Abstract
Background. Implementation of cone beam computed tomography (CBCT) in frameless stereotactic body radiotherapy (SBRT) of lung tumours enables setup correction based on tumour position. The aim of this study was to compare setup accuracy with daily soft tissue matching to bony anatomy matching and evaluate intra- and inter-fractional translational and rotational errors in patient and target positions. Material and methods. Fifteen consecutive SBRT patients were included in the study. Vacuum cushions were used for immobilisation. SBRT plans were based on midventilation phase of four-dimensional (4D)-CT or three-dimensional (3D)-CT from PET/CT. Margins of 5 mm in the transversal plane and 10 mm in the cranio-caudal (CC) direction were applied. SBRT was delivered in three fractions within a week. At each fraction, CBCT was performed before and after the treatment. Setup accuracy comparison between soft tissue matching and bony anatomy matching was evaluated on pretreatment CBCTs. From differences in pre- and post-treatment CBCTs, we evaluated the extent of translational and rotational intra-fractional changes in patient position, tumour position and tumour baseline shift. All image registration was rigid with six degrees of freedom. Results. The median 3D difference between patient position based on bony anatomy matching and soft tissue matching was 3.0 mm (0–8.3 mm). The median 3D intra-fractional change in patient position was 1.4 mm (0–12.2 mm) and 2.2 mm (0–13.2 mm) in tumour position. The median 3D intra-fractional baseline shift was 2.2 mm (0–4.7 mm). With correction of translational errors, the remaining systematic and random errors were approximately 1°. Conclusion. Soft tissue tumour matching improved precision of treatment delivery in frameless SBRT of lung tumours compared to image guidance using bone matching. The intra-fractional displacement of the target position was affected by both translational and rotational changes in tumour baseline position relative to the bony anatomy and by changes in patient position.
Stereotactic body radiation therapy (SBRT) has proven a promising alternative to surgery for patients with early stage non-small cell lung cancer or solitary metastases in the lungs [Citation1–3]. In SBRT, high doses are delivered in a few treatment fractions and therefore high precision in target localisation and hence treatment delivery is of great importance [Citation4]. When delivering SBRT for lung lesions, respiratory-induced tumour motion represents an important uncertainty in target localisation [Citation5]. Breathing related variations in tumour position appear both intra- and inter-fractionally. Intra-fraction variation describes the variation in tumour position during one treatment fraction, manifested as both tumour motion during the breathing cycle and baseline shifts [Citation6,Citation7]. The patient moving on the couch during treatment affects intra-fractional tumour position as well. Inter-fraction variation describes the variations between fractions, e.g. baseline shifts [Citation8,Citation9].
Until recently the image guidance for SBRT of tumours in the lungs at our clinic was performed using two orthogonal setup kV-images and online match was based on bony anatomy. This implied uncertainty since the position of the tumour relatively to the bony anatomy can change in the period from simulation to treatment due to baseline shifts [Citation8,Citation9]. Furthermore, it has been shown [Citation10], that two-dimensional (2D) planar imaging introduces additional uncertainty in matching due to difficulties in interpretation of the image data compared to volumetric images.
Recent implementation of cone beam computed tomography (CBCT) at our clinic enabled setup correction for SBRT based on tumour position. Since CBCT acquisition takes approximately one minute, the image data includes several breathing cycles, resulting in a smeared out image of the tumour providing additional information on tumour motion extent.
The purpose of this study is to examine the improvement in tumour targeting accuracy in frameless SBRT with daily CBCT image guidance with soft tissue matching compared to bony anatomy matching. Furthermore, we will evaluate the extent of intra-fractional changes in patient and target position; both translational and rotational errors will be assessed.
Material and methods
Fifteen consecutive patients, treated with SBRT for peripheral lung tumours from May 2010 to January 2011 were included in the study. The patients’ characteristics are presented in .
Table I. Patient characteristics.
Data collection for this project was approved by the Danish Data Protection Agency (j.nr. 30-0484).
Planning scans
According to our routine clinical protocol for SBRT planning, all patients underwent a positron emission tomography (PET)/CT-scan (on BiographTM 16, Siemens Medical Solutions), a 4DCT scan and a deep inspiration breath hold (DIBH) CT-scan (both performed on Sensation Open, Siemens Medical Solutions). 4DCT was performed within one to two days after the PET/CT. The DIBH, acquired immediately after the 4DCT, was performed to ensure that tumour volume was not over- or underestimated due to artefacts in the scan eventually selected for planning [Citation11]. For all scanning procedures, patients were immobilized with their arms above their head in a VacFix® vacuum cushion and a knee support.
A respiratory correlated scan was acquired in a helical scanning mode during free breathing. The respiratory signal was tracked and recorded with the Real-time Position ManagementTM 1.8 system (Varian® Medical Systems). The 4DCT with 10 phases was reconstructed using phase-binning. The CT images had pixel size 0.98 mm × 0.98 mm, and 2 mm plane separation.
Peak-to-peak tumour motion in all directions was assessed from the 4DCT images. In case the motion was 5 mm or more in any direction, the midventilation bin was chosen for the treatment planning [Citation12]. Otherwise the CT part of the PET/CT scan was used, as contrast enhancement was available on the PET/CT images, but not on the 4DCT images.
EclipseTM version 8.9 (Varian® Medical Systems) was used for target delineation and treatment planning. The gross tumour volume (GTV) delineation was performed multi-disciplinarily based on all image data. The GTV to clinical target volume (CTV) margin was zero. Standard CTV to planning target volume (PTV) margins of 5 mm in the transversal plane and 10 mm in the cranio-caudal (CC) direction were applied [Citation5]. All patients were treated with 6 MV photons with five to seven coplanar beams on a Trilogy® linear accelerator (Varian Medical Systems) with 6D Robotic couch (Brainlab). The SBRT was delivered in three 15 Gy fractions within a week.
On-line image guidance
Prior to each treatment fraction the patient was aligned with room lasers to the skin tattoos, according to our clinical routine. A CBCT was performed as the image guidance and matched with the planning CT, using automatic soft tissue match. Volume of interest (VOI) for the match included the PTV with a small individually defined margin (1–2 cm). The matching was performed only for the translational corrections since the technical possibility to correct for the rotational errors with the use of robotic couch was not yet evaluated for SBRT patients. The automatic match was evaluated visually and corrected if necessary. All translational errors were corrected for prior to the treatment. A second CBCT was performed immediately after the treatment delivery.
Off-line image registration and data analysis
For the study, rigid image-registrations were performed off-line using six degrees of freedom, hence giving a possibility to evaluate both translational and rotational errors. Matching was performed with the automatic tools in Aria Off-line Review (Varian® Medical Systems). The CBCT resolution was 1.172 mm × 1.172 mm and 2.5 mm plane separation. The high contrast resolution measured on our CBCT was approximately 0.7 mm. The centre of rotations corresponded to the treatment plan isocentre, which was placed approximately in the middle of the GTV. Use of VOI limited the search area for the automatic matching; VOI was defined as “GTV + 1 cm” when applying soft tissue tumour matching or the whole CBCT volume in case of bone matching. All matches were manually evaluated and if necessary corrected by a single observer (MJ).
First, a soft tissue match of the pretreatment CBCT with the planning CT was performed. Differences in translational displacements between the on-line and off-line match were used as a measure of residual positional error, when rotational errors are not corrected for. We evaluated the extent of rotational errors in order to justify a possible use of the robotic couch for further improvement of the positioning uncertainties.
Differences between bone match and soft tissue tumour match of the pretreatment CBCT with the planning CT were used as a measure of improvement in positioning uncertainty compared to our earlier clinical practice, when bone matching was used based on a set of orthogonal images.
The post-treatment CBCT scans were matched with the planning CT-scans in the same way using both soft tissue match and bone match. Differences between pre- and post-treatment soft tissue matches provided a measure of intra-fractional uncertainty in tumour position, while the differences between the pre- and post-treatment bone matches provided a measure of intra-fractional stability in patient position. Furthermore, we evaluated the extent of intra-fractional changes in tumour position relative to the bones (also referred to as tumour baseline shift) by analysing the deviation between pre- and post-treatment differences between soft tissue and bone match of CBCT.
Differences between the matches were presented as 3D vector displacement of the isocentre. Since the centre of rotations corresponded to the isocentre, rotations did not affect the calculation of the 3D displacement vector. Hence the 3D vector was calculated as (x2 + y2 + z2)½, with x representing the displacement in anterior-posterior (AP), y the CC and z the left-right (LR) direction.
Statistical analysis
All tumour and patient position differences, quantified as defined above for both translational and rotational directions, were expressed in terms of group mean (M), systematic (∑) and random errors (ς) as defined in [Citation13]. In brief, mean and standard deviation (SD) were calculated for each patient's and each target's displacement. M is mean value of all mean values, ∑ is estimated from SD of all mean values and ς is, due to limited number of measurements for each patient, obtained from root mean square (RMS) of all individual SD.
To investigate possible correlations between the treatment duration and changes in tumour position, patient position and baseline shift, linear regression analysis with calculation of a correlation coefficient (R2) was performed.
Results
Due to technical issues with implementation of the new imaging strategy at our department, CBCT was obtained both before and after the treatment in only 33 of overall 45 treatment fractions. In the remaining 12 fractions only the pretreatment CBCT was obtained.
Residual positional error after online correction
By correcting soft tissue tumour match on-line for translational errors only, a systematic error of around 1 mm (1 SD) in all directions remained; the random error was equal or slightly larger. Ninety seven percent of rotational errors were below 4° and 91% below 3° in the single treatment fractions. In one third of the patients mean rotational errors between 2° and 3° were observed in either roll, pitch or rotation, while the systematic error of the population was 1.2°–1.4° ().
Table II. Residual positioning error when correcting for three degrees of freedom compared to six degrees of freedom, i.e. when rotational errors are not corrected for.
Soft tissue versus bony anatomy match
The median 3D difference between target position based on bony anatomy matching and soft tissue matching was 3.0 mm (0–8.3 mm) for all fractions. The largest difference was seen for the tumour with the largest motion (20 mm in CC), however the second largest difference was seen for a tumour with motion of less than 4 mm. For the population as a whole, the systematic error in CC direction was much larger than in LR and AP direction (2.4 mm vs. 1.2 mm and 1.2 mm; 1 SD), while the random error was around 1 mm in all directions.
The rotational differences between bone match and soft tissue tumour match were below 3° in 93% of fractions (range 0°–4.3°). The largest systematic rotational error introduced by using bone match instead of soft tissue tumour match on a 6D robotic couch was 2.8° in a single patient. However, the systematic and random errors of the population as a whole were 1.0°–1.4° and 0.7°–1.2° (1 SD), respectively. These errors were largest around the roll axis ().
Table III. Errors introduced when using bone match as the image guidance instead of soft tissue tumour match.
There was no apparent trend of increasing difference between bony anatomy match and soft tissue tumour match throughout the treatment course.
Intra-fractional uncertainties in tumour and patient position
The median 3D difference between pre- and post-treatment soft tissue matched CBCT (i.e. assessing changes in tumour position) was 2.2 mm (range 0–13.2 mm) for all fractions, resulting in a population systematic error of 1.3–1.5 mm (1 SD). Both the systematic and random rotational intra-fractional errors were below 1.1° (1 SD) ().
Table IV. Intra-fractional differences in tumour and patient position and baseline shift.
The median 3D difference between pre- and post-treatment bone matched CBCT (i.e. assessment of changes in patient position) was 1.4 mm (range 0–12.2 mm) for all treatment fractions, resulting in a population systematic error of 0.9–1.6 mm (1 SD). The rotational systematic and random errors were below 0.35° (1 SD) ().
Baseline shift
The median 3D deviation between differences in soft tissue and bone match of pre- and post-treatment CBCT (i.e. assessing baseline shift) was 2.2 mm (range 0–4.7 mm) for all treatment fractions. The largest translational systematic and random errors due to baseline shift were in AP direction (1.7 mm and 1.6 mm, respectively), the baseline rotational errors were comparable to the rotational errors in intra-fractional target position (). In , 3D baseline shift as a function of tumour motion in CC is presented. Linear regression analysis resulting in the correlation coefficient (R2) of 0.06 does not imply any correlation between the extent of tumour motion and 3D baseline shift.
Figure 1. Three-dimensional baseline shift as a function of tumour motion in cranio-caudal direction: linear regression analysis does not indicate any baseline shift dependence on peak-to-peak tumour motion.
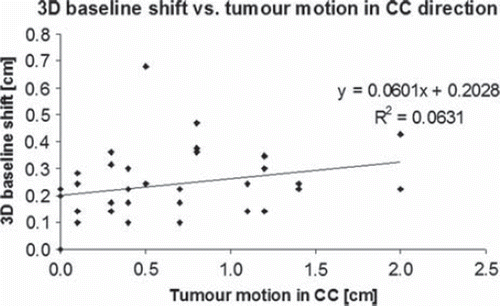
Average time between the pre- and post-treatment CBCT was 18:30 min (SD 3:37 min). The correlation coefficient (R2) between intra-fractional 3D changes in patient position, tumour position and baseline shift and the duration of treatment fraction was close to zero (< 0.01, 0.02 and 0.01, respectively), indicating no time dependence on intra-fractional changes. As an example, 3D changes in baseline shift as a function of fraction duration are illustrated in .
Discussion
In the present study, the precision of delivering frameless SBRT increased substantially with daily CBCT image guidance in combination with soft tissue matching. For half of the patients this improvement was at least 3 mm in any direction in any fraction. Improvement in target localisation with soft tissue has been shown previously [Citation10,Citation14,Citation15]. The median 3D difference between bone match and soft tissue match in our study was smaller by more than a factor of 2 compared to the study by Purdie et al. [Citation10], even though the patients in their study were immobilised in a stereotactic body frame. The mean 3D tumour motion in both studies was comparable (7.0 mm in our study versus 6.7 mm in their subgroup with the largest motion). The difference between the results can be explained partly by their use of translational corrections only, but also by different volumes used for soft tissue matching. The CBCTs in the study of Purdie et al. [Citation10] were matched upon an internal target volume (ITV) that was the sum of two extreme positions of the tumour (i.e. end-inspiration and end-expiration), while the tumour visualisation on the CBCT is an average over several respiratory cycles. Since most tumours spend more time in the expiration than in the inspiration phase [Citation7], their presentation on CBCT is closer to the end-expiration phase. The tumour presentation on the midventilation phase (which is time weighted average tumour position) is therefore also closer to the end-expiration phase.
To our knowledge, our study is the first study investigating rotational variability in patient and tumour position, and baseline shift in SBRT. The extent of rotational errors was evaluated in order to justify a possible use of the robotic couch for further improvement of positioning uncertainties. Rotational errors exceeded the typically applied tolerance of 3° [Citation16] in less than 10% of all treatment fractions. By using soft tissue tumour matching and correcting for rotational deviations with translational corrections alone, small systematic and random translational errors of around 1 mm (1 SD) remained in all directions.
Omitting the correction of the rotational deviations resulted in systematic rotational errors smaller than 1.5° (1 SD) and slightly smaller random rotational errors. Correction of relatively small rotational errors of a few degrees with the robotic couch would, if the correction was around pitch axis, introduce couch motion of several centimetres at the foot of the treatment couch, due to the fact that the mechanical centre of couch rotation is placed there. This relatively large couch motion may be counteracted by the patient. Patients’ secondary motion, as a reaction to the couch movement, was investigated in prostate patients [Citation17,Citation18]. Linthout et al. [Citation17] investigated tolerance limits for correction of secondary motion and recommended a tolerance of 3 mm and 2°. Scherman et al. [Citation18] showed that 75% of translational secondary motion was < 1 mm, i.e. smaller than the systematic error in SBRT when rotational corrections are omitted. Around 95% of secondary rotations in [Citation18] were within 1.5°, which corresponds to the systematic error when using 3D soft tissue match instead of 6D. However, there may be differences between the patients’ secondary motion as a reaction to the couch movement between the prostate patients and the SBRT patients due to different immobilisation used and general differences in performance status between the two patient groups.
Estimating rotational errors of relatively small and round volumes, such as GTV in SBRT, represents an uncertainty in itself. For a perfect sphere, the same position in space can be achieved by translation only and for a combination of translation and rotation. In cases of non-isotropic target dimensions, concave shapes and/or non-smooth surface irregularities, the correct position in space can not be achieved by translations only and rotational corrections can be necessary. Majority of SBRT targets in this study were not spherical, however this might at least partly be a result of motion induced image artefacts on both CBCT and 4DCT [Citation11].
Based on our clinical experience, automatic matching of very small target volumes often required additional manual corrections of 2–3 mm 3D. Small changes in the size of matching VOI may affect the automatic soft tissue tumour match by up to 1–2 mm and 1°–2°, which was in the range of the observed errors (as presented in ). Furthermore, correction of positional rotational errors of the target by rotating it around the isocentre may also introduce relatively large displacement of an organ at risk (such as spinal cord) into the higher dose region. Therefore, we believe implementation of rotational corrections on the robotic couch would introduce limited improvement in the precision of SBRT treatment delivery. However, the question of clinical importance when aiming for margin reduction and delivering intensity modulated treatments, such as volumetric modulated arc therapy, still needs to be addressed.
Volumetric CBCT imaging before and after the treatment fractions enabled assessment of intra-fractional changes in tumour position; the knowledge of extent of intra-fractional uncertainties is of great importance for PTV margin prescription and hence adequate target coverage. The intra-fractional systematic errors in target position evaluated in our study were similar to other studies [Citation16,Citation19], despite the use of different patient immobilisation devices in the three studies. The differences between the studies were in sub-millimetre range and do not appear clinically significant. The intra-fractional baseline shift we observed was slightly larger than in the findings from Worm et al. [Citation14], probably because patients in their study were immobilised more rigidly in a stereotactic body frame with the use of abdominal compression.
While the intra-fractional baseline shift was largest in AP direction, the systematic inter-fractional error that bone matching would introduce was twice as large in the CC direction as in the AP and LR directions, indicating that, intra-fractionally, tumour position shifts relative to the bones primarily in CC direction. Li et al. [Citation16] showed that in patients with poorer performance status the target tends to drift especially in CC direction, regardless of immobilisation device used. However, they did not evaluate whether this target drift was due to baseline shift or intra-fractional change in the patient position.
Guckenberger et al. [Citation20] investigated intra-fractional changes and found a weak correlation between changes in patient position and target position, indicating that compensation of patient motion during treatment will not eliminate the changes in the target's position, which are, as shown in our study as well, affected to a larger extent by the baseline shifts.
A reduction of baseline shift may occur if the patients’ respiratory pattern was more regular. A study from our institution [Citation21] showed that significant inter-fraction variation in respiration amplitude is present regardless the use of respiratory coaching. Therefore we do not expect that coaching of SBRT patients for the 4DCT and treatment delivery would reduce the baseline shift. Another factor influencing the tumour baseline shift may be immediate physiological or structural changes in the surrounding normal tissue as a reaction to the high radiation dose delivered [Citation22].
The average treatment fraction duration was shorter in our study compared to other studies [Citation10,Citation14,Citation18] where treatments were delivered with more time consuming non-coplanar beam configurations. We found no correlation between treatment delivery duration and target displacement, patient displacement or baseline-shift. This is in agreement with the results from [Citation14,Citation16] where no statistical difference between the 3D position of the target and the duration of a single treatment fraction was found, regardless of patient immobilisation devices used. Results from Purdie et al. [Citation10] showed significant differences in intra-fractional target position when treatment time exceeded 34 min; however, none of the treatment fractions in our study lasted more than 30 min.
Ikushima et al. [Citation22] found, what they define as clinically but not statistically significant inter-fractional trending effects in GTV position relative to the bones: in 20% of the patients the baseline shift was greater than 5 mm. In our study, all baseline shifts were below 5 mm, even though the immobilisation was less rigid. In a study comparing SBRT patients immobilised in either the stereotactic body frame or alpha-cradle, it was shown that adequate image guidance reduces positioning errors to a larger extent than immobilisation [Citation19].
Intra-fractional uncertainties were also evaluated in two recent studies, involving 4D-CBCT image guidance [Citation15,Citation23], where time-weighted tumour mean position from CBCT was used as image guidance. The intra-fractional systematic error in baseline for frameless SBRT [Citation15] was in excellent agreement with our results, despite the additional information yielded by 4D-CBCT compared to standard 3D-CBCT used in our study. The study from Gottlieb et al. [Citation23] demonstrated smaller intra-fractional variability, that can be explained by the higher tumour stage in their study (i.e. loco-regional disease) and hence possible smaller mobility of the lesions investigated [Citation7].
The evaluation of the intra-fractional uncertainties from the pre- and post-treatment CBCTs (in our study as well as in [Citation10,Citation14–16,Citation19,Citation22,Citation23] among others) has a potential drawback: the data does not yield any information on the respiratory movement of the tumour during the beam-on, but refers to an average position of the tumour before and after the daily fraction, while greater movement of the tumour might have occurred during the treatment fraction.
The demonstrated intra-fractional target position changes add further to the uncertainties that need to be accounted for in margin definition, therefore caution needs to be taken prior to implementing a reduction to the applied CTV-PTV margins, even though both systematic and random errors are reduced compared to the bony anatomy based image guidance. In SBRT, as opposed to normo-fractionated radiotherapy, often no GTV to CTV margin is applied, simply assuming GTV = CTV. The correctness of this approach was questioned in a clinicopathological study [Citation24], where the extent of microscopic disease beyond the GTV was found to be up to 9 mm, depending on tumour grade: the microscopic disease was more extensive for lower grade lesions, which may be typical SBRT lesions. However, the required dose for the microscopic disease is unknown and may well be lower than for the GTV [Citation25].
In conclusion, soft tissue tumour match improved precision of treatment delivery, making CBCT essential for target localisation in frameless SBRT of lung tumours. A step from translational corrections (3D) only towards the inclusion of the rotational corrections (6D) as well may further improve the precision of SBRT delivery. Despite a relatively low degree of patient immobilisation, the changes in target position were primarily affected by translational and rotational changes in tumour baseline position relatively to the bony anatomy.
Declaration of interest: The authors report no conflicts of interest. The authors alone are responsible for the content and writing of the paper.
References
- Bauman P, Nyman J, Lax I, Friesland S, Høyer M, Ericsson SR, . Factors important for efficacy of stereotactic body radiotherapy of medically inoperable stage I lung cancer. A retrospective analysis of patients treated in the Nordic countries. Acta Oncol 2006;45:787–95.
- Bauman P, Nyman J, Høyer M, Wenneberg B, Gagliardi G, Lax I, . Outcome in a prospective phase II trial of medically inoperable stage I non-small-cell lung cancer patients treated with stereotactic body radiotherapy. J Clin Oncol 2009;27:3290–6.
- Timmerman R, Paulus E, Galvin J, Michalski J, Straube W, Bradley J, . Stereotactic body radiation therapy for inoperable early stage lung cancer. JAMA 2010;303:1070–6.
- Benedict SH, Yenice KM, Followill D, Galvin JM, Hinson W, Kavanagh B, . Stereotactic body radiation therapy: The report of AAPM Task Group 101. Med Phys 2010;37:4078–101.
- Taylor ML, Kron T, Franich RD. A contemporary review of stereotactic radiotherapy: Inherent dosimetric complexities and the potential for detrimet. Acta Oncol 2011;50:483–508.
- Korreman S, Mostafavi H, Le QT, Boyer A. Comparison of respiratory surrogates for gated lung radiotherapy without internal fiducials. Acta Oncol 2006;45:935–42.
- Seppenwoolde Y, Shirato H, Kitamura K, Shimizu S, van Herk M, Lebesque J, . Precise and real-time measurement of 3D tumour motion in lung due to breathing and heart beat, measured during radiotherapy. Int J Radiat Oncol Biol Phys 2002;53:822–34.
- Juhler-Nottrup T, Korreman SS, Pedersen AN, Persson GF, Aarup LR, Nystrom H, . Interfractional changes in tumour volume and position during entire radiotherapy courses for lung cancer with respiratory gating and image guidance. Acta Oncol 2008;47:1406–13.
- Sonke J-J, Lebesque J, van Herk M. Variability of four-dimensional computed tomography patient models. Int J Radiat Oncol Biol Phys 2008;70:590–8.
- Purdie TG, Bisonnette J-P, Franks K, Bezjak A, Payne D, Sie F, . Cone-beam computed tomography for on-line image guidance of lung stereotactic radiotherapy: Localisation, verification, and intrafraction tumor position. Int J Radiat Oncol Biol Phys 2007;68:243–52.
- Persson GF, Nygaard DE, Munck af Rosenschold P, Vogelius IR, Josipovic M, Specht L, . Artifacts in conventional computed tomography (CT) and free breathing four-dimensional CT induce uncertainty in gross tumor volume determination. Int J Radiat Oncol Biol Phys (in press) doi:10.1016/j.ijrobp.2010.10.036
- Wolthaus JWH, Schneider C, Sonke J-J, van Herk M, Belderbos JSA, Rossi MMG, . Mid-ventilation CT scan construction from four-dimensional respiration-correlated CT scans for radiotherapy planning of lung cancer patients. Int J Radiat Oncol Biol Phys 2006;65:1560–71.
- van Herk M. Errors and margins in radiotherapy. Semin Radiat Oncol 2004;14:52–64.
- Worm ES, Hansen AT, Petersen JB, Muren LP, Prestegaard LH, Høyer M. Inter- and intrafractional localisation errors in cone-beam CT guided stereotactic radiation therapy of tumours in the liver and lung. Acta Oncol 2010;49:1177–83.
- Sonke J-J, Rossi M, Wolthaus J, van Herk M, Damen E, Belderbos J. Frameless stereotactic body radiotherapy for lung cancer using four-dimensional cone beam CT guidance. Int J Radiat Oncol Biol Phys 2009;74:567–74.
- Li W, Purdie TG, Taremi M, Fung S, Brade A, Cho BCJ, . Effect of immobilization and performance status on intrafraction motion for stereotactic lung radiotherapy: Analysis of 133 patients. Int J Radiat Oncol Biol Phys (in press) doi:10.1016/j.ijrobp.2010.09.035
- Linthout N, Verellen D, Tournel K, Reynders T, Duchateau M, Storme G. Assessment of secondary patient motion induced by automatic couch movement during on-line 6 dimensional repositioning in prostate cancer treatment. Radiother Oncol 2007;83:168–74.
- Scherman JB, Seiron K, Logadottir A, Munck af Rosenschöld P, Petersen PM, Bangsgaard JP. Evaluation of intrafractional motion for prostate patients using a 6D couch positioning system. Proceedings of ESTRO (European Society of Therapeutic Radiology and Oncology) Conference on Physics and Radiation Technology for Clinical Radiotherapy. London, 2011.
- Grills IS, Hugo G, Kestin LL, Galerani AP, Chao KK, Wloch J, . Image-guided radiotherapy via daily online conebeam CT substantially reduces margin requirements for stereotactic lung radiotherapy. Int J Radiat Oncol Biol Phys 2008; 70:1045–56.
- Guckenberger M, Meyer J, Wilbert J, Richter A, Baier K, Mueller G, . Intra-fractional uncertainties in cone-beam CT based image-guided radiotherapy (IGRT) of pulmonary tumors. Radiother Oncol 2007;83:57–64.
- Persson GF, Nygaard DE, Olsen M, Juhler-Nøttrup T, Pedersen AN, Specht L, . Can audio coached 4DCT emulate free breathing during the treatment course? Acta Oncol 2008;47:1397–405.
- Ikushima H, Balter P, Komaki R, Hunjun S, Bucci MK, Liao Z, . Daily alignment results of in-room computed tomography-guided stereotactic body radiation therapy for lung cancer. Int J Radiat Oncol Biol Phys 2011;79:473–80.
- Gottlieb KL, Hansen CR, Hansen O, Westberg J, Brink C. Investigation of respiration induced intra- and inter-fractional tumour motion using a standard Cone Beam CT. Acta Oncol 2010;49:1192–8.
- Grills IS, Fitch DL, Goldstein NS, Yan D, Chmielewski GW, Welsh RJ, . Clinicopathologic analysis of microscopic extension in lung adenocarcinoma: Defining clinical target volume for radiotherapy. Int J Radiat Oncol Biol Phys 2007; 69:334–41.
- Sonke J-J, Belderbos J. Adaptive radiotherapy for lung cancer. Semin Radiat Oncol 2010;20:94–106.