To the Editor,
Chordomas are rare, slow-growing tumors that arise from the remnant of the primitive notochord. They are characterized by a locally aggressive growth pattern and high recurrence rates. The most common site is the sacrococcygeal area (50%), followed by the skull base (35%). The remaining 15% of cases occur in the midline along the path of the notochord, and primarily involve the cervical vertebrae [Citation1]. The management of chordomas is challenging, because they lie in the vicinity of critical structures, such as the optic pathway/brainstem/spinal cord for skull base and cervical vertebral tumors, and the small intestine/rectum/spinal nerves for sacrococcygeal tumors. These anatomic structures often limit surgical access and resectability, as well as the delivery of high radiation doses. A dose-response relationship has been reported for chordomas: 80% of tumors were controlled in patients treated with > 80 cobalt gray equivalents (CGE = proton dose in Gy × 1.1), whereas only 20% were controlled in patients treated with 40–60 CGE [Citation2]. Proton therapy has been used to precisely deliver radiation to the target, sparing the normal critical structures [Citation3–5]. The optimal dose/fractionation is, however, not well defined. In this study, we prospectively evaluated volumetric tumor response following hypofractionated proton therapy (PT) and tried to determine the optimal dose to achieve local control with a literature review of patients with chordomas.
Material and methods
Patient eligibility
Eligibility criteria were as follows: biopsy-proven chordomas; postoperative gross residual or recurrent tumor in imaging studies; no previous irradiation for the tumor in the same location; no distant metastasis; and an Eastern Cooperative Oncology Group performance status (ECOG-PS) of 0–2. All patients signed informed consent forms before entering the study. Our institutional review board approved the study protocol.
Proton therapy
The gross tumor volume (GTV) was defined as the gross volume of all tumors detected, based on clinical and imaging studies, including computed tomography (CT), magnetic resonance imaging (MRI). The planning target volume (PTV) was expanded from the GTV with 2–10 mm margins, considering the organs at risk (OAR) and the set-up uncertainty depending on the locations of primary sites. In postoperative cases, it was optional with delineation of clinical target volume (CTV) to include high-risk areas of subclinical disease, such as the surgical entry region for the trans-sphenoidal approach and the surgical tumor bed, with 2–5 mm margins. With heterogeneity correction, the dose was prescribed to the 100% iso-dose line, which covers at least 95% of the volume of the PTV. Patients were treated daily, five times per week, with one of the following doses: 64.8 CGE/27 fractions, 69.6 CGE/29 fractions, 74.4 CGE/31 fractions, and 79.2 CGE/33 fractions. The corresponding doses for PTV2 were 54 CGE/27 fractions, 55.1 CGE/29 fractions, 55.8 CGE/31 fractions, and 56.1 CGE/33 fractions. Dose constraints for OAR were as follows: the median dose was not allowed to exceed 54 CGE for the brain, brain stem, optic pathway, cochlea, and recto-sigmoid colon, and 45 CGE for the spinal cord and small intestine. Moreover, 1% of the volume was not allowed to exceed 60 CGE for the brain, brain stem, and cochlea; 58 CGE for the optic pathway; 54 CGE for the spinal cord and small intestine; and 70 CGE for the recto-sigmoid colon. The dose level that delivered the highest possible dose to the PTV such that the doses to the adjacent OARs were acceptable was chosen by evaluating the dose-volume histogram. Treatment planning was performed using bilateral beams with the Eclipse proton beam planning system (Ver. 8.1; Varian Medical System, Palo Alto, CA, USA). All patients were treated with proton of passive double scattering mode (Proteus 235; Ion Beam Application, S.A., Louvain-la-Neuve, Belgium). Equivalent uniform dose (EUD) was calculated using the program suggested by Gay and Niemierko [Citation6].
Measurement methods, radiographic response and toxicity criteria
For volumetric measurement, volumetric reconstruction was achieved using our institutional Picture Archiving and Communication System software (Infinitt Co. Ltd., Seoul, Korea) by multiplying the sum of all areas measured on axial images by the section thickness. Response criteria were as follows: complete response (CR), complete resolution; partial response (PR), a decrease of at least 50%; minimal response (MR), a decrease of at least 25% but less than 50%; progressive disease (PD), an increase of more than 25%; and stable disease (SD), neither a MR nor PD. Acute toxicity was assessed according to the Common Terminology Criteria (CTCAE v.3.0), late toxicity was classified according to the Radiation Therapy Oncology Group (RTOG) / European Organization for Research and Treatment of Cancer (EORTC) score.
Statistical analysis
Survival rates were calculated from the date of the start of treatment to the date of either an event occurring or the end of follow-up using the Kaplan-Meier method. Univariate log-rank tests were used to assess variables affecting survival. The SPSS statistical software (version 18.0; SPSS, Chicago, IL, USA) was used for statistical analyses. Statistical significance was set at p < 0.05.
Data collection and follow-up
Follow-up involved visits to the clinic, correspondence with the referring physician, or direct telephone contact with the patient. Serial imaging (MRI) studies were requested every six months for two years and annually thereafter. Acute toxicities were defined as adverse events that occurred from the first day of treatment through day 90 after treatment. All side effects seen after 90 days from the end of PT were considered late adverse events.
Results
From May 2007 to May 2011, 20 patients were enrolled. Primary sites were located in the skull base (eight patients), cervical spine (four patients), and sacrum (eight patients). Seven, nine, three and one patients received doses 1 (64.8 CGE), 2 (69.6 CGE), 3 (74.4 CGE), and 4 (79.2 CGE), respectively. Median tumor volume was 21.4 cm3 (range 0.1–1014.1 cm3). Details are shown in .
Table I. Patient characteristics.
The median EUD/prescribed dose for GTV was 1.045 (range 0.97–1.10). The corresponding figure for PTV2 was 1.04 (range 1.00–1.08). The median follow-up duration was 43 months (range 9–53 months). Four patients experienced recurrence; all of them had been treated with 64.8 CGE. There were two cases of in-field recurrence: one patient with a tumor in the cervical spine suffered local recurrence 21 months after therapy, while a second patient with a bulky tumor (1014.11 cm3) in the sacrum suffered local recurrence and bone metastasis 12 months after therapy. Craniospinal fluid seeding was revealed at 25 months after therapy in one patient who had a tumor at the skull base and who had undergone tumor removal by the trans-sphenoidal approach prior to proton beam radiotherapy. Multiple bone metastases were detected 25 months after therapy in one patient who had a tumor in the sacrum. The patient who had local recurrence underwent only a salvage operation and is alive with residual tumors after surgery. The other three patients received palliative radiotherapy with photons and are alive with disease.
A total of 76 MRI scans (20 pre-RT and 56 post-RT) were used for the volumetric evaluation (Supplementary Figure A1 available online at http://informahealthcare.com/doi/abs/10.3109/0284186X.2013.833345). The median number of MRI scans per patient was 5.5. Tumor volume ratios (volume measured at follow-up by MRI relative to the pre-PT value) were plotted on a scattergram and a line of best fit was obtained (). The line of best fit was intended to show the initial tumor response and the speed of tumor shrinkage before progression. Therefore, the plot was created after points representing progression after an initial response were discarded. Time to a 50% or greater reduction in volume (T50) was 26 months.
Figure 1. Relative volumes measured using follow-up MRI scans with reference to pre-radiotherapy values were plotted on a scattergram and a line of best fit was obtained. Time to a 50% or greater reduction in volume (T50) was 26 months.
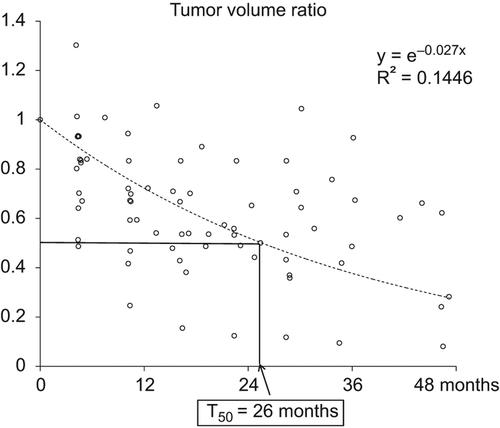
All of the tumors treated with a dose of 74.4 CGE or more showed objective responses (a volume reduction of at least 25%), compared with only 69% of the tumors treated with a dose of 69.6 CGE (11/16). Representative cases of successful serial volume reduction are shown in .
Figure 2. Serial sagittal and axial T2-weighted images (short T1 inversion recovery image at 2-year follow-up (FU) sagittal image) of a sacral chordoma in a 62-year-old male. The patient received 74.4 CGE and his tumor shrank gradually. He achieved a near complete response at 3-year FU.
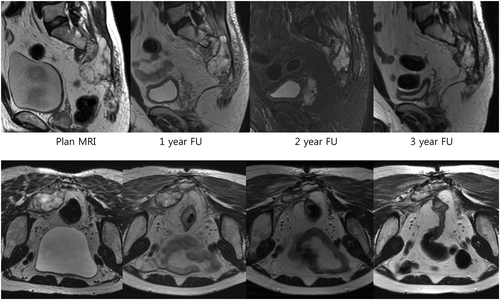
There was no grade 3 acute toxicity. One patient with a sacral chordoma treated with 69.6 CGE experienced grade 3 rectal bleeding at 14 months after therapy. Telangiectasia and active bleeding were observed 10 cm above the anal verge by sigmoidoscopy and were treated by Argon laser cauterization. The same patient also developed grade 3 skin and subcutaneous tissue contracture at nine months after therapy. These adverse events persisted until the end of follow-up (46 months). Another patient, who had a sacral chordoma and was treated with 64.8 CGE, also suffered from persistent grade 3 skin and subcutaneous contracture beginning 10 months after therapy.
Discussion
In the past, conventional X-ray therapy with relatively low dose radiation resulted in suboptimal LCRs, with no objective response in most cases. However, as shown in this study, chordomas are very radio-responsive if the dose of radiation is sufficient to control the disease. Chordomas shrink as much as other cancers in a dose-dependent manner: in the present study, all tumors treated with a dose of 74.4 CGE or greater showed objective responses, compared with only 69% of tumors treated with a dose of 69.6 CGE or less (11/16). Unlike other common cancers, however, the rate of volume reduction is much slower, as shown by the time to a 50% or greater reduction in volume (T50) of 26 months. In other words, it takes over two years to reduce the volume of the original chordoma by 50%, and so long-term follow-up is needed to evaluate objective responses. To our knowledge, this study is the first to report changes in chordoma volume after PT. One previous study showed volume changes in chordomas after carbon ion radiotherapy [Citation7]. Interestingly, the T50 value in our study was similar to that of carbon ion therapy. The authors of the carbon ion radiotherapy study also found that, at the end of treatment, the tumor showed an increase in volume of more than 10% in 13 of the 23 cases, which showed reductions in tumor volume afterwards, except in one case. They suggested that this phenomenon, pseudo-progression, might be due to tumor necrosis and edema after treatment. We did not routinely perform imaging follow-up at the end of PT, but one patient showed a 30% increase in tumor volume four months after PT. The tumor volume decreased afterwards and the patient showed SD at the 36-month follow-up. This suggested that tumor necrosis and edema can persist for up to few months after radiotherapy. Considering these results, progression should be distinguished from pseudo-progression; two consecutive increments in tumor volume could be reasonable criteria for progression.
To determine the dose-response relationship, we compiled our results with published data from studies using photon, photon plus proton, proton and carbon ion beams [Citation2,Citation4,Citation5,Citation8–18], which provided information concerning dose/fractionation and five-year LCRs ( and Supplementary Figure A2 to A3 available online at http://informahealthcare.com/doi/abs/10.3109/0284186X.2013.833345). A wide range of doses and fractionation schemes were used in these studies. Therefore, we decided to convert the total doses to EQD2, assuming an α/β ratio of 3 Gy. In these studies, the outcome of particle beam therapy is usually superior to that of photon therapy. The five-year LCRs were 23–69% after treatment with photons alone or photons plus protons at doses of 50.0–67.3 CGE EQD2 [Citation8–12]; 59–100% after proton therapy with doses of 68.5–74.0 CGE EQD2 [Citation4,Citation5,Citation17,Citation18]; and 70–100% after carbon ion therapy with doses of 72–104 CGE EQD2 [Citation2,Citation13,Citation14] (Supplementary Table A1 available online at http://informahealthcare.com/doi/abs/10.3109/0284186X.2013.833345). We observed a clear dose-response relationship in chordoma. Schulz-Ertner et al. [Citation2] reported a dose-response relationship in chordomas. In their study, the total doses in various fractionation schemes were used to draw a dose-response curve, which made determination of the recommended dose difficult because there will be significant differences in biological effects according to fractionation scheme. In our study, we collected more data and converted the total doses to EQD2 to facilitate determination of the appropriate dose.
Figure 3. Compilation of 5-year local control rates for the treatment of chordoma achieved in several reports in the literature. a, photon; b, photon + proton; c, carbon; d, proton.
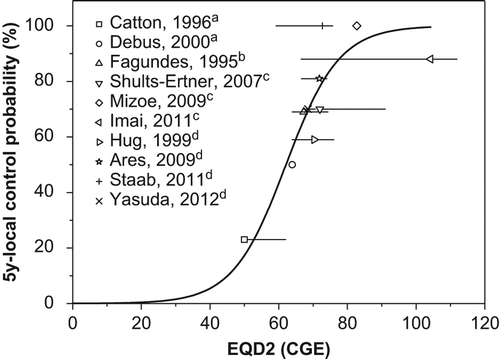
Volumetric modulated arc therapy (VMAT), a recent evolution of photon technique, was introduced and applied to the cases where critical structures were located very close or abutting to the target. VMAT demonstrated benefits in healthy tissue sparing together with good target coverage. However, intensity-modulated proton plans showed significantly higher PTV dose homogeneity than VMAT in the previously reported dosimetric comparison study for soft tissue sarcoma [Citation19]. We were also interested in whether type of particle therapy (proton vs. carbon) has any impact on the outcomes of chordomas. Carbon ion therapy has a few theoretical advantages, such as its higher linear energy transfer and its smaller oxygen enhancement ratio and cell cycle dependency in cytotoxicity compared to proton therapy, although its superiority in terms of the clinical outcomes of patients has not been demonstrated. Five-year LCRs after carbon ion therapy in the literature are 70–100% [Citation2,Citation13,Citation14], which are superior to the five-year LCRs after proton therapy (59–100%) [Citation4,Citation5,Citation17,Citation18]. Its superior outcomes could be attributable to either the potential biological advantages or to the higher total dose. Indeed, the total EQD2 for carbon therapy ranged from 72 to 104 CGE, higher than the 68.5–74 CGE for proton therapy. Although further investigation is warranted to determine the real benefits of carbon therapy, we believe that it is possible to achieve a good objective response with proton therapy if a sufficient dose is delivered, as shown in .
Two patients experienced grade 3 skin and subcutaneous toxicity. One had been treated with 64.8 CGE/27 fractions and the other with 69.9 CGE/29 fractions. This toxicity persisted until the end of follow-up (46 months). These two patients had been treated with a single field because of the technical limitations of oblique beam arrangement for large tumors. There was no serious skin toxicity in the other patients, who had been treated with two or more fields (median 3, range 2–4). Yasuda et al. [Citation18] reported that two of 26 patients treated with PT experienced grade 3 late toxicity. One patient (73.8 CGE) required surgery for necrotic subcutaneous tissue at 18 months after PT; another patient (71 CGE) required surgery for osteonecrosis of irradiated lumbar vertebrae at 26 months after PT. Attention should be given to the skin dose, and a multiple beam arrangement should be used whenever possible.
In conclusion, our data suggest that chordomas are radio-responsive, but that detecting an objective response requires time, with a T50 of 26 months. A weakness of our study is the small number of patients and the short follow-up period. To make up for these weaknesses, we extensively reviewed relevant articles in the literature and compiled them with our data to determine the dose-response relationship. We identified a clear dose-response relationship in chordomas. Based on this study, the EQD2 values for LCRs of 50%, 80%, and 90% at five years were estimated to be 62.1, 72.8, and 79.1 CGE, respectively, although further investigation is warranted to verify the results (Supplementary Figure A4 available online at http://informahealthcare.com/doi/abs/10.3109/0284186X.2013.833345).
Supplementary Figure A1 to A4 and Table A1
Download PDF (602.8 KB)Acknowledgments
This study was supported by Grant No.1010480 from the National Cancer Center, Korea.
Declaration of interest: The authors report no conflicts of interest. The authors alone are responsible for the content and writing of the paper.
References
- Tai PT, Craighead P, Bagdon F. Optimization of radiotherapy for patients with cranial chordoma. A review of dose-response ratios for photon techniques. Cancer 1995;75:749–56.
- Schulz-Ertner D, Karger CP, Feuerhake A, Nikoghosyan A, Combs SE, Jakel O, et al. Effectiveness of carbon ion radiotherapy in the treatment of skull-base chordomas. Int J Radiat Oncol Biol Phys 2007;68:449–57.
- Munzenrider JE, Liebsch NJ. Proton therapy for tumors of the skull base. Strahlenther Onkol 1999;175(Suppl 2):57–63.
- Hug EB, Loredo LN, Slater JD, DeVries A, Grove RI, Schaefer RA, et al. Proton radiation therapy for chordomas and chondrosarcomas of the skull base. J Neurosurg 1999;91:432–9.
- Ares C, Hug EB, Lomax AJ, Bolsi A, Timmermann B, Rutz HP, et al. Effectiveness and safety of spot scanning proton radiation therapy for chordomas and chondrosarcomas of the skull base: First long-term report. Int J Radiat Oncol Biol Phys 2009;75:1111–8.
- Gay HA, Niemierko A. A free program for calculating EUD-based NTCP and TCP in external beam radiotherapy. Phys Med 2007;23:115–25.
- Serizawa I, Imai R, Kamada T, Tsuji H, Kishimoto R, Kandatsu S, et al. Changes in tumor volume of sacral chordoma after carbon ion radiotherapy. J Comput Assist Tomogr 2009;33:795–8.
- Catton C, O’Sullivan B, Bell R, Laperriere N, Cummings B, Fornasier V, et al. Chordoma: Long-term follow-up after radical photon irradiation. Radiother Oncol 1996;41:67–72.
- Debus J, Schulz-Ertner D, Schad L, Essig M, Rhein B, Thillmann CO, et al. Stereotactic fractionated radiotherapy for chordomas and chondrosarcomas of the skull base. Int J Radiat Oncol Biol Phys 2000;47:591–6.
- Zabel-du Bois A, Nikoghosyan A, Schwahofer A, Huber P, Schlegel W, Debus J, et al. Intensity modulated radiotherapy in the management of sacral chordoma in primary versus recurrent disease. Radiother Oncol 2010;97: 408–12.
- Fagundes MA, Hug EB, Liebsch NJ, Daly W, Efird J, Munzenrider JE. Radiation therapy for chordomas of the base of skull and cervical spine: Patterns of failure and outcome after relapse. Int J Radiat Oncol Biol Phys 1995;33:579–84.
- Noel G, Feuvret L, Calugaru V, Dhermain F, Mammar H, Haie-Meder C, et al. Chordomas of the base of the skull and upper cervical spine. One hundred patients irradiated by a 3D conformal technique combining photon and proton beams. Acta Oncol 2005;44:700–8.
- Mizoe JE, Hasegawa A, Takagi R, Bessho H, Onda T, Tsujii H. Carbon ion radiotherapy for skull base chordoma. Skull Base 2009;19:219–24.
- Imai R, Kamada T, Sugahara S, Tsuji H, Tsujii H. Carbon ion radiotherapy for sacral chordoma. Br J Radiol 2011;84: S48–54.
- Weber DC, Rutz HP, Pedroni ES, Bolsi A, Timmermann B, Verwey J, et al. Results of spot-scanning proton radiation therapy for chordoma and chondrosarcoma of the skull base: The Paul Scherrer Institute experience. Int J Radiat Oncol Biol Phys 2005;63:401–9.
- Rutz HP, Weber DC, Sugahara S, Timmermann B, Lomax AJ, Bolsi A, et al. Extracranial chordoma: Outcome in patients treated with function-preserving surgery followed by spot-scanning proton beam irradiation. Int J Radiat Oncol Biol Phys 2007;67:512–20.
- Staab A, Rutz HP, Ares C, Timmermann B, Schneider R, Bolsi A, et al. Spot-scanning-based proton therapy for extracranial chordoma. Int J Radiat Oncol Biol Phys 2011; 81:e489–96.
- Yasuda M, Bresson D, Chibbaro S, Cornelius JF, Polivka M, Feuvret L, et al. Chordomas of the skull base and cervical spine: Clinical outcomes associated with a multimodal surgical resection combined with proton-beam radiation in 40 patients. Neurosurg Rev 2012;35:171–82.
- Fogliata A, Scorsetti M, Navarria P, Catalano M, Clivio A, Cozzi L, et al. Dosimetric comparison between VMAT with different dose calculation algorithms and protons for soft-tissue sarcoma radiotherapy. Acta Oncol 2013;52:545–52.