To the Editor,
Standard three-dimensional (3D) planning studies have shown that dose escalation by use of inhomogeneous dose distribution may increase the local tumour control by more than 10 percentage points for advanced non-small cell lung cancer (NSCLC) patients [Citation1–4]. Nevertheless, only a few clinical studies investigating dose escalation have been initiated [Citation5,Citation6]. There have been concerns regarding the dose distributions with steep gradients within the planned volume. One concern is how the respiratory motion affects the calculated 3D dose to the tumour. Another issue is whether the 3D dose to the organs at risk is representative for actual delivered dose when position uncertainties are included, or whether the steep dose gradients within the tumour might shift towards the organs at risk causing unexpected toxicity. Some studies have investigated the dosimetric effect of geometric uncertainties, e.g. respiratory motion and setup uncertainties [Citation7–9].
Previously, 3D calculations of homogeneous dose distributions and dose-escalated inhomogeneous dose distributions have been studied by Nielsen et al. [Citation1] to evaluate the potential of inhomogeneous dose escalations for NSCLC patients with involved lymph nodes. In the current study, recalculations of the same treatment plans accounting for systematic and random uncertainties (4D calculations [Citation10,Citation11]) were performed in order to validate dose to organs at risk and the reported tumour control probability (TCP) values.
Material and methods
Details of the patient characteristics for the 20 included NSCLC patients have been reported previously [Citation1]. The treatment plans were performed on a mid-ventilation phase from a 4DCT divided in 10 time equidistant phases. Two treatment plans were created for each patient with homogeneous and dose-escalated inhomogeneous dose distributions.
In 3D planning, a planning target volume (PTV) is included to ensure adequate dose coverage of the moving target inside the PTV. Margins were calculated based on the margin equation by van Herk et al. [Citation12]. The idea of that equation was to ensure that 90% of the patients would receive a minimum dose of 95% of the prescribed dose. The dose distribution of the PTV is considered as a surrogate for the 4D dose distribution of the moving target.
In this study, two different PTVs were generated, the standard one around the clinical target volume (CTV) labelled PTVCTV and an additional one related directly to the gross target volume (GTV) labelled PTVGTV, which was used for dose escalation. Margins were calculated based on the patient-specific respiratory motion obtained from the 4DCT and population based systematic and random uncertainties (see specific values below).
4D dose calculation
A schematic of the 4D calculations is available in Supplementary Figure 1 (available online at http://informahealthcare.com/doi/abs/10.3109/0284186X.2013.835492). The 3D dose distribution was recalculated in each respiratory phase and accumulated to an averaged dose distribution in order to account for the respiration-induced uncertainties. The transformations to the mid-ventilation phase were based on the deformation fields from deformable registrations of the respiratory phases using the elastixs freeware toolbox developed and validated for lung registration by Klein et al. ([Citation13], R6 with B-spline grid refinement). The registrations were visually inspected prior to further data analysis. A convolution of the dose distribution was performed with the population-averaged overall random uncertainty, which consists of the residual random components from baseline and setup uncertainties after daily image guidance (each assumed to be residual sizes of 1 mm [Citation14]).
The systematic uncertainty is a position change which for a given patient is the same for all treatment fractions. Translations of the dose cube due to the systematic uncertainty was sampled 1000 times per patient by distributing the locations of sampled points according to a Gaussion distribution. The values for the systematic uncertainties were: baseline 1 mm, setup 1 mm, and delineation 2 mm [Citation14]. All samplings and dose accumulations for the 4D dose calculations were performed in Matlab.
TCP evaluation
All the 4D calculated plans were evaluated by two TCP models with the same radiobiological model parameters and annotation (TCPM and TCPLQ) as in the previous 3D study [Citation1]. The increases in TCP values between inhomogeneous and homogeneous dose distributions were calculated for a homogeneous clonogenic cell density distributed either in the GTV or CTV.
Results
Example of results from a 4D calculation is seen in . The figure shows DVHs from both homogeneous and inhomogeneous plans for the CTV and GTV. It is seen that inclusion of the respiration only changed the DVH with a limited amount, which was common for all patients. For the selected patient, the grey region is very large due to a rather small GTV volume (9.9 cm3). It is also seen that the PTV, which might serve as a 3D surrogate for the moving target, had much lower dose values than the actual 4D calculations. The deviation between the actual DVH (grey region) and the PTV was larger for the inhomogeneous plans than for the homogeneous plans, which was common for all patients.
Figure 1. Example of DVHs for a selected patient. 3D calculations (solid line), 4D inclusion of respiration (dashed line), 4D inclusion of all random and systematic uncertainties (grey region), and 3D calculation of related PTV (dotted line). The grey region is for each dose bin given as the 10th to 90th percentile of the 4D calculated DVHs.
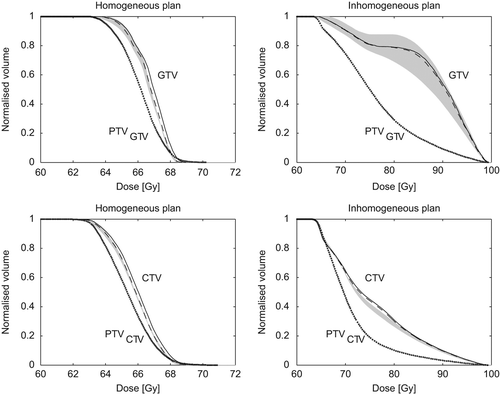
Dose coverage
Minimum, mean and maximum dose values for the two type of plans are summarised in and the patient individual values are shown in Supplementary Figure 2 (available online at http://informahealthcare.com/doi/abs/10.3109/0284186X.2013.835492). It is seen that the minimum dose for the moving CTV and GTV was larger than the corresponding minimum dose for the 3D PTVs. The mean doses were almost unchanged for the homogeneous plans while a clear increase in mean dose is seen for the inhomogeneous plans. For the maximum doses, slight decreases are seen for the homogeneous plans while the values were almost unchanged for the inhomogeneous plans.
Table I. Overview of dose and TCP values.
For the 4D calculated doses only 0.3% and 0.06% of the DVHs had minimum doses (D99%) below the 95% dose level for homogeneous and inhomogeneous plans, respectively. In an additional 4D calculation in which the respiration uncertainty was included as a convolution together with the remaining random uncertainties, the same numbers were 3% and 1%, respectively.
Changes in predicted dose due to 4D calculations for the organs at risk are shown in . Differences between predicted dose level for 4D and 3D calculations related to the dose constraints are shown together with the uncertainty of the 4D calculated average values. Very small differences (all ≤ 1.1 Gy) were observed between the average 4D value and the corresponding 3D calculation. The uncertainties on the average value from the 4D calculations are given in columns labelled “4D Dev”. For some of the organs quite large, but very similar, values were observed for both homogeneous and inhomogeneous plans.
Table II. Organs at risk.
TCP values
Increase in TCP values based on the 4D calculations between inhomogeneous and homogeneous plans are shown in for TCP calculations based on homogeneous clonogenic cells distribution in either the CTV or GTV. The error bars shown in the histograms are one standard deviation of the combined uncertainties for the homogeneous and inhomogeneous plans. For all patients, it is seen that the TCP increases based on the 3D PTV calculations were less than those based on a full 4D calculation of the related CTV or GTV.
Discussion
Four-dimensional calculations confirmed that it was possible to increase the expected local control rate by use of inhomogeneous dose escalation for all patients. The average 4D dose to critical structures were quite close to the 3D calculations as seen in . For some of the critical structures, the uncertainty on the maximum dose calculated in 4D is relatively high (e.g. 4 Gy for spinal canal). This could be critical for some patients as the maximum dose could exceed the tolerance dose. However, it should be noted that this uncertainty is almost the same for the homogeneous and inhomogeneous plans. Hence, dose-escalated inhomogeneous treatment plans are not more sensitive to the uncertainties included in this study, than the homogeneous treatment plans that have been used in standard clinical practice for many years.
The reason for the increase in minimum doses to the target in 4D calculations compared to the 3D calculations are caused by various effects: 1) dose distribution moves relative to tumour position; and 2) imperfect conformity: only a part of the target surface is at the 95% isodose level, while the remaining receives higher doses. These effects will increase the minimum dose with respect to the calculated 3D dose for the PTV. Moreover, due to the steeper dose gradients at the edge of the target in the inhomogeneous plan, the dose level slightly inside the tumour edge is higher for these plans than the corresponding homogenous plans. Thus, a low dose to the tumour in an respiration extreme and “cold dose” position is compensated by the delivered dose to the tumour in non-extreme positions. This is less difficult in the inhomogeneous plans since they on average have higher doses than the homogenous plans.
Maximum doses in homogeneous plans consist typically of small spots located in multiple places. Such spots will be reduced by the “averaging” performed during a 4D calculation and is the cause for the smaller maximum doses. For the inhomogeneous plans, the maximum dose is typically located centrally in a single volume in the target and are therefore not influenced to the same degree by the “averaging” involved in 4D calculations as the homogeneous plans are.
The changes in minimum, mean, and maximum dose values were different for the homogeneous and inhomogeneous plans. These effects make the 3D PTV less suitable to predict the dose distribution for the moving target for the inhomogeneous plans than for the homogeneous plans. This is seen in the change of TCP values shown in , which was larger than the corresponding increases based on the 3D PTV dose distributions. The increase in TCP value was actually better predicted by use of the 3D target dose distribution (GTV and CTV) than the 3D dose distribution of the related PTV.
The number of 4D DVHs with minimum doses less than 95% of prescribed dose was significantly lower when the respiratory motion was included by recalculation in all phases compared to the test in which the respiration was included as a convolution. This shows that the dose distribution is not constant in space as the tumour moves, which is assumed in the convolution approach. The same assumption was actually used in sampling of the systematic uncertainties in the current study. It is therefore expected that the actual dose distribution for the moving target is slightly higher than calculated in this study.
The effect of respiration on target DVH were quite modest in the current study. This may be related to the peak-to-peak respiration amplitude of the patients which ranges 0–12 mm. This somewhat limited range is similar to the motions observed in previous studies from our institution [Citation15,Citation16]. It can not be concluded from our study how the 4D calculations would be for a large motion, e.g. 2 cm amplitude. However, such a large amplitude is quite rare among our patients, especially for those with the lymph node involvement. The 3D DVH for the target is close to the average DVH from the 4D calculations. TCP increases are seen to be alike for the 3D and 4D calculations, thus lack of access to 4D calculations is not a hindrance to start using the advantages of inhomogeneous dose distributions, especially given that the dose to organs at risk are changed equally for the homogeneous and inhomogeneous plans when recalculated in 4D.
In summary, there were changes in calculated doses and TCP values with the 4D calculations compared to standard 3D calculations. However, the changes were small and therefore of little clinical relevance. The changes were of similar sizes in both the homogeneous and inhomogeneous treatment plans. For organs at risk, only very small dose changes were observed between the average 4D calculation and the standard 3D calculation. The similarity between TCP increases calculated in 4D and 3D is very promising since 4D recalculations are then not needed for a reliable clinical introduction of dose escalation using inhomogeneous doses.
http://informahealthcare.com/doi/abs/10.3109/0284186X.2013.835492
Download PDF (110.6 KB)Acknowledgements
This work is supported by the Region of Southern Denmark and by CIRRO – The Lundbeck Foundation Center for Interventional Research in Radiation Oncology and The Danish Council for Strategic Research.
Declaration of interest: The authors report no conflicts of interest. The authors alone are responsible for the content and writing of the paper.
References
- Nielsen TB, Hansen O, Schytte T, Brink C. Inhomogeneous dose escalation increases expected local control for NSCLC patients with lymph node involvement without increased mean lung dose. Acta Oncol 2013 Apr 29.
- Engelsman M, Remeijer P, van Herk M, Lebesque JV, Mijnheer BJ, Damen EM. Field size reduction enables iso-NTCP escalation of tumor control probability for irradiation of lung tumors. Int J Radiat Oncol Biol Phys 2001; 51:1290–8.
- Baker M, Nielsen M, Hansen O, Jahn JW, Korreman S, Brink C. Isotoxic dose escalation in the treatment of lung cancer by means of heterogeneous dose distributions in the presence of respiratory motion. Int J Radiat Oncol Biol Phys 2011;81:849–55.
- Schwarz M, Alber M, Lebesque JV, Mijnheer BJ, Damen EMF. Dose heterogeneity in the target volume and intensity-modulated radiotherapy to escalate the dose in the treatment of non-small-cell lung cancer. Int J Radiat Oncol Biol Phys 2005;62:561–70.
- Kong FM, Ten Haken RK, Schipper MJ, Sullivan MA, Chen M, Lopez C, et al. High-dose radiation improved local tumor control and overall survival in patients with inoperable/unresectable non-small-cell lung cancer: Long-term results of a radiation dose escalation study. Int J Radiat Oncol Biol Phys 2005;63:324–33.
- Belderbos JSA, Heemsbergen WD, De Jaeger K, Baas P, Lebesque JV. Final results of a Phase I/II dose escalation trial in non-small-cell lung cancer using three-dimensional conformal radiotherapy. Int J Radiat Oncol Biol Phys 2006; 66:126–34.
- Schwarz M, van der Geer J, van Herk M, Lebesque JV, Mijnheer BJ, Damen EMF. Impact of geometrical uncertainties on 3D CRT and IMRT dose distributions for lung cancer treatment. Int J Radiat Oncol Biol Phys 2006; 65:1260–9.
- Mexner V, Wolthaus JWH, van Herk M, Damen EMF, Sonke JJ. Effects of respiration-induced density variations on dose distributions in radiotherapy of lung cancer. Int J Radiat Oncol Biol Phys 2009;74:1266–75.
- Mechalakos J, Yorke E, Mageras GS, Hertanto A, Jackson A, Obcemea C, et al. Dosimetric effect of respiratory motion in external beam radiotherapy of lung. Radiother Oncol 2004; 71:191–200.
- Kavanagh A, McQuaid D, Evans P, Webb S, Guckenberger M. Dosimetric consequences of inter-fraction breathing-pattern variation on radiotherapy with personalized motion-assessed margins. Phys Med Biol 2011;56:7033–43.
- Witte M, Shakirin G, Houweling A, Peulen H, van Herk M. Dealing with geometric uncertainties in dose painting by numbers: Introducing the Δ vh. Radiother Oncol 2011;100:402–6.
- van Herk M, Remeijer P, Rasch C, Lebesque JV. The probability of correct target dosage: Dose-population histograms for deriving treatment margins in radiotherapy. Int J Radiat Oncol Biol Phys 2000;47:1121–35.
- Klein S, Staring M, Murphy K, Viergever MA, Pluim JPW. Elastix: A toolbox for intensity-based medical image registration. IEEE Trans Med Imaging 2010;29:196–205.
- Nielsen TB, Hansen VN, Westberg J, Hansen O, Brink C. A dual centre study of setup accuracy for thoracic patients based on cone-beam CT data. Radiother Oncol 2012; 102:281–6.
- Jensen HR, Hansen O, Hjelm-Hansen M, Brink C. Inter- and intrafractional movement of the tumour in extracranial stereotactic radiotherapy of NSCLC. Acta Oncol 2008; 47:1432–7.
- Gottlieb KL, Hansen CR, Hansen O, Westberg J, Brink C. Investigation of respiration induced intra- and interfractional tumour motion using a standard cone beam CT. Acta Oncol 2010;49:1192–8.