Abstract
Background. In radiotherapy, the magnitude of respiratory-induced tumor motion is often measured using a single four-dimensional computed tomography (4DCT). This magnitude is required to determine the internal target volume. The aim of this study was to compare the magnitude of respiratory-induced motion of pancreatic tumors on a single 4DCT with the motion on daily cone beam CT (CBCT) scans during a 3–5-week fractionated radiotherapy scheme. In addition, we investigated changes in the respiratory motion during the treatment course.
Material and methods. The mean peak-to-peak motion (i.e. magnitude of motion) of pancreatic tumors was measured for 18 patients using intratumoral gold fiducials visible on CBCT scans made prior to each treatment fraction (10–27 CBCTs per patient; 401 CBCTs in total). For each patient, these magnitudes were compared to the magnitude measured on 4DCT. Possible time trends were investigated by applying linear fits to the tumor motion determined from daily CBCTs as a function of treatment day.
Results. We found a significant (p ≤ 0.01) difference between motion magnitude on 4DCT and on CBCT in superior-inferior, anterior-posterior and left-right direction, in 13, 9 and 12 of 18 patients, respectively. In the anterior- posterior and left-right direction no fractions had a difference ≥ 5 mm. In the superior-inferior direction the difference was ≥ 5 mm for 17% of the 401 fractions. In this direction, a significant (p ≤ 0.05) time trend in tumor motion was observed in 4 of 18 patients, but all trends were small (− 0.17–0.10 mm/day) and did not explain the large differences in motion magnitude between 4DCT and CBCT.
Conclusion. A single measurement of the respiratory-induced motion magnitude of pancreatic tumors using 4DCT is often not representative for the magnitude during daily treatment over a 3–5-week radiotherapy scheme. For this patient group it may be beneficial to introduce breath-hold to eliminate respiratory-induced tumor motion.
Neoadjuvant radiochemotherapy has a potential benefit in resectable and borderline resectable pancreatic cancer [Citation1]. This treatment, however, is highly toxic due to the high radiation dose levels to surrounding organs at risk (OARs), such as stomach, duodenum and kidneys. Reducing the size of the planning target volume (PTV) may help spare these OARs.
The size of the PTV is partially determined by the amount of respiratory-induced motion of the tumor. The respiratory-induced motion magnitude, investigated for various tumor sites [Citation2,Citation3], is for the pancreas reported to be 5–15 mm [Citation2,Citation4–8]. The motion magnitude is often measured only once before treatment planning [e.g. using four-dimensional computed tomography (4DCT)]. However, tumor motion can vary from day to day and even from one respiratory cycle to the next, so a single measurement can be misrepresenting and might lead to insufficient target coverage [Citation9]. Therefore, thorough investigation is needed to determine whether the tumor motion as represented on planning CT is a good predictor for the tumor motion during daily treatment.
For the pancreas, very few studies have investigated the predictive value of 4DCT [Citation10,Citation11]. Ge et al. investigated 3–5 respiratory cycles per measurement [Citation11]. However, respiratory motion is known to change over short periods of time [Citation12,Citation13]. Minn et al. used hundreds of cycles per measurement, but compared each 4DCT with only a single (Cyberknife) fraction [Citation10]. With respiratory motion also changing over longer periods of time [Citation12,Citation13], their work is not representative for fractionated treatments of several weeks [Citation10]. Daily CBCTs enable investigation of magnitudes of and trends in the respiratory-induced pancreatic tumor motion over the complete course of treatment.
We studied the respiratory-induced tumor motion in 18 pancreatic cancer patients using 4DCT and daily CBCTs over a 3–5-week fractionated treatment. Tumor motion was measured using intratumoral gold fiducials. The aim of this study was to compare the magnitude of respiratory-induced motion of pancreatic tumors using a single 4DCT, with daily CBCT scans during a fractionated radiation treatment scheme. In addition, we investigated trends in the respiratory motion during the treatment course.
Material and methods
Patients and treatment
From October 2010 to August 2013, 18 consecutive patients (11 males and 7 females) with a pancreatic carcinoma, who had received a 4DCT scan and were treated with radiotherapy, were included in this retrospective study. All patients, with a mean age of 65 years (range, 46–79), underwent an endoscopic ultrasound-guided implantation of 2–4 intratumoral gold fiducial markers to enable daily CBCT position verification. Three patients received Gold Anchor fiducials (Naslund Medical, Huddinge, Sweden; thickness, 0.28 mm; length, 1 cm) and the remaining patients were implanted with Visicoil markers (RadioMed, Barlett, TN; thickness, 0.35 mm; length, 0.5–2.0 cm). Patient 16 received a Gold Anchor as well as a Visicoil fiducial. Patients 1–16 were also included in earlier studies of our group on interfractional tumor position variations [Citation14,Citation15]. The corresponding patient numbering in these papers [Citation14,Citation15] with the present paper can be found in Supplementary Table A1 (available online at http://informahealthcare.com/doi/abs/10.3109/0284186X.2014.905699). Marker motion is representative for tumor motion since no migration of the fiducials over the course of treatment was observed [Citation14].
Patient 18 had a tumor in the pancreatic tail, patient 4 in the pancreatic body/tail, patient 9 in the pancreatic body and all others in the pancreatic head. Patients were treated with radiochemotherapy using three different schemes. The prescription dose for patients 9, 14 and 16 was 28 × 1.8 Gy, for patients 17 and 18 it was 15 × 2.4 Gy and for all other patients 25 × 2.0 Gy. All treatment schemes were combined with concurrent chemotherapy (Supplementary Table A1 available online at http://informahealthcare.com/doi/abs/10.3109/0284186X.2014.905699).
All included patients received a 4DCT scan (LightSpeed TR16 system, General Electric Company, Waukesha WI, USA), taken under normal breathing conditions. The respiratory cycle was divided into 10 bins, resulting in 10 4DCT phase scans (slice thickness, 2.5 mm; pixel size, 1.0 × 1.0 mm2). For patients 1 and 3 the 4DCT scan was made at a later stage than the planning CT scan (on day 30 and 10, respectively, with the planning CT on day 0). All patients received CBCT imaging (Synergy, Elekta Oncology systems, Crawley, UK) prior to each treatment fraction. Thirty-five of the planned treatment fractions were not delivered due to early termination of treatments and three CBCTs were not retrievable, resulting in 10–27 scans per patient (mean, 22; 401 CBCTs in total).
The magnitude of respiratory-induced marker motion (MM), defined as the mean peak-to-peak motion of the markers due to respiration along one of the main axes, was determined for the 4DCT scans (MM4DCT) as well as for each CBCT (MMCBCT), in superior-inferior (S-I), anterior- posterior (A-P) and left-right (L-R) direction.
4DCT
A 4DCT scan took about 90 seconds and for every couch position a single respiratory cycle was used. To measure MM4DCT, the 10 phase scans were individually matched. This was done by using the end-inhale (EI) phase scan as a reference and performing rigid registrations of the other nine phase scans to this reference scan, based on the markers and using translations only. The obtained translation values gave the excursion of the markers throughout the respiratory cycle in all three directions. In each direction, the difference between the most extreme translations was considered as MM4DCT.
CBCT
The CBCT scans were reconstructed from kV projection images that were made while the system rotated around the patient. Each scan took approximately 2 minutes and produced about 700 projection images over a full 360° rotation, during which the patient was breathing freely. To determine MMCBCT, we made reconstructions of the EI and end-exhale (EE) phases. We selected the projections belonging to each of these phases by extracting the respiratory signal from the projection images.
Extraction of the respiratory signal was done using an in-house developed program written in MATLAB (The MathWorks Inc., Natick, MA, USA). The sum of the pixel values over a region of interest (ROI) within the projections was plotted against the gantry angle ( and ). Three main components contributed to the obtained signal: gantry angle (causing changes in attenuation), cardiac motion and respiratory-induced motion [Citation16,Citation17]. The contribution of the gantry rotation was filtered out by applying a moving average filter (sliding-window width of 31 data points) and subtracting this filtered signal from the original signal. The contribution of the cardiac motion was filtered out by applying a second moving average filter (sliding-window width of 5 data points), leaving the respiratory signal ( and ). A similar method was described by Kavanagh et al. [Citation16].
Figure 1. Sum of pixel values over the ROI divided by the mean sum over all gantry angles (a, b) and the same signal after filtering out the change in attenuation and cardiac motion (c, d). The values on the vertical axes are given in arbitrary units (a.u.).
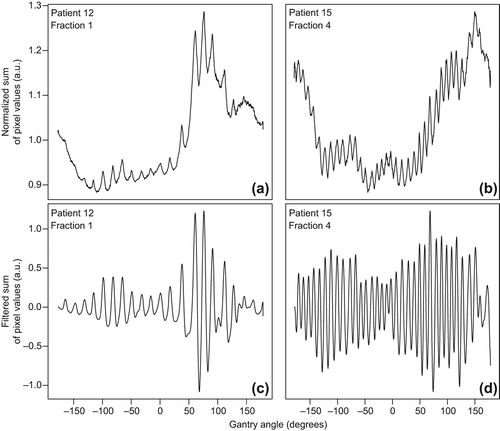
The acquired signal contained phase and frequency of the respiratory signal, but no information on the amplitude of respiratory-induced motion of the markers. By selecting the projection images corresponding to the peaks and troughs in the signal, 3D CBCT reconstructions were made of the patient in the EE and the EI phase, respectively. Both reconstructions contained between 20 and 40 projections depending on the frequency of respiration. Even though this resulted in low-quality reconstructions, blurred in part due to the cardiac motion, the fiducials remained clearly visible. We matched the reconstructions to the planning CT by rigid registration on the fiducial markers, using translations only. The differences in translation values for the two registrations gave MMCBCT in all three directions.
Motion magnitude measurement validation
For validation of the performance of the MMCBCT procedure, MM was also obtained directly from individual projections (MMCBproj) using a procedure described by Marchant et al. [Citation18]. By manually selecting the positions of the fiducials in every single projection image and applying a coordinate transformation to go from CBCT-panel coordinates to patient coordinates, the true 3D marker motion could be determined. After the marker motion during CBCT acquisition was obtained, the peak-to-peak motion of every respiratory cycle was determined and then averaged to yield MMCBproj. The marker motion in the S-I direction is along the axis of the gantry rotation and can therefore be measured during the complete CBCT acquisition. In L-R and in A-P, the motion is perpendicular to this axis and can be measured during small parts of the CBCT acquisition only; therefore L-R and A-P were not taken into account for this validation.
This procedure was very time consuming (about 10 hours per CBCT). MMCBproj was measured for seven fractions from five patients, randomly chosen, and we checked the performance of the (MATLAB) MMCBCT program by analyzing the absolute differences between MMCBCT and MMCBproj in the S-I direction for each CBCT.
Comparison 4DCT and CBCT data
In order to determine whether MM4DCT was a good predictor for MMCBCT, we tested for each patient whether the mean MMCBCT was equal to MM4DCT using a one-sample Wilcoxon rank sum test. To examine the relevance of the difference between MM4DCT and MMCBCT, we calculated for each patient the absolute difference between MM4DCT and each MMCBCT value (Δ) in all three directions separately and determined the numbers of fractions for which Δ ≥ 3 mm and Δ ≥ 5 mm. Also, a paired Student's t-test was used to evaluate the difference between the mean MM4DCT and the average of the mean MMCBCT values (normality of the data was tested using the Shapiro-Wilk test).
To test whether MMCBCT was constant over the course of treatment, we plotted for each patient MMCBCT as a function of treatment day and applied linear fits to determine the behavior of MMCBCT over time. All analyses were performed in the three directions and all statistical analyses were done using R version 3.0.1 (R Foundation for Statistical Computing, USA).
Results
The mean MM4DCT was 8.3 (SD 3.3) mm, 2.9 (1.0) mm and 2.4 (1.1) mm in S-I, A-P and L-R direction, respectively. The mean MMCBCT over all patients was 7.0 (SD 2.8) mm, 2.2 (0.8) mm and 1.5 (0.8) mm, respectively ().
Figure 2. 4DCT (red solid lines) and CBCT data (boxplots) of respiratory-induced fiducial marker motion magnitude (MM) in (a) superior-inferior, (b) anterior-posterior and (c) left-right direction.
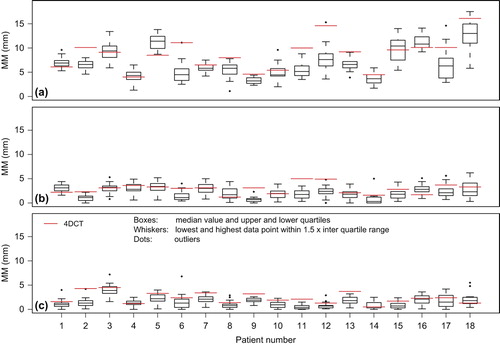
In the S-I direction, the mean absolute difference, after post-processing of the data, between MMCBCT and MMCBproj over the seven evaluated CBCTs was 0.6 mm (details can be found in the Supplementary material, available online at http://informahealthcare.com/doi/abs/10.3109/0284186X.2014.905699). This was used as a validation for the used measurement procedures.
In the S-I direction, tumor motion on 4DCT was significantly different from the mean motion during the treatment itself for 13 of 18 patients (p ≤ 0.01; ). We found Δ ≥ 3 mm for 36% and Δ ≥ 5 mm for 17% of the 401 fractions. The mean MM4DCT was significantly larger than the average mean MMCBCT (p = 0.012). MM4DCT seems therefore not a good predictor for daily tumor motion when used for creating PTVs.
Table I. Tumor motion in superior-inferior direction, comparing 4DCT with daily CBCT.
In the A-P and L-R direction, tumor motion on 4DCT was significantly different from the mean motion during the treatment itself for 9 and 12 of 18 patients, respectively (p ≤ 0.01). Δ was ≥ 3 mm for 6% and 5%, respectively, and in both directions there were no fractions with Δ ≥ 5 mm. The mean MM4DCT was significantly larger than the average mean MMCBCT in both directions, p = 0.021 and p = 0.001 in A-P and L-R, respectively.
We found that 16, 10 and 9 of the 18 trend lines had a negative slope [ and Supplementary Figures B1–B3 (available online at http://informahealthcare.com/doi/abs/10.3109/0284186X.2014.905699)], in S-I, A-P and L-R, respectively, indicating that the respiratory-induced motion magnitude decreased over time. The largest slope that was found was − 0.17 mm/day for patient 15 in the S-I direction. Nine patients had a significant slope (p ≤ 0.05) in one direction; for three of these patients the absolute slope was larger than 0.1 mm/day.
Figure 3. Marker motion in the superior-inferior direction during a CBCT, for 3 fractions (a–c). Circles indicate projections selected by the program to make end-inhale (open) and end-exhale (closed) phase reconstructions.
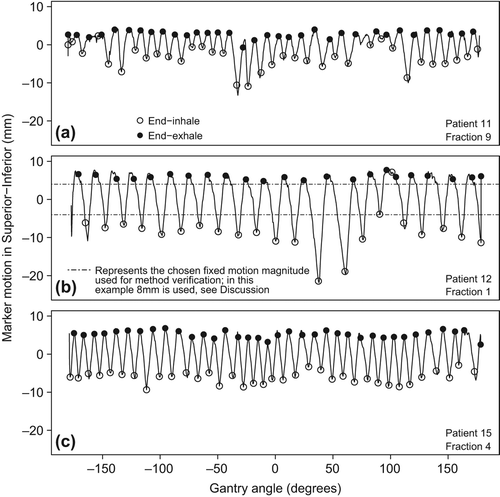
Figure 4. MMCBCT values (closed symbols) in superior-inferior direction for patients 8 (a) and 15 (b), plotted as function of treatment day (day 0 is the day of planning CT). Lines are linear fits to the MMCBCT data; slopes (mm/day) are indicated in the legends (*p < 0.05; **p < 0.01; ***p < 0.001). MM4DCT values (open symbols) were not included in the fit.
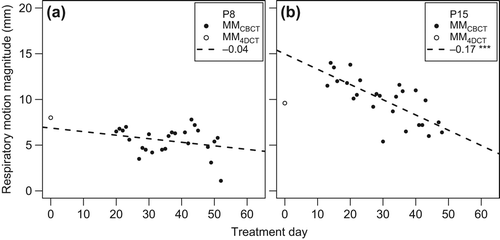
Discussion
We compared tumor motion in pancreatic cancer patients on 4DCT to tumor motion on daily CBCT using intratumoral gold fiducials. The present study was the first to include such a large number of patients and use daily measurements during a 3–5-week treatment course. This resulted in an extensive dataset with up to 28 data points per patient, allowing us to make a comprehensive comparison of the tumor motion during treatment planning and treatment and to detect time trends in tumor motion during treatment, which have not been reported for pancreatic tumors so far. The mean motion magnitude was 8.3 (SD 3.3) mm on 4DCT and 7.0 (SD 2.8) mm on CBCT. Our study showed that for the majority of the evaluated patients the tumor motion at treatment planning was not representative for the tumor motion during a 3–5-week fractionated treatment.
The algorithm by Kavanagh et al., similar to our algorithm used to extract the respiratory signal from the CBCT projections, has been shown to compare well to the Amsterdam shroud procedure [Citation16]. We validated our method to determine MMCBCT by comparing MMCBCT to MMCBproj for seven CBCTs and found small differences. As an additional validation we determined MMCBCT for a chosen fixed magnitude of motion. This was done by making two 3D-CBCT reconstructions from projections for which the marker was at one of two preselected positions (e.g. -4 and 4 mm in , so that the expected MMCBCT was 8 mm) and compared the obtained MMCBCT to this fixed magnitude of motion. This was done for two CBCTs and three values of MMCBCT (6, 8 and 10 mm). Differences between the expected MMCBCT and the determined MMCBCT were small (up to 0.3 mm) when using these selected projections. This residual error was most likely due to uncertainties in the image registration.
The used algorithm did not always identify the correct projections as EE or EI. For two CBCTs we manually selected the correct EE and EI projections using the marker motion shown in and and found a maximum discrepancy of 0.3 mm for MMCBCT. Even though the reconstructions were in a specific respiratory phase, the markers may still have been blurred due to the variation in marker position in EE or EI during a single CBCT acquisition; shows a variation in EI marker position of 18 mm. This blurring combined with the inability of the program to always select the correct projections led to an uncertainty estimated to be < 1 mm (SD) in the values of MMCBCT. For the detection of fiducials in the CBCT projection images no automated procedures (e.g. as proposed by Fledelius et al. [Citation19]) were used. Implementing such procedures would be difficult for this patient group because small fiducials, as used in this study, produce very low contrast on the projection images. Also, 12 of 18 patients had a biliary stent very close to the fiducials, further compromising automatic marker detection for these patients.
The measured respiratory-induced motion of pancreatic tumors was similar to the 5–15 mm reported in the literature [Citation2,Citation4–8]. Our results show that the respiratory-induced motion during 4DCT is often not representative for the motion during daily CBCT measurements. No additional analyses were performed to determine dependences on tumor location or stage of the disease, as patient numbers were too small to do so. Only three patients had a tumor located somewhere else than the pancreatic head.
In the S-I direction, MM4DCT was significantly different from the mean MMCBCT in 13 of 18 patients. This was in agreement with two papers that compared the 4DCT results of respiratory-induced motion of the pancreas to daily measurements [Citation10,Citation11]. Both found significant differences between tumor motion at treatment planning and during daily treatment, as we did for considerably more measurements per patient and during all daily fractions of the 3–5-week treatment, with each measurement consisting of at least 20 respiratory cycles.
Respiratory-induced motion is not very regular or uniform. The peak-to-peak motion can vary greatly during CBCT acquisition due to irregular breathing [Citation5] and a single value for MM is not necessarily representative for the entire motion. In addition, the actual MM during irradiation can again be different from the MM during CBCT acquisition. Also for 4DCT, irregular breathing patterns can influence the measured MM. For liver and lung patients, with a mean peak-to-peak motion of 20 mm, SDs of mean tumor location of 1.7 and 3.0 mm were found in EE and EI, respectively, due to irregular breathing patterns [Citation20]. This could lead to a suboptimal treatment when the incorporation of respiratory-induced motion in the PTV is based on such a single measurement. What can be seen from our data is that the fiducial position in EE is much more reproducible than in EI; this is clearly visible in .
For 10 patients the MM4DCT was significantly larger than the MMCBCT in the S-I direction. Also, the mean MM4DCT was significantly larger than the average mean MMCBCT for this patient group. Overestimating the respiratory-induced motion during treatment would lead to too large a PTV. The observation that tumor motion during 4DCT was larger than tumor motion during treatment has been reported for other tumor sites as well, e.g. for lungs by Britton et al., who reported that a single 4DCT may not be sufficient to determine an ITV for highly mobile tumors [Citation21]. In addition, a considerable variation in absolute amplitude of the tumor motion in the lung was reported [Citation22]. For a 30-minute period directly following a 4DCT scan, it was found that the scan could sufficiently predict respiratory- induced lung tumor motion [Citation23].
The observed time trends were small and did not explain the discrepancy between treatment planning and daily treatment. The largest significant slope resulted in a decrease of MMCBCT of 5.8 mm, in S-I direction, over the complete course of treatment (patient 15). The negative slopes could be due to patients relaxing more as the treatment progresses, as was also speculated by Seppenwoolde et al. [Citation3]. Other papers reported non-significant time trends in the magnitude of respiratory-induced motion over time, e.g. in lung cancer patients [Citation24].
Using MM4DCT as a measure for daily tumor motion could lead to sub-optimal PTVs. Our results suggest that introducing a form of active breath control such as tracking or voluntary breath-hold may be beneficial for irradiation of patients with pancreatic cancer. That way, the differences in motion magnitude between 4DCT, used for margin determination, and daily treatment could be eliminated. A remaining margin of 5 mm is reported to be sufficient to account for all position variations in EE breath-hold for pancreatic cancer patients [Citation25].
In conclusion, the respiratory-induced motion of pancreatic tumors measured with 4DCT was often not representative for the tumor motion during treatment measured using daily CBCTs, as they differed significantly in the majority of patients. Also, tumor motion was variable throughout the treatment. Time trends, however small, suggested that tumor motion can change as the treatment progresses. Our results show the limitations of using a single 4DCT to take into account the patient-specific respiratory-induced pancreatic tumor motion when doing treatment planning. Introducing breath-hold may be beneficial for pancreatic cancer patients.
Supplementary Figures B1–B3
Download PDF (2.6 MB)Acknowledgments
This work was supported by the foundation Bergh in het Zadel through the Dutch Cancer Society (KWF Kankerbestrijding) project no. UVA 2011–5271.
Declaration of interest: The authors report no conflicts of interest. The authors alone are responsible for the content and writing of the paper.
Dr Bel does consultancy work for Nucletron. Dr van Hooft reports personal fees from Boston Scientific and Cook Ireland Ltd. Dr Fockens reports personal fees from Cook Ireland Ltd, Boston Scientific, Olympus Medical Systems and Fujifilm, outside the submitted work.
References
- Van Tienhoven G, Gouma DJ, Richel DJ. Neoadjuvant chemoradiotherapy has a potential role in pancreatic carcinoma. Ther Adv Med Oncol 2011;3:27–33.
- Wysocka B, Kassam Z, Lockwood G, Brierley J, Dawson LA, Buckley CA, et al. Interfraction and respiratory organ motion during conformal radiotherapy in gastric cancer. Int J Radiat Oncol Biol Phys 2010;77:53–9.
- Seppenwoolde Y, Shirato H, Kitamura K, Shimizu S, Van Herk M, Lebesque JV, et al. Precise and real-time measurement of 3D tumor motion in lung due to breathing and heartbeat, measured during radiotherapy. Int J Radiat Oncol Biol Phys 2002;53:822–34.
- Gwynne S, Wills L, Joseph G, John G, Staffurth J, Hurt C, et al. Respiratory movement of upper abdominal organs and its effect on radiotherapy planning in pancreatic cancer. Clin Oncol (R Coll Radiol) 2009;21:713–9.
- Mori S, Hara R, Yanagi T, Sharp GC, Kumagai M, Asakura H, et al. Four-dimensional measurement of intrafractional respiratory motion of pancreatic tumors using a 256 multi-slice CT scanner. Radiother Oncol 2009;92:231–7.
- Goldstein SD, Ford EC, Duhon M, McNutt T, Wong J, Herman JM. Use of respiratory-correlated four-dimensional computed tomography to determine acceptable treatment margins for locally advanced pancreatic adenocarcinoma. Int J Radiat Oncol Biol Phys 2010;76:597–602.
- Whitfield G, Jain P, Green M, Watkins G, Henry A, Stratford J, et al. Quantifying motion for pancreatic radiotherapy margin calculation. Radiother Oncol 2012;103:360–6.
- Hallman JL, Mori S, Sharp GC, Lu H, Hong TS, Chen GTY. A four-dimensional computed tomography analysis of multiorgan abdominal motion. Int J Radiat Oncol Biol Phys 2012;83:435–41.
- Gierga DP, Chen GTY, Kung JH, Betke M, Lombardi J, Willet CG. Quantification of respiration-induced abdominal tumor motion and its impact on IMRT dose distributions. Int J Radiat Oncol Biol Phys 2004;58:1584–95.
- Minn AY, Schellenberg D, Maxim P, Suh Y, McKenna S, Cox B, et al. Pancreatic tumor motion on a single planning 4D-CT does not correlate with intrafraction tumor motion during treatment. Am J Clin Oncol 2009;32:364–8.
- Ge J, Santanam L, Noel C, Parikh PJ. Planning 4-dimensional computed tomography (4DCT) cannot adequately represent daily intrafractional motion of abdominal tumors. Int J Radiat Oncol Biol Phys 2013;85:999–1005.
- Ozhasoglu C, Murphy MJ. Issues in respiratory motion compensation during external-beam radiotherapy. Int J Radiat Oncol Biol Phys 2002;52:1389–99.
- Bussels B, Goethals L, Feron M, Bielen D, Dymarkowski S, Suetens P, et al. Respiration-induced movement of the upper abdominal organs: A pitfall for the three-dimensional conformal radiation treatment of pancreatic cancer. Radiother Oncol 2003;68:69–74.
- Van der Horst A, Wognum S, Dávila Fajardo R, de Jong R, van Hooft JE, Fockens P, et al. Interfractional position variation of pancreatic tumors quantified using intratumoral fiducial markers and daily cone beam computed tomography. Int J Radiat Oncol Biol Phys 2013;87:202–8.
- Van der Horst A, Lens E, Wognum S, de Jong R, van Hooft JE, van Tienhoven G, et al. Limited role for biliary stent as surrogate fiducial marker in pancreatic cancer: stent and intratumoral fiducials compared. Int J Radiat Oncol Biol Phys DOI: 10.1016/ j.ijrobp.2014.03.029
- Kavanagh A, Evans PM, Hansen VN, Webb S. Obtaining breathing patterns from any sequential thoracic x-ray image set. Phys Med Biol 2009;54:4879–88.
- Vergalasova I, Cai J, Yin F-F. A novel technique for markerless, self-sorted 4D-CBCT: Feasibility study. Med Phys 2012;39:1442–51.
- Marchant TE, Amer AM, Moore CJ. Measurement of inter and intra fraction organ motion in radiotherapy using cone beam CT projection images. Phys Med Biol 2008;53:1087–98.
- Fledelius W, Worm E, Elstrøm UV, Petersen JB, Grau C, Høyer M, et al. Robust automatic segmentation of multiple implanted cylindrical gold fiducial markers in cone-beam CT projections. Med Phys 2011;38:6351–61.
- Sarker J, Chu A, Mui K, Wolfgang JA, Hirsch AE, Chen GTY, et al. Variations in tumor size and position due to irregular breathing in 4D-CT: A simulation study. Med Phys 2010;37:1254–60.
- Britton KR, Starkschall G, Tucker SL, Pan T, Nelson C, Chang JY, et al. Assessment of gross tumor volume regression and motion changes during radiotherapy for non-small-cell lung cancer as measured by four-dimensional computed tomography. Int J Radiat Oncol Biol Phys 2007;68:1036–46.
- Shirato H, Suzuki K, Sharp GC, Fujita K, Onimaru R, Fujino M, et al. Speed and amplitude of lung tumor motion precisely detected in four-dimensional setup and in real-time tumor-tracking radiotherapy. Int J Radiat Oncol Biol Phys 2006;64:1229–36.
- Guckenberger M, Wilbert J, Meyer J, Baier K, Richter A, Flentje M. Is a single respiratory correlated 4D-CT study sufficient for evaluation of breathing motion?Int J Radiat Oncol Biol Phys 2007;67:1352–9.
- Hugo G, Vargas C, Liang J, Kestin L, Wong JW, Yan D. Changes in the respiratory pattern during radiotherapy for cancer in the lung. Radiother Oncol 2006;78:326–31.
- Nakamura M, Shibuya K, Shiinoki T, Matsuo Y, Nakamura A, Nakata M, et al. Positional reproducibility of pancreatic tumors under end-exhalation breath-hold conditions using a visual feedback technique. Int J Radiat Oncol Biol Phys 2011;79:1565–71.