Abstract
While the detrimental effects of cranial radiotherapy on the developing brain are well known, the effects on cognitive performance of low doses of ionizing radiation is less studied. We performed a population-based cohort study to determine whether low doses of ionizing radiation to the brain in infancy affects cognitive function later in life. Further we hypothesized that the dose to the hippocampus predicts cognitive late side effects better than the anterior or the posterior brain doses.
Material and methods. During 1950–1960 3860 boys were treated with radiation in Sweden for cutaneous hemangiomas before the age of 18 months. Of these, 3030 were analyzed for military test scores at the age of 18 years and 2559 for the highest obtained educational level.
Results. Logical, spatial and technical test scores were not affected by increasing irradiation doses. The verbal test scores displayed a significant trend for decreasing scores with increasing doses to the hippocampus (p = 0.005). However, the absolute mean difference between the zero dose and the highest dose category (median 680 mGy) was very small, only 0.64 stanine points, and the significance was dependent on the highest dose category, containing few subjects. The educational level was not affected by brain irradiation. Overall, the hippocampal dose was a better predictor of late cognitive side effects than the doses to the anterior or the posterior brain. In conclusion, there was no decrease in logical, spatial and technical verbal or global test scores after ionizing radiation doses up to 250 mGy, but a subtle decrease in verbal test scores if the highest dose category was included (median 680 mGy). However, the clinical relevance of this decline in the highest dose group is questionable, since we could not find any effect on the highest obtained educational level.
It is well known that radiation therapy (RT) is a major cause of long-term complications observed in survivors of pediatric brain tumors [Citation1,Citation2]. Cognitive impairments, perturbed growth and puberty as well as secondary malignancies are some of the so-called late effects seen after RT [Citation3–6]. Cranial irradiation is associated with negative effects on a range of different cognitive functions, including attention span, working memory, processing speed and other executive functions, as well as measurable decreases in IQ scores [Citation7]. Cognitive deficits have been shown to be more severe in younger children after RT and the impairments increase progressively over time [Citation8]. However, RT is often combined with other treatment modalities and it is complicated to distinguish the effects of radiation alone from the effects of disease, surgery or chemotherapy.
Effects of low-dose radiation to the brain are less well studied. Negative influence on cognitive performance and scholastic aptitude was reported in children treated for tinea capitis, receiving an estimated mean brain dose of 1.3 Gy (mean age at treatment: 7.1 ± 3.1 years) [Citation9]. A study by Hall et al. published in 2004, demonstrated a decline in cognitive function after radiation doses lower than 1 Gy to the brains of children treated for cutaneous hemangiomas before the age of 18 months [Citation10]. The outcome of their study has been heavily debated regarding possible bias, and started a discussion on safety aspects regarding the frequently used computed tomography (CT) of the brain in children for diagnostic purposes [Citation10].
Neurogenesis in the hippocampus is thought to be important for memory and learning [Citation11]. Recent studies have addressed the contribution of hippocampal dysfunction in cognitive outcome after cranial irradiation in rodents and humans [Citation12–14]. The hippocampus is situated in the temporal lobe of the brain and human studies have shown a correlation between cognitive outcome and radiation dose to the temporal lobe in general and to the hippocampus in particular [Citation15–17]. In the study by Hall and coworkers the radiation dose to the anterior or the posterior parts of the brain was correlated to cognitive performance, but we hypothesized that the dose to the hippocampus would correlate better with the cognitive test results and therefore added the hippocampal dose to our analyses.
The aims of the present study was to validate previously published results indicating that dose levels overlapping the range of normal CT scans to the brain in infancy results in significantly decreased cognitive function [Citation10], and further to test the hypothesis that the dose to the hippocampus better predicts cognitive outcome than anterior or posterior brain doses.
Material and methods
Study population
Two cohorts of in total more than 26 000 infants treated for cutaneous hemangiomas have been established in Stockholm and Gothenburg, Sweden, to study late effects after treatment with low doses of ionizing radiation. The infants were up to 18 months old when they received their first treatment during the time period 1920–1965. In Stockholm the treatments started in 1920 and ended in 1959, in Gothenburg this treatment method started in 1930 and ended in 1965. Hall et al. studied the effects of low doses of ionizing radiation in infancy on cognitive function in adulthood in the boys of the Stockholm cohort born up to 1950 [Citation10].
In the current study, all 3860 boys born from 1950 to 1960 in the Stockholm and the Gothenburg cohorts were included. In 1950, electronic registration of military records started, replacing to the previous paper records. We only included the electronically recorded scores, whereas Hall et al. only used the paper records. Hence, there is no overlap between the current study population and that of the study published by Hall and coworkers.
The median age at the first treatment was five months. On average, the children were treated 1.3 times each.
Register linkage
All Swedes have a unique personal identification number making linkage between different registers possible. The analyses were possible by linking dosimetry data with test results from the Swedish Military Service Conscription Register and educational data from the Longitudinal Integration Database for Health Insurance and Labor Market Studies (Swedish acronym LISA). All data handling was in accordance with the ethical approval obtained from the Regional Ethical Committee in Gothenburg, Sweden (Dnr 215-05, T 019-10).
Databases
Swedish Military Service Conscription Register Data
During the study period, Swedish law required all 18-year-old Swedish men to undergo extensive and highly standardized intelligence tests and physical examinations prior to the compulsory conscription assignment in the Swedish Armed Forces. Exemptions were granted only for incarcerated males, severe chronic medical conditions, or handicaps documented by a medical certificate (2–3% of the yearly population). The cognitive performance tests are described in detail elsewhere [Citation18,Citation19] and results have been used in other studies [Citation20]. Four cognitive tests were used during the years of assessment covered by the current study: logical performance test, verbal test of synonyms and opposites, test of visuospatial/ geometric perception, and technical/mechanical skills, including mathematical/physics problems. The combined performance on all four tests yielded a global intelligence score, which was regarded as a measure of general cognitive capacity [Citation18]. The intelligence tests used in the present study use a conversion to a nine-point stanine (standard nine) scale which provided long-term stability of data sets. Central training and instruction of the psychologists who supervise the tests and the use of a standard manual helps to ensure consistency. The stanine standard scores have means of 5 and standard deviations of 2. They divide the normal curve into nine scores. The Wechsler Adult Intelligence test (WAIS), as well as many other intelligence tests, uses a scale with a mean of 100 and a standard deviation of 15. For comparison between the stanine scale and a scale with a mean of 100 and a standard deviation of 15 we used the formula: x stainine scores * 7.5 = yIQ scores [Citation21].
Longitudinal integration database for health insurance and labor market studies (Swedish acronym LISA)
The LISA database (http://www.scb.se/Pages/List____257743.aspx) includes all individuals 16 years of age and older registered in Sweden in 1990 or later. The database, which is updated annually, integrates data from the labor market, as well as educational and social sectors. Information on parental education and subsequent education of the subjects was obtained from LISA.
Radiation treatment and dosimetry
Details about the dosimetry were published previously [Citation22,Citation23]. In brief, the hemangiomas were located all over the bodies, 45% in the head and neck region, 29% on the upper trunk, 9% on the lower trunk and 17% on the extremities. The hemangiomas were mostly treated with radium needles and tubes (71%) but also with radium plaques used as β-emitters (8%), x-rays (21%) and 32P sheets (< 1%) [Citation22]. The body surface was divided into 28 different areas and the treatments were classified according to area treated. For all treatments the absorbed doses to the anterior- and posterior brain [Citation10] and to the right and left hippocampus were calculated for each patient, taking into account the duration of the exposure and the distance between the treated body surface area and the defined brain regions. For statistical analysis the mean dose of the right and left hippocampus was used in the tables. For treatments in the six body surface regions in the head and neck region the exact position of the treatment inside the region was used for the calculations. Patients were divided into three age groups, 0–4 months, 5–11 months and ≥ 12 months and the skull sizes at these ages were determined from representative magnetic resonance (MR) images. This was used to adjust the estimated absorbed doses at different ages. For distances outside the skull, data published by Lundell et al. were used [Citation22]. Earlier determined dose-rate tables were used when estimating the dose contribution from radium needles and tubes [Citation23]. For radium plaques [Citation24] and x-ray treatments (mostly contact therapy 50–60 kVp) depth dose curves were used.
Statistical methods
The mean values of the absorbed doses in the anterior brain, posterior brain and hippocampus were used in the analyses. Absorbed doses were divided into five categories: 0, 1–20, > 20–100, > 100–250 and > 250 mGy. The mean logical, verbal, spatial, technical and global scores were analyzed for different dose categories and adjusted for age at treatment and year of birth by using one-way analysis of variance. The mean test values were adjusted for age at treatment and year of birth using ANOVA and were reported with standard error (SE). P for trend < 0.05 was considered as significant.
Educational data were categorized in non-higher and higher education (completed post high school education). Logistic regression was used to model the probability of having completed higher education, using the categorical explanatory variables for estimated absorbed doses to the defined brain regions, age at treatment (0–4, 5–11 and ≥ 12 months), year of birth and parents’ education. Results from the logistic regression are presented as odds ratios with 95% confidence intervals. Likelihood ratio tests were used to assess the significance of the explanatory variables. The absorbed dose was used as a continuous variable to test the trend by dose.
Results
From the study population of 3860 boys we were able to analyze the military test scores of 3030 and further out of these the educational level of 2559 individuals (). Importantly, the largest loss to follow-up was for those tested in 1950 and 1951 (447 and 198, respectively) for technical reasons, since we excluded all individuals not electronically recorded in the registers (see also Material and methods above). A separate analysis was performed on the excluded individuals and the mean radiation dose to the different parts of the brain did not differ significantly from the rest of the population (data not shown). The numbers of individuals in each dose category are reported in . The mean and median doses to the anterior brain, posterior brain and the hippocampus for the individuals included in the military test score analyses are shown in . For individuals included in the military test score analyses, but for whom data on educational level were not available, the doses were similar (data not shown).
Figure 2. For each patient the estimated absorbed dose to the anterior brain, the posterior brain and the hippocampus was calculated. The number of subjects in each dose category (mGy) is shown, separated into the anterior brain (green), posterior brain (blue) and the hippocampus (red).
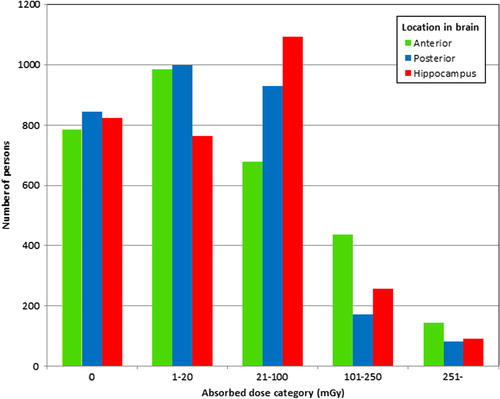
Table I. Estimated absorbed doses (mGy) to different parts of the brain. Cohort for analysis of military tests as outcome.
For the verbal test there was a trend for decreasing scores with increasing dose to the anterior part of the brain (p = 0.02) and to the hippocampus (p = 0.005) (). However, the logical, technical and spatial test scores were not affected by increasing irradiation doses, irrespective of the brain region analyzed (). When the global intelligence scores were analyzed we could not find lower scores with increasing doses, though there was a tendency for the doses to the hippocampus (p = 0.06) (). Stanine scores, as described in the methods, range from 1 (low) to 9 (high). The absolute mean test score differences between the zero dose and the > 250 mGy dose categories (median 680 mGy) in the hippocampus were: 5.39–4.98 = 0.41 (logical), 5.36–4.72 = 0.64 (verbal), 5.64–5.29 = 0.35 (spatial) and 5.14–4.76 = 0.38 (technical) (). Using a scale with a mean of 100 and a standard deviation of 15, like the WAIS and other IQ-tests, this corresponds to approximately 3.1, 4.8, 2.6 and 2.9 points, respectively. When all four tests were congregated to generate a general global intelligence score, the difference was only 5.56–5.04 = 0.52 (). Only 19% of the children to parents with non-higher education, completed higher education, as compared to 53% of the children to parents with higher education (). Higher education was not affected by brain irradiation, irrespective of dose and brain region ().
Figure 3. Results from the Swedish Military Service Conscription Register Data from the cognitive test for the 3030 subjects, separated into the five dose categories. The results are plotted based on the dose to the anterior brain (green), the posterior brain (blue) and the hippocampus (red). The tests results were adjusted for age at treatment and year of birth. The error bars indicate standard error. (A) Results from the logical test. P for trend: anterior 0.12, posterior 0.79 and hippocampus 0.09. (B) Results from the verbal test. P for trend: anterior 0.02, posterior 0.97 and hippocampus 0.005. (C) Results from the spatial test. P for trend: anterior 0.70, posterior 0.58 and hippocampus 0.35. (D) Results from the technical test. P for trend: anterior 0.45, posterior 0.81 and hippocampus 0.18. (E) Combined results from the four tests, yielding a global score. P for trend: anterior 0.23, posterior 0.91 and hippocampus 0.06.
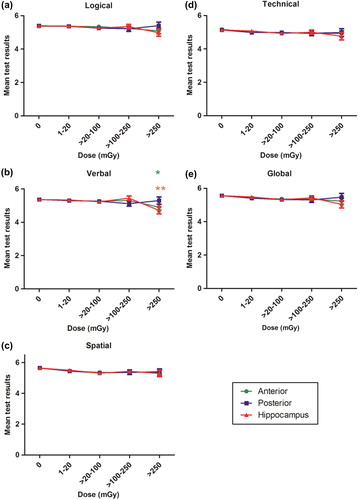
Table II. Higher education in relation to dose, part of the brain and parents’ education. The numbers represent absolute values and in brackets the proportion of individuals with higher education is shown.
Table III. Odds ratios and 95% confidence intervals of higher education, in relation to dose.
Discussion
While the detrimental effects of cranial RT on the developing brain are well known in the field of pediatric oncology [Citation6,Citation7], the effects on cognitive performance of low doses of ionizing radiation, such as for diagnostic imaging purposes have been a matter of much debate. The current study was designed to assess possible effects of low doses of ionizing radiation in infancy on cognitive performance in adulthood. The study was deliberately designed in a similar way to the study conducted by Hall et al. in 2004 to enable comparisons [Citation10].
We found no reductions in the logical, spatial, technical or global test scores for any brain region analyzed. We did find a modest reduction in verbal scores, particularly when analyzing the doses to the hippocampus. This may seem surprising, considering the proposed role for the hippocampus in spatial navigation and memory [Citation25], but is supported by a Finnish study where children, especially girls, treated with RT had lower language school grades [Citation26]. Although we found an effect of low-dose radiation on verbal cognitive performance, it should be emphasized that it was the highest dose category that contributed the most to the dose-response relationship and this category contained relatively few subjects (91 of 3030), warranting caution before making statements about causality. Importantly, higher education was not influenced by the radiation dose to the brain, irrespective of which brain region that was analyzed. The most obvious explanation for these results is that the decline in verbal test score observed was indeed very small and could be compensated for somehow, thereby making it clinically less relevant. The possibility that the endpoint higher education was not an appropriate measure to reveal existing differences must be considered.
The dose to the hippocampus showed the best correlation with reduced cognitive performance. The stronger correlation between the hippocampal radiation dose and the effects on cognition is supported by the particular radiosensitivity of the hippocampal neurogenic niche and its proposed role in memory and learning, as mentioned in the introduction [Citation11,Citation14]. In a recent paper we showed that reducing the radiation dose only to the neurogenic niches in the brain (hippocampus and subventricular zone) was estimated to ameliorate the cognitive deficits in children treated for medulloblastoma [Citation27]. In accordance with the results from Hall et al., the dose to the anterior brain correlated better with reduced test scores than the dose to the posterior brain [Citation10], but the hippocampal dose was even better than the anterior brain dose for this purpose.
The strengths of our study include the large size of the cohort, the limited loss to follow-up, the long time to follow-up, the use of independent parameters for assessment, and the use of internal controls (zero dose) to minimize selection bias. The study further allowed evaluation of the effects of radiation alone on cognitive performance, unlike the situation in patients, where it is difficult to distinguish between the relative contributions of the disease, surgery, chemotherapy and RT.
A limitation of the study is that the compulsory military conscription only included men, and it is known that at least higher doses of ionizing radiation affect the cognitive function of girls more than boys [Citation26,Citation28]. Our data were collected during a period when methods for more detailed neurocognitive assessment were still under development. More detailed neuropsychological examinations, e.g. analysis of executive functions, would likely have enabled us to draw further conclusions about the nature of the effects of low-dose radiation, if any, on cognitive performance. Children with the largest hemangiomas on the face were likely treated with the highest doses, resulting in the most mutilating scars. This may have influenced their self-esteem or confidence and thereby also their test results and performance in the educational system. Unfortunately, the nature of the collected material did not allow us to control for this possible psychological confounder.
Hall et al. reported a significant, inverse dose-response relation for logical, verbal and technical test scores but not visuospatial recognition. Further, they showed a decreasing proportion of boys attending high school with increasing doses of irradiation to the brain and concluded that cognitive performance in adulthood is influenced by low doses of irradiation to the brain in infancy. We analyzed a similar, but larger study population than Hall et al. As we could only find a dose-response relation for the verbal test scores, we could not verify their findings. However, there are some differences between the two studies. First, we stratified by parental educational level and not socioeconomic status. However, at the time of treatment (1950–1960) and testing (age 18 years old), these two concepts were assumed to be tightly linked and this difference unlikely explains the discrepancies. Second, whereas they used only the father's socioeconomic status, we used the father's or the mother's highest obtained education. Since the combination of a mother with higher education and a father without higher education was uncommon at the time, this does not likely explain the discrepancy between our results. Third, our study population was treated in a later time period, and routines might have changed over the decades. However, we did not observe any differences that could be attributed to the year of treatment within our cohort, spanning over a 10-year period. However, even though we could not explain the differences observed, we cannot exclude the possibility of an, as of yet, unidentified factor introducing bias. Importantly, the educational endpoint we used (completed post high school education) is more demanding than just high school attendance, which was used in the study by Hall et al. This would, if anything, have made it more likely for us to detect differences in scholastic aptitude, had there been any.
The potential hazard of low-dose radiation is still a research area in great need of solid data. The current study allowed unbiased assessment of doses in the range from 0 up to about 500 mGy median dose (). For comparison, the dose from a CT brain scan after 2001 in a child is in the range of 28–44 mGy [Citation29]. A CT brain scan is a powerful and sometimes vital diagnostic tool, but in addition to the risk of cognitive effects, the risk of secondary malignancies should also be considered [Citation29].
In conclusion, we found no significant effects on logical, spatial, technical or global test scores after low doses of ionizing radiation. For verbal test scores, there was an inverse dose-response relation with increasing doses to the hippocampus, but the absolute decline in test score was low and strongly dependent on the contribution of the highest dose category. We could not detect any influence on the highest obtained education and hence the clinical relevance of the subtle cognitive verbal decline should be interpreted with great caution. To the best of our knowledge, there are no similar studies conducted outside Sweden, and it remains to be shown if the results can be generalized to other parts of the world. Further investigations to address this question are warranted. Nevertheless, our results expand the knowledge of low-dose radiation to the brain and will hopefully contribute to risk assessments and future guidelines in pediatric diagnostic radiation.
Acknowledgments
This work was supported by the Swedish Research Council, the Swedish Childhood Cancer Foundation, the King Gustav the Vth Jubilee Clinic Cancer Foundation (JK-fonden), the Frimurare Barnhus Foundation, Swedish governmental grants to scientists working in health care (ALF), the Swedish Cancer Foundation, the Wilhelm and Martina Lundgren Foundation, Edit Jacobsons Donations Foundation, and the Gothenburg Medical Society.
Declaration of interest: The authors report no conflicts of interest. The authors alone are responsible for the content and writing of the paper.
References
- Mulhern RK, Merchant TE, Gajjar A, Reddick WE, Kun LE. Late neurocognitive sequelae in survivors of brain tumours in childhood. Lancet Oncol 2004;5:399–408.
- Pollack IF, Jakacki RI. Childhood brain tumors: Epidemiology, current management and future directions. Nature Rev Neurol 2011;7:495–506.
- Packer RJ, Gurney JG, Punyko JA, Donaldson SS, Inskip PD, Stovall M, et al. Long-term neurologic and neurosensory sequelae in adult survivors of a childhood brain tumor: Childhood cancer survivor study. J Clin Oncol 2003;21: 3255–61.
- Lannering B, Marky I, Lundberg A, Olsson E. Long-term sequelae after pediatric brain tumors: Their effect on disability and quality of life. Med Pediatr Oncol 1990;18:304–10.
- Neglia JP, Robison LL, Stovall M, Liu Y, Packer RJ, Hammond S, et al. New primary neoplasms of the central nervous system in survivors of childhood cancer: A report from the Childhood Cancer Survivor Study. J Natl Cancer Inst 2006;98:1528–37.
- Armstrong GT, Jain N, Liu W, Merchant TE, Stovall M, Srivastava DK, et al. Region-specific radiotherapy and neuropsychological outcomes in adult survivors of childhood CNS malignancies. Neuro Oncol 2010;12:1173–86.
- Rodgers SP, Trevino M, Zawaski JA, Gaber MW, Leasure JL. Neurogenesis, exercise, and cognitive late effects of pediatric radiotherapy. Neural Plast 2013;2013:698528.
- Palmer SL, Armstrong C, Onar-Thomas A, Wu S, Wallace D, Bonner MJ, et al. Processing speed, attention, and working memory after treatment for medulloblastoma: An international, prospective, and longitudinal study. J Clin Oncol 2013;31:3494–500.
- Ron E, Modan B, Floro S, Harkedar I, Gurewitz R. Mental function following scalp irradiation during childhood. Am J Epidemiol 1982;116:149–60.
- Hall P, Adami HO, Trichopoulos D, Pedersen NL, Lagiou P, Ekbom A, et al. Effect of low doses of ionising radiation in infancy on cognitive function in adulthood: Swedish population based cohort study. Br Med J 2004;328:19.
- Shors TJ, Miesegaes G, Beylin A, Zhao M, Rydel T, Gould E. Neurogenesis in the adult is involved in the formation of trace memories. Nature 2001;410:372–6.
- Kalm M, Karlsson N, Nilsson MK, Blomgren K. Loss of hippocampal neurogenesis, increased novelty-induced activity, decreased home cage activity, and impaired reversal learning one year after irradiation of the young mouse brain. Exp Neurol 2013;247:402–9.
- Rola R, Raber J, Rizk A, Otsuka S, VandenBerg SR, Morhardt DR, et al. Radiation-induced impairment of hippocampal neurogenesis is associated with cognitive deficits in young mice. Exp Neurol 2004;188:316–30.
- Monje ML, Vogel H, Masek M, Ligon KL, Fisher PG, Palmer TD. Impaired human hippocampal neurogenesis after treatment for central nervous system malignancies. Ann Neurol 2007;62:515–20.
- Armstrong GT, Liu Q, Yasui Y, Huang S, Ness KK, Leisenring W, et al. Long-term outcomes among adult survivors of childhood central nervous system malignancies in the Childhood Cancer Survivor Study. J Natl Cancer Inst 2009;101:946–58.
- Jalali R, Mallick I, Dutta D, Goswami S, Gupta T, Munshi A, et al. Factors influencing neurocognitive outcomes in young patients with benign and low-grade brain tumors treated with stereotactic conformal radiotherapy. Int J Radiat Oncol Biol Phys 2010;77:974–9.
- Gondi V, Hermann BP, Mehta MP, Tome WA. Hippocampal dosimetry predicts neurocognitive function impairment after fractionated stereotactic radiotherapy for benign or low-grade adult brain tumors. Int J Radiat Oncol Biol Phys 2012;83:e487–93.
- Carlstedt B. Cognitive abilities – aspects of structure, process and measurement. [Thesis]. Acta Universitatis Gothoburgensis. Gothenburg: University of Gothenburg; 2000.
- David AS, Malmberg A, Brandt L, Allebeck P, Lewis G. IQ and risk for schizophrenia: A population-based cohort study. Psychol Med 1997;27:1311–23.
- Gunnell D, Magnusson PK, Rasmussen F. Low intelligence test scores in 18 year old men and risk of suicide: Cohort study. Br Med J 2005;330:167.
- Salvia J, Ysseldyke JE. Assessment. 8th ed. Boston: Houghton Mifflin; 2001. xxiv, 712 p.
- Lundell M, Furst CJ, Hedlund B, Holm LE. Radium treatment for hemangioma in early childhood. Reconstruction and dosimetry of treatments, 1920–1959. Acta Oncol 1990; 29:551–6.
- Lundell M. Estimates of absorbed dose in different organs in children treated with radium for skin hemangiomas. Radiat Res 1994;140:327–33.
- Larsson H. Results of radiotherapy of epibulbar tumors at Radiumhemmet, Stockholm, 1920–1940. Acta Radiolog 1946;27:358–73.
- Buzsaki G, Moser EI. Memory, navigation and theta rhythm in the hippocampal-entorhinal system. Nature Neurosci 2013;16:130–8.
- Lahteenmaki PM, Harila-Saari A, Pukkala EI, Kyyronen P, Salmi TT, Sankila R. Scholastic achievements of children with brain tumors at the end of comprehensive education: A nationwide, register-based study. Neurology 2007;69: 296–305.
- Blomstrand M, Brodin NP, Munck Af Rosenschold P, Vogelius IR, Sanchez Merino G, Kiil-Berthlesen A, et al. Estimated clinical benefit of protecting neurogenesis in the developing brain during radiation therapy for pediatric medulloblastoma. Neuro Oncol 2012;14:882–9.
- Waber DP, Gioia G, Paccia J, Sherman B, Dinklage D, Sollee N, et al. Sex differences in cognitive processing in children treated with CNS prophylaxis for acute lymphoblastic leukemia. J Pediatr Psychol 1990;15:105–22.
- Pearce MS, Salotti JA, Little MP, McHugh K, Lee C, Kim KP, et al. Radiation exposure from CT scans in childhood and subsequent risk of leukaemia and brain tumours: A retrospective cohort study. Lancet 2012;380: 499–505.