Abstract
Background. Fiducials can be used as surrogate for target position during radiotherapy. However, fiducial motion could lead to potential position errors when using fiducials in four-dimensional computed tomography (4DCT) treatment planning and for gated image guided radiotherapy (IGRT). Material and methods. One gold marker (GM) and 5, 10 and 15 mm nickel-titanium (NiTi) stents were inserted in a moving phantom for the purpose of fiducial detection in 4DCT and gated IGRT. Fiducial position errors in 4DCT and BrainLAB's gated IGRT were defined as residuals between fiducial detection and the actual physical position at the instance of image acquisition. Results. Fiducials position errors correlate to speed, fiducial type and orientation during 4DCT acquisition. Lower detection accuracy was measured for the 5 mm NiTi-stent relative to the 10 and 15 mm NiTi stents and GM. Fiducials with orientation 45° relative to the scan direction showed a lower detection accuracy relative to parallel and perpendicular orientations. The standard deviation of position errors in 4DCT were up to 2.2 mm with a maximum deviation of 4.0 mm. Using BrainLAB's gated IGRT the fiducials were detected with a standard deviation of 0.6 mm and a maximum deviation of 1.9 mm. For gated IGRT no correlation to fiducial speed was found. Conclusions. Clinical use of fiducials in combination with treatment planning on mid-ventilation CT phase for moving target should include margins up to 5.5 mm due to potential systematic position errors.
One of the challenges in radiotherapy of thoracic cancer patients is the management of respiratory motion in both planning and treatment. One method for quantifying respiratory motion is four-dimensional computed tomography (4DCT) for treatment planning combined with gated image guided radiotherapy (gated IGRT). However, 4DCT does produce artefacts by irregularly respiration or lack of temporal resolution [Citation1–3]. These uncertainties from the treatment preparation could lead to systematic errors, which calls for additional margin around the clinical target volume (CTV). A recent study [Citation4] showed an increased risk of pneumonitis for inferior tumor positions, probably due to tumor motion and increased margin. IGRT could be one method to minimize the target margin. A variety of technical solutions have been developed for IGRT [Citation5]. Some of the IGRT systems may furthermore be combined with respiratory management. Handling of respiratory motion requires observable and identical target definition on both planning CT and for IGRT. A recent study [Citation6] comparing soft tissue and bone matching for lung tumors showed increased accuracy for soft tissue matching. Other surrogates visible in x-ray images are implanted fiducials such as gold marker seeds or wires (GM) [Citation7,Citation8]. An alternative fiducial could be insertion of intrabronchial nickel-titanium stent (NiTi-stent) recently tested on animals [Citation9,Citation10]. A study by Matney et al. [Citation11] investigated the detection accuracy for a coil fiducial within 4DCT and for BrainLAB's gated IGRT system. However only one coil fiducial aligned parallel to the motion and a single slice thickness was investigated. Margin calculation according to van Herks formula [Citation12] requires standard deviations of the systematic and random errors for clinical use.
The aim of this study is to quantify detection accuracies in terms of standard deviations within 4DCT and gated IGRT for different fiducials designed for thoracic cancer patients.
Material and methods
Fiducials and phantom
A GM sized 5 mm × 1 mm and NiTi-stents with different lengths of 5, 10 and 15 mm were used. The NiTi-stents had a diameter of 3.5 mm and a 6.0 mm expanded collar (). Three identical fiducials were positioned in a solid plastic phantom for each experiment, separated laterally and longitudinally with a minimum distance of 40 mm. A standard BrainLAB ET Gating Phantom Ver1.0.0, manufactured by BrainLAB AG Feldkirchen Germany was used to simulate respiratory motion. It consists of two moving platforms, where one simulates an internal respiratory motion in the superior-inferior (S-I) direction, and the other simulates the external respiration (thoracic wall motion) in the anterior-posterior (AP) direction. The AP motion was used as a surrogate for the internal respiration during the 4DCT scans and the treatment simulation. For this study, synchronized motions between internal and external platforms were necessary as the external motion was used to define the physical phantom position during both 4DCT and gated IGRT. Consequently the two platforms were connected by a wire and driven by a single step motor. With synchronic platform motion, calibration of the phantom S-I motion amplitude was performed using the BrainLAB Infra-Red (IR) camera. Additionally, a reference AP motion curve was recorded as a mean motion path (SD = 0.1 mm, max deviation of 0.2 mm) within 2 min. The fiducial physical positions were defined relative to the AP reference curve. A nearly sine motion path with 20.0 mm amplitude and cycle time of 5.0 s was used. A motion closely related to a sine profile was selected to distribute phantom velocities almost equally between minimum and maximum values during the phase bin acquisitions.
Scan protocol
4DCT with 10 phase bins were acquired using a General Electric Medical Systems LightSpeed RT16 CT scanner with a GE workstation V4.2 and Varian RPM. 3D volumes were named according to the phase bin with CT phase bin 0% corresponding to maximum inhale and CT phase bin 50% to full exhale. The scanner was operated in cine axial mode at 120 kV and 80 mAs with a CT gantry rotation time and cine time between images of 0.5 s. Fiducials were scanned with slice thicknesses of 1.25 mm and 2.5 mm both with CT scanner collimation at 10 mm and axial resolution of 0.977 mm/pixels. While the phantom motion was only 1D with S-I displacement, the potentially fiducial orientation dependency was investigated. The NiTi-stents were scanned with orientations of 0°, 45° and 90° relative to the motion. The GMs were scanned with orientations 0° and 90° only.
Fiducials in each 4DCT scans were automatically detected using an in-house MatLab software script. All fiducials were segmented with a region growing method using the maximum pixel value within each slice as a seed point. The surrounding 26 voxels of the seed point were accepted by the segmentation algorithm according to the following two criteria: 1) A CT value above 1300 HU; and 2) A maximum distance threshold of 5 mm where clusters were accepted to merge a segmented volume. A CT value of 1300 HU was selected for two reasons. First, no phantom component contains CT values above 1300 HU except the electrical and mechanical parts. Second, all fiducials have CT value above 3072 HU (CT saturation value) in the stationary CT phases. Due to motion blur the CT values of the fiducials decrease in the non-stationary phases. Nevertheless fiducials were still detectable at 1300 HU. Ideally, this segmentation would provide three clusters, one for each of the three fiducials in the phantom. However, motion artefacts lead to fragments of segmented clusters. These fragments were handled by the second criteria, by which fragments were merging a segmented volume if the length of the 3D vectors between fragments were within the threshold of 5 mm. The non-fiducial parts (electric and mechanical) were excluded using a region of interest defined as the phantom surface.
Respiratory gated image guidance
Gated IGRT simulations were performed using a Varian Clinac iX equipped with BrainLAB ExacTrac Robotics V.5.52 and beam gating. The x-ray settings for image acquisitions were 100 kV, 50 mA and 100 mS. The acquired x-ray images were obtained at predefined amplitude levels according to and during the exhale period only. The amplitude levels in were selected to cover the phantom speed range during the time period from full inhale to end exhale. The fiducials were defined in the ExacTrac software using endpoint description for fiducials of type 10 mm and 15 mm NiTi-stents. The GM and the 5 mm NiTi-stent were defined using a single point as these fiducials were displayed on a few number of CT slices. The BrainLAB gating module operates with relative amplitude values (0–100%) as described in . Initially, the phantom was positioned with an amplitude level of 0% identical to CT phase bin 50%. With motion applied to the phantom, the fiducials were moving with a displacement of 20 mm relative to the initial setup. Accuracies of the gated IGRT were calculated as residuals between the fiducial positions detected on gated verification images and the expected physical displacement from the initial setup.
Table I. Correlation between phantom speed values [mm/s], amplitude levels [%] for gated IGRT and phantom physical displacement [mm] relative to the initial setup with amplitude of 0 mm.
Results
4DCT
Three segmented volumes were achieved within all 4DCT scans using the region growing method. A total of 66 fiducial volumes were segmented for each phase bin, using the combination of three fiducials in each scan, four different fiducials, two slice thicknesses and three orientations (two orientations for GM). All the fiducial center-of-masses detected in retrospectively sorted CT phases were plotted in . No deviation occurs at phase bin 50% as this phase was used as reference for the entire positioning measurements. Segmented position errors were within 0.2 mm in the AP and left-right directions. The maximum fiducial deviation from the reference position was 4.0 mm. A summary of fiducial detected position errors in 4DCT is listed in . F-tests for equal variances were used to separate position errors between fiducial types, orientations and slice thicknesses. No differences in position errors between the CT slice thicknesses of 1.25 mm and 2.50 mm were observed (p = 0.85). The standard deviations of position errors were correlated to the phantom speed at each phase bin center. In the standard deviations are plotted for each fiducial type and in according to fiducial orientations. Linear regression on the former data confirmed a significant different slope for the 5 mm NiTi-stent (p = 0.003) compared to the GM, 10 and 15 mm NiTi-stent which have identical parameters. The linear regression for fiducial orientations (all fiducials included) confirmed a significant different slope for fiducial aligned at 45° relative to phantom motion (p = 0.006).
Figure 2. Fiducials center-of-masses within the 4DCT normalized to phase bin 50%. The figure represents all fiducials, slice thicknesses and orientations. The solid line indicates the actual physical position of the fiducials within a full cycle.
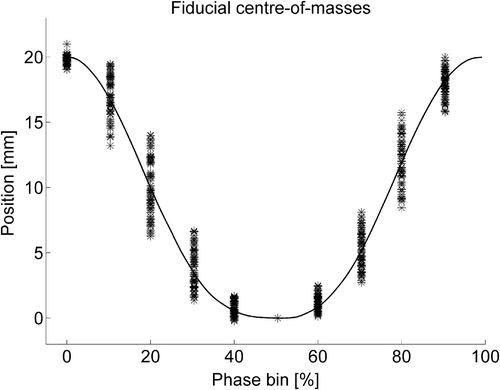
Figure 3. 4DCT standard deviations of the fiducial position errors relative to phantom speed at center of phase bin acquisition. All three orientations and both slice thicknesses were included with separation into groups of fiducial types. Linear regression for the 5 mm NiTi stent were significant different from the other three fiducials ().
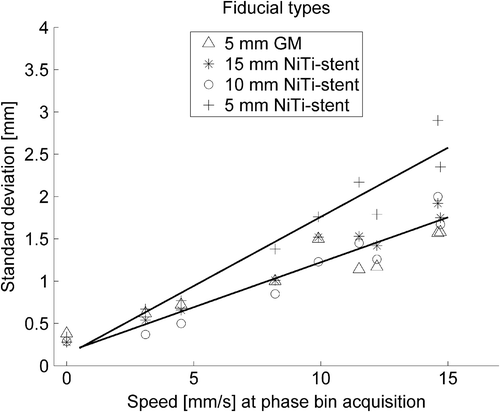
Figure 4. 4DCT standard deviations of the fiducial position errors relative to phantom speed at center of phase bin acquisition. All fiducial types were included with both slice thicknesses with separation into fiducial orientations. Linear regression for fiducial orientated at 45° were significant different from the other two orientations ().
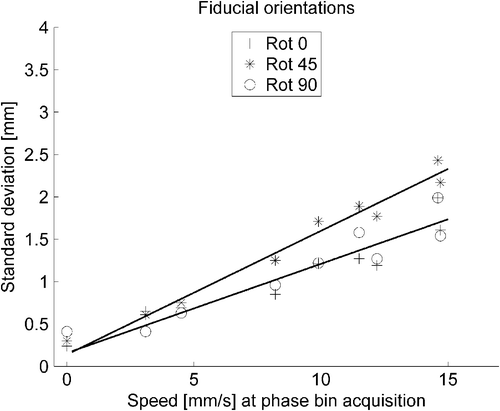
Table II. 4DCT fiducial detection divided into groups of fiducial types, orientations and CT scan slice thicknesses. The fiducial position errors were listed with mean residuals over all CT phases and both slice thicknesses and the 95% CI of the mean and standard deviation (SD) of the group distributions. The mean of fiducial types were for all orientations and both slice thicknesses. Similarly the mean of fiducial orientations were for all types and both slice thicknesses. The mean of slice thicknesses were for all fiducials and orientations. Regression parameters for the standard deviations were listed with 95% CI for fiducial types and orientations with both slice thicknesses included.
The interception points of the linear regression for fiducial types and orientations, respectively, showed no significant variation, corresponding to identical position errors on CT phases acquired at low speed. The standard deviation of fiducial position errors were in the range of 0.2 mm to 2.2 mm, using regression parameters for fiducials with 45° orientation only.
BrainLAB's gated IGRT
A total of 44 fiducial detections were performed at each phantom speed level in . Two sets of verification images were acquired for each of the four fiducial types, two slice thicknesses and three orientations (GM two orientations). The standard deviations for verification images showed no significant differences across phantom speed values. The maximum fiducial detection from the expected position was 1.9 mm. For the gated IGRT no correlation was found to fiducial type, orientation and slice thickness. In this study fiducial position with BrainLAB's ExacTrac gated image guidance was verified with a mean deviation from the physical position of 0.15 mm CI (0.06; 0.24). The standard deviation of the total distribution of position errors was 0.63 mm. Using one-sample T-test points to an offset of the mean value from zero (p = 0.0008).
Discussion
The distributions of the segmented fiducials agreed with the actual physical positions within 4DCT, as the mean and 95% CI in were close to zero. However, by separating the position errors into different speed regimes during the 4DCT some differences could be observed. The lower accuracy of the 5 mm NiTi-stent position is likely to be a combination of a small size and less dense material (NiTi). This could affect the segmented volume and thus the center-of-mass in CT phases acquired at high speed. Similarly, less accuracy for fiducials with 45° orientation was observed. An exact alignment of fiducials during implantation parallel or perpendicular to the motion is not feasible in a clinical situation. Therefore, the safety margin for fiducials should be considered from position errors on fiducials aligned at 45°, which in this study ranged from 0.2 mm to 2.2 mm. Applying a systematic deviation of 2.2 mm into Van Herks formula [Citation12] for margin calculation, results in a CTV margin of 5.5 mm due to position uncertainty. The maximum speed value in this study was closely related to the time weighted mid-ventilation phase. The mid-ventilation phase is frequently used for dose calculation in radiotherapy [Citation13–15]. Thus, calculated CTV margin in this study may be clinically relevant for a target in the inferior thorax where 20 mm motion [Citation1] has been observed. Alternatively using the 4DCT exhale phase for dose calculation, the CTV margin due to fiducial position uncertainty can be reduced to 0.5 mm, because of the minimal motion during phase bin acquisition. Even though GMs were only segmented on one or two slices with orientation at 90°, the slice thicknesses showed not to influence the fiducial precision. Using the center-of-mass instead of full length as fiducial detection may have blurred the distribution within equal variances.
The position accuracy of the BrainLAB gated IGRT system shows no correlation to motion speed during image acquisition. The lack of correlation to phantom speed may be related to the rapid x-ray verification time of 100 ms. During this time the phantom moves up to 1.5 mm which is not far from the measured 95% prediction interval of 1.2 mm (two standard deviations for position errors). BrainLAB's gating system uses IR markers on the phantom to predict the respiratory motion, combined with two X-ray images which are not acquired synchronically in one respiration cycle. The precision of this system is consequently linked to its ability to acquire two sequential X-ray images at reproducible amplitudes during respiration. This, however, does not constitute a problem in the current study, as the respiratory motion is close to sine shaped and identical within repeated cycles. Irregular patient respirations would probably influence the tracking precision which was not investigated in this study. Our study showed a systematic position error of 0.15 mm (mean deviation from expected position) for gated IGRT. This indicates that x-ray images were acquired slightly before time. Results in this study are in accordance with Matney et al. [Citation11] who used a coil fiducial and reported a maximum position deviation up to 3.5 mm in 4DCT and less than a millimenter using BrainLAB gated IGRT. Matney et al. also found the largest position deviations occurred in CT phases with the highest velocities. In contrast to the present study, Matney et al. only measured for a coil fiducial aligned parallel to phantom motion and thus not correcting for the effects of a fiducial rotation. The group only reported maximum deviations and did not provide an error estimate for the clinical situation. The maximum deviation of a single detected fiducial center-of-mass in our study was 4.0 mm on 4DCT and 1.9 mm for the gated IGRT.
In conclusion accuracy of fiducial position in 4DCT demonstrated correlation to fiducial orientation, material density/size and speed during the CT phase selected for treatment planning. These effects may have to be included in the CTV to planning target volume margin in case of radiotherapy for respiratory moving targets.
Acknowledgements
The authors acknowledge The Lundbeck Foundation Center for Interventional Research in Radiation Oncology (CIRRO), The Danish Graduate School in Clinical Oncology (DAFKO) and PNN Medical. For technical assistance the authors acknowledge Frank Meyer-Lassen.
Declaration of interest: The authors report no conflicts of interest. The authors alone are responsible for the content and writing of the paper.
References
- Keall PJ, Mageras GS, Balter JM, Emery RS, Forster KM, Jiang SB, et al. The management of respiratory motion in radiation oncology report of AAPM Task Group 76. Med Phys 2006;33:3874–900.
- Persson GF, Nygaard DE, Brink C, Jahn JW, Munck Af RP, Specht L, et al. Deviations in delineated GTV caused by artefacts in 4DCT. Radiother Oncol 2010;96:61–6.
- Persson GF, Nygaard DE, Af Rosenschold PM, Richter V, I, Josipovic M, Specht L, et al. Artifacts in conventional computed tomography (CT) and free breathing four-dimensional CT induce uncertainty in gross tumor volume determination. Int J Radiat Oncol Biol Phys 2011;80:1573–80.
- Vogelius IR, Bentzen SM. A literature-based meta-analysis of clinical risk factors for development of radiation induced pneumonitis. Acta Oncol 2012;51:975–83.
- Verellen D, De RM, Storme G. A (short) history of image-guided radiotherapy. Radiother Oncol 2008;86:4–13.
- Josipovic M, Persson GF, Logadottir A, Smulders B, Westmann G, Bangsgaard JP. Translational and rotational intra- and inter-fractional errors in patient and target position during a short course of frameless stereotactic body radiotherapy. Acta Oncol 2012;51:610–7.
- Bhagat N, Fidelman N, Durack JC, Collins J, Gordon RL, LaBerge JM, et al. Complications associated with the percutaneous insertion of fiducial markers in the thorax. Cardiovasc Intervent Radiol 2010;33:1186–91.
- Yousefi S, Collins BT, Reichner CA, Anderson ED, Jamis-Dow C, Gagnon G, et al. Complications of thoracic computed tomography-guided fiducial placement for the purpose of stereotactic body radiation therapy. Clin Lung Cancer 2007;8:252–6.
- Carl J, Nielsen J, Nielsen MS, Zepernick PR, Kjaergaard B, Jensen HK. A new lung stent tested as fiducial marker in a porcine model. Radiother Oncol 2012;102:297–302.
- Carl J, Jensen HK, Nielsen J, Nielsen MS, Schmid M, Siegfried L. A new fiducial marker for gated radiotherapy in the lung – a feasibility study of bronchoscopy based insertion and removal in Göttingen mini-pig. Scand J Lab Animal Sci 2010;37:117–27.
- Matney JE, Parker BC, Neck DW, Henkelmann G, Rosen II. Target localization accuracy in a respiratory phantom using BrainLAB ExacTrac and 4DCT imaging. J Appl Clin Med Phys 2011;12:3296.
- van Herk M, Remeijer P, Rasch C, Lebesque JV. The probability of correct target dosage: Dose-population histograms for deriving treatment margins in radiotherapy. Int J Radiat Oncol Biol Phys 2000;47:1121–35.
- Wolthaus JW, Schneider C, Sonke JJ, van HM, Belderbos JS, Rossi MM, et al. Mid-ventilation CT scan construction from four-dimensional respiration-correlated CT scans for radiotherapy planning of lung cancer patients. Int J Radiat Oncol Biol Phys 2006;65:1560–71.
- Korreman S, Persson G, Nygaard D, Brink C, Juhler- Nottrup T. Respiration-correlated image guidance is the most important radiotherapy motion management strategy for most lung cancer patients. Int J Radiat Oncol Biol Phys 2012;83:1338–43.
- Li G, Citrin D, Camphausen K, Mueller B, Burman C, Mychalczak B, et al. Advances in 4D medical imaging and 4D radiation therapy. Technol Cancer Res Treat 2008;7:67–81.