SA, . A treatment planning study of the potential of geometrical tracking for intensity modulated proton therapy of lung cancer. Acta Oncol 2010;49:1141–8.
- Yoon M, Park SY, Shin D, Lee SB, Pyo HR, Kim DY, . A new homogeneity index based on statistical analysis of the dose-volume histogram. J Appl Clin Med Phys 2007;8: 9–17.
- Amelio D, Lorentini S, Schwarz M, Amichetti M. Intensity-modulated radiation therapy in newly diagnosed glioblastoma: A systematic review on clinical and technical issues. Radiother Oncol 2010;97:361–9.
- Shaffer R, Nichol AM, Vollans E, Fong M, Nakano S, Moiseenko V, . A comparison of volumetric modulated arc therapy and conventional intensity-modulated radiotherapy for frontal and temporal high-grade gliomas. Int J Radiat Oncol Biol Phys 2010;76:1177–84.
- Wagner D, Christiansen H, Wolff H, Vorwerk H. Radiotherapy of malignant gliomas: Comparison of volumetric single arc technique (RapidArc), dynamic intensity-modulated technique and 3D conformal technique. Radiother Oncol 2009;93:593–6.
- Lawrence YR, Li XA, El Naqa I, Hahn CA, Marks LB, Merchant TE, . Radiation dose-volume effects in the brain. Int J Radiat Oncol Biol Phys 2010;76(3 Suppl):S20–7.
- Maruyama K, Kamada K, Ota T, Koga T, Itoh D, Ino K, . Tolerance of pyramidal tract to gamma knife radiosurgery based on diffusion-tensor tractography. Int J Radiat Oncol Biol Phys 2008;70:1330–5.
- Mabbott DJ, Noseworthy MD, Bouffet E, Rockel C, Laughlin S. Diffusion tensor imaging of white matter after cranial radiation in children for medulloblastoma: Correlation with IQ. Neuro Oncol 2006;8:244–52.
- Gondi V, Tome WA, Mehta MP. Why avoid the hippocampus? A comprehensive review. Radiother Oncol 2010;97:370–6.
- Henriksson R, Asklund T, Poulsen HS. Impact of therapy on quality of life, neurocognitive function and their correlates in glioblastoma multiforme: A review. J Neurooncol Epub 2011 Apr 6.
- Ottosson RO, Engstrom PE, Sjostrom D, Behrens CF, Karlsson A, Knoos T, . The feasibility of using Pareto fronts for comparison of treatment planning systems and delivery techniques. Acta Oncol 2009;48:233–7.