Abstract
Introduction. The concern of secondary cancer induction and normal tissue complications have motivated a more frequent use of protons in radiotherapy (RT) of children. However, proton RT is likely to be less robust to anatomical changes occurring during therapy. In this study we present a recent clinical case to illustrate this issue. Material and methods. A five-year-old boy with a highly proliferating malignant intracranial nerve sheath tumour underwent a partial resection prior to RT and developed a post-surgery oedema close to the surgical cavity. RT was delivered with volumetric modulated arc therapy (VMAT) to a total tumour dose of 61.2 Gy. The most critical organs at risk (ORs) were the right optical nerve, brainstem and chiasm. Proton plans were constructed for the purpose of this study. In order to simulate a worst-case scenario, the extent of the oedema observed in the last part of the treatment was used to modify the oedema on the planning computed tomography (CT). Both the photon and proton plans were then re-calculated, as follows: Scenario A: Treatment planning based on the planning CT with oedema and dose calculated as if it was delivered without oedema. Scenario B: Treatment planning on the modified planning CT without oedema, but re-calculated with oedema. These two scenarios were compared to the situation where the oedema was present at treatment planning and unchanged during RT. Results. Total dose to critical ORs remained unchanged for the photon plans, with changes within 0.3 Gy for the normal tissues and nearly identical target coverage. For protons, scenario A led to increased maximal doses in all critical ORs, 5.1 Gy in the brainstem, 6.1 Gy in the chiasm and 6.4 Gy in the right optical nerve. For scenario B the proton plans resulted in a loss in target coverage. Conclusion. This case study shows that RT with protons were far less robust to anatomical changes than when treated with photons, emphasising the increased need for adaptive approaches in RT with protons.
Radiotherapy (RT) of brain tumours is challenging because of the close vicinity of normal structures that need to be spared to avoid often disabling long-term consequences that will affect the patient's quality of life. When irradiating children the immaturity of the structures at risk and its often devastating consequences is a special concern. RT is often necessary in the treatment of central nervous malignancies and is often given with curative intent. Brain cancers are the most common solid tumours in children.
Due to the favourable depths-dose characteristics and the subsequent lower risk for secondary malignant neoplasms [Citation1], proton beam RT is ideal for treatment of children. The integral dose in proton therapy is often two to three times lower compared to photon therapy [Citation2]. However, the very same characteristics that make protons attractive also make proton beams more sensitive to changes in the patient anatomy during RT.
In this paper we present a recent clinical paediatric case to illustrate and discuss the issue of plan robustness to anatomical changes in photon and proton beam RT. The child was treated for a malignant brain tumour in close vicinity to organs at risk. A subdural oedema due to postoperative complications, which regressed during the course of therapy, was already present on the planning CT. In this study we have evaluated whether changes in the oedema would be disadvantageous when using a proton or a photon plan while irradiating this patient. We evaluated the dosimetric changes in the target and the organs at risk (ORs) assuming the oedema was shrinking (scenario A) or progressing (scenario B) during the treatment course, assuming no action was taken for the anatomical changes.
Material and methods
A five-year-old boy was after operation for a left frontal tumour referred to our centre for RT. The histological diagnosis was a central malignant peripheral nerve sheath tumour invading into the brain parenchyma [Citation3], with a high proliferation index of 95%. A partial resection was performed, but re-growth was observed on MRI two weeks after surgery (). A second craniotomy had been performed shortly after the first operation due to postoperative complications and development of an extensive oedema. During re-operation part of the cranial bone was removed on the left side to relieve for the increased intracranial pressure.
Figure 1. MR scanning for fusion with dose planning CT slice showing the tumour close to the organs at risk.
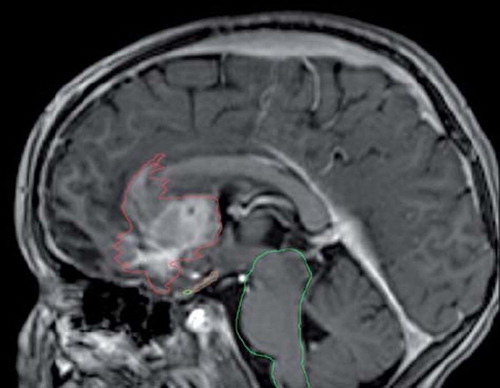
Photon treatment course delivered
RT was delivered with a Trilogy linear accelerator (Varian Medical Systems, Palo Alto, CA, USA) using 6 MV photon beams with volumetric modulated arc therapy (VMAT). Treatment was planned using the Eclipse treatment planning system (v.10, Varian Medical Systems). VMAT was chosen over conventional IMRT in order to minimise leakage radiation from the accelerator [Citation4–6] (). Due to urgent need for consolidation, the child was first treated with a dose of 36 Gy in 22 fractions to the GTV, defined as the residual tumour and resection cavity, with a 2 cm margin (CTV 2 cm), followed by a more complex simultaneous integrated boost (SIB) of 19.8–25.2 Gy in 14 fractions; the lower boost dose was given to the tumour with 1 cm margin (CTV 1 cm) and the higher dose to the tumour only (CTV 0 cm). Thus, the total target dose was 61.2 Gy in 36 fractions. The most critical ORs were the right optical nerve, the brainstem and the chiasm. Daily low-dose CBCT was acquired prior to delivery of each treatment fraction.
Anatomical changes during the course of radiotherapy
Already on the planning computed tomography (CT) a large subdural right frontal oedema was present. During the course of RT, the oedema varied in size, with a maximal deviation (normal to the skull) of 8 mm. In order to compare the robustness of the photon and proton therapy, two extreme scenarios of anatomical changes were suggested.
Scenario A: Planning performed on the original planning CT with oedema and re-calculated on a modified planning CT without oedema.
Scenario B: Planning performed on the modified planning CT without oedema and re-calculated on the original planning CT with oedema.
In order to construct the modified planning CT, the planning CT was co-registered to a CBCT scan acquired in the last treatment phase. The body contour from the CBCT scan was copied to the planning CT and used for the calculation ().
Retrospective proton beam RT planning
For the purpose of this study, separate intensity-modulated proton therapy (IMPT) plans for both the first and the SIB phase were constructed using the Eclipse system (Eclipse v. 10.0.28 Varian Medical Systems). The proton plans were optimised to give the same target coverage as the photon plans as well as having the same constraints on the maximum doses to the chiasm, brainstem and right optical nerve. Proton planning was performed using active scanning beam technique (). The spot size (sigma), the spot spacing in scan direction and the spacing between scanning lines were 5 mm. Simultaneous spot optimisation was used as optimisation method and the Proton Convolution Superposition algorithm (v 10.0.25) were used for dose calculations for the proton plans.
Results
Proton therapy reduced the integral dose to surrounding normal tissue, in particular in the medium and low dose regions. In this case the V30 Gy for the normal brain tissue (outside the CTV) was reduced from 224 cm3 with VMAT to 176 cm3 with IMPT, at the V15 Gy a reduction from 576 cm3 to 354 cm3 was obtained. Dose volume histograms of the three targets and the three ORs are presented in for the planned treatment and the two scenarios A and B for the VMAT and the IMPT course, respectively. The target coverage is nearly unchanged for the VMAT course and only minor changes are seen in the DVHs of the ORs for the VMAT treatment (). Regarding the changes for the IMPT course, the changes in the DVHs for the ORs are substantial, with an increase of 6 Gy for the chiasm, optic nerve and brain stem in scenario A, whereas scenario B results in a decreased target coverage for all the three targets (). Maximal doses to ORs and the percentages of the target covered with 95% of the prescribed dose are summarised in for the planned treatment courses as well as the two scenarios A and B.
Figure 5. Dose Volume histograms for the VMAT course A: the three targets and B: the three ORs and for the IMPT course C: the three targets and D: the three ORs, for the planned treatment as well as changes according to Scenarios A and B.
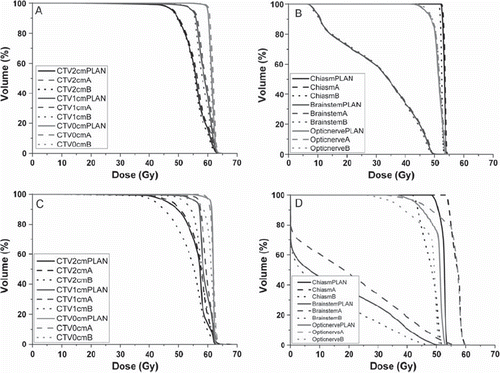
Table I. Comparison of planned treatment versus scenario A and B, for VMAT and IMPT treatment courses.
Discussion
The present study has illustrated that proton therapy and more specifically IMPT can be less robust to anatomical changes during the course of therapy than corresponding highly conformal photon plans. In this specific case the vicinity of the tumour and Bragg peaks close to critical anatomical structures such as the optical chiasm, right optical nerve and brainstem caused severe over-dosage of these structures if the oedema shrinks during the treatment course (scenario A). This is due to the finite and fixed radiologic range of the proton pencil beams that in the planning geometry will stop before the critical structures but in reality during treatment will reach these structures. Conversely, target under-dosage is the consequence when using protons if the oedema grows during the course (scenario B).
Both courses of anatomy changes simulated in this study are worst case scenarios, in fact for several reasons. The scenarios were constructed by comparing treatment planning outcome for the smallest versus the largest observed size of the oedema. In the actual case the oedema was gradually changing size from the planning CT and throughout the treatment course – shrinking in the beginning and then growing again. A more realistic scenario would probably not have shown the same extreme changes in the DVH parameters. Another issue is the selected beam configuration. One beam had an anterior-superior direction that might have been a logical choice in absence of the oedema. This field enters directly through the oedema and the position of the Bragg peak is therefore shifted accordingly. Two beams were used in both phases of the treatment but a larger number of beams spread out in angular spaces might have smeared out the effect of range changes and increased robustness at the expense of normal tissue sparing. Also different field configurations in the first and boost phases of the course might have increased the robustness of the IMPT plans.
IMPT has also previously been shown to be sensitive to uncertainties in proton range [Citation7–10]. These uncertainties will originate from several sources like patient setup, CT image quality, Hounsfield Unit to stopping power conversion, target motion and like in this case, anatomical changes. To increase the robustness of proton plans the treatment margins could be enlarged. In our clinical case this would have been difficult as there were critical ORs close to the target that needed to be spared and that could thus overlap with the treatment field. Another method of handling daily anatomical changes may be addressed by robust or risk adapted planning as described by several authors [Citation11–13]. This may account for minor anatomical variations or setup uncertainties, but for major changes adaptive proton therapy seems to be the solution. Routine repeat CT scanning could be used to observe for anatomical changes during the treatment course, and then act (i.e. re-plan) when required [Citation14]. However, even minor daily intra-fractional anatomic changes could also contribute to range uncertainties and these would still not be monitored with conventional CT scanning prior to beam delivery. With the increasing demand for patient positioning precision in our quest for tighter treatment margins, cone-beam CT scanners are now in widespread clinical use for photon radiotherapy. Daily CBCT can monitor anatomical changes during a long course of radiotherapy [Citation15]. In this case, we have estimated the dose to the cranial part of the head from the whole course using daily CBCT to be less than 0.3 Gy, which we found clinically acceptable taking the advantages connected to the image information into account. Adaptive planning strategies using daily CBCT for photon therapy have been proposed [Citation16–18] as well as methods to increase the imaging quality of the CBCT and to use it better for re-planning purposes [Citation19–21]. The same development is to be expected for proton facilities as well. Even though the quality of CBCT images is inferior to diagnostic CT and hardly sufficient to use for re-planning in proton therapy, anatomical changes registered on the CBCT might still serve as indicators for re-planning.
Specific clinical situations could require repeat magnetic resonance imaging (MRI) during the course of proton therapy. MRI provides improved soft tissue contrast compared to CT, which could facilitate routine adaptive RT. A prototype integrating a 6 MV accelerator with an MRI scanner has been described. This concept could be equally interesting for protons as the accuracy of the images might more easily be used for routine plan adaptation [Citation22,Citation23].
In conclusion, our case illustrates that protons might be less robust to anatomical changes than photons and although we have chosen a worst case scenario this could have consequences for the clinical outcome with either an increased risk for side effects or a decreased tumour control probability. This should be taken into consideration when planning complex targets adjacent to multiple critical structures in cases with high probability for anatomical changes that could increase the range uncertainty. Adaptive approaches could be used to decrease the problem.
Declaration of interest: The authors report no conflicts of interest. The authors alone are responsible for the content and writing of the paper.
References
- Athar PS, Paganetti H. Comparison of second cancer risk due to out-of-field doses from 6 MV IMRT and proton therapy based on 6 pediatric treatment plans. Radiother Oncol 2011;98:87–92.
- Lomax AJ, Bortfeld T, Goitein G, Debus J, Tercier PA, Coucke PA, . A treatment planning inter-comparison of protons and intensity modulated photon therapy. Radiother Oncol 1999;51:257–71.
- Chen L, Mao Y, Chen H, Zhou LF. Diagnosis and management of intracranial malignant peripheral nerve sheath tumors. Neurosurgery 2008;62:825–32.
- Zhang P, Happersett L, Hunt M, Jackson A, Zelefsky M, Mageras G. Volumetric modulated arc therapy: Planning and evaluation for prostate cancer cases. Int J Radiat Oncol Biol Phys 2010;76:1456–62.
- Shaffer R, Nichol AM, Vollans E, Fong M, Nakano S, Moiseenko V, . A comparison of volumetric modulated arc therapy and conventional intensity-modulated radiotherapy for frontal and temporal high-grade gliomas. Int J Radiat Oncol Biol Phys 2010;76:1177–84.
- Wagner D, Christiansen H, Wolff H, Vorwerk H. Radiotherapy of malignant gliomas: Comparison of volumetric single arc (RapidArc), dynamic intensity-modulated technique and 3 D conformal technique. Radiother Oncol 2009;93:593–6.
- Lomax AJ. Intensity modulated proton therapy and its sensitivity to treatment uncertainties 1: The potential effects of calculational uncertainties. Phys Med Biol 2008;53:1027–42.
- Lomax AJ. Intensity modulated proton therapy and its sensitivity to treatment uncertainties 2: The potential effects of inter-fraction and inter-field motions. Phys Med Biol 2008; 53:1043–56.
- Albertini F, Bolsi A, Lomax AJ, Rutz HP, Timmermann B, Goitin G, . Sensitivity of intensity modulated proton therapy plans to changes in patient weight. Radiother Oncol 2008;86:187–94.
- Soukup M, Söhn M, Yan D, Liang J, Alber M. Study of robustness of IMPT and IMRT for prostate cancer against organ movement. Int J Radiat Oncol Biol Phys 2009;75:941–9.
- Unkelbach J, Bortfeld T, Martin BC, Soukup M. Reducing the sensitivity of IMPT treatment plans to setup errors and range uncertainties via probabilistic treatment planning. Med Phys 2009;36:149–63.
- Pflugfelder D, Wilkens JJ, Oelfke U. Worst case optimization: A method to account for uncertainties in the optimization of intensity modulated proton therapy. Phys Med Biol 2008;53:1689–700.
- Albertini F, Hug EB, Lomax AJ. The influence of the optimization starting conditions on the robustness of intensity-modulated proton therapy plans. Phys Med Biol 2010;55: 2863–78.
- Hui Z, Zhang X, Starkschall G, Li Y, Mohan R, Komaki R, . Effects of interfractional motion and anatomic changes on proton therapy dose distribution in lung cancer. Int J Radiat Oncol Biol Phys 2008;72:1385–95.
- Knap MM, Hoffmann L, Nordsmark M, Vestergaard A. Daily cone-beam computed tomography used to determine tumor shrinkage and localisation in lung cancer patients. Acta Oncol 2010;49:1077–84.
- Vestergaard A, Søndergaard J, Petersen JB, Høyer M, Muren LP. A comparison of three different adaptive strategies in image-guided radiotherapy of bladder cancer. Acta Oncol 2010;49:1069–76.
- Kron T, Wong J, Rolfo A, Pham D, Cramb J, Foroudi F. Adaptive radiotherapy for bladder cancer reduces integral dose despite daily volumetric imaging. Radiother Oncol 2010;97:485–7.
- Lütgendorf-Caucig C, Fotina I, Stock M, Pötter R, Goldner G, Georg D. Feasibility of CBCT-based target and normal structure delineation in prostate cancer radiotherapy: Multi-observer and image multi-modality study. Radiother Oncol 2011;98:154–61.
- Elstrøm UV, Wysocka BA, Muren LP, Petersen JB, Grau C. Daily kv cone-beam CT and deformable image registration as a method of studying dosimetric consequences of anatomic changes in adaptive IMRT of head and neck cancer. Acta Oncol 2010;49:1101–8.
- Søvik Å, Rødal J, Skogmo HK, Lervåg C, Eilertsen K, Malinen E. Adaptive radiotherapy based on contrast enhanced cone beam CT imaging. Acta Oncol 2010;49:972–7.
- Buhl SK, Duun-Kristensen AK, Christensen BH, Behrens CF. Clinical evaluation of 3D/3D MRI-CBCT automatching on brain tumors for online patient setup verification – A step toward MRI-based treatment planning. Acta Oncol 2010; 49:1085–91.
- Raaymakers BW, Lagendijk JJ, Overweg J, Kok JG, Raaijmakers AJ, Kerkhof EM, . Integrating a 1.5 T MRI scanner with a 6 MV accelerator: Proof of concept. Phys Med Biol 2009;54:N229–37.
- Raaymakers BW, Raaijemakers AJE, Lagendijk JJW. Feasibility of MRI guided proton therapy: Magnetic field dose effects. Phys Med Biol 2008;53:5615–22.