ABSTRACT
Background. The benefit of proton therapy may be jeopardized by dose deterioration caused by water equivalent path length (WEPL) variations. In this study we introduced a method to evaluate robustness of proton therapy with respect to inter-fractional motion and applied it to irradiation of the pelvic lymph nodes (LNs) from different beam angles. Patient- versus population-specific patterns in dose deterioration were explored.
Material and methods. Patient data sets consisting of a planning computed tomography (pCT) as well as multiple repeat CT (rCT) scans for three patients were used, with target volumes and organs at risk (ORs) outlined in all scans. Single beam spot scanning proton plans were optimized for the left and right LN targets separately, across all possible beam angle configurations (5° angle intervals). Isotropic margins of 0, 3, 5 and 7 mm from the clinical target volume (CTV) to the planning target volume (PTV) were investigated. The optimized fluence maps for the pCT for each beam were applied onto all rCTs and the dose distributions were re-calculated. WEPL variation for each beam angle was computed by averaging over beams eye view WEPL distributions.
Results. Similarity in deterioration patterns were found for the investigated patients, with beam angles delivering less dose to rectum, bladder and overall normal tissue identified around 40° and around 150°–160° for the left LNs, and corresponding angles for the right LNs. These angles were also associated with low values of WEPL variation.
Conclusion. We have established and explored a method to quantify the robustness towards inter-fractional motion of single beam proton plans treating the pelvic LNs from different beam configurations and with different CTV to PTV margins. For the patients investigated we were able to identify beam orientations that were robust to dose deterioration in the target and ORs.
Prostate cancer is the most frequent cancer disease among men, with increasing incidence during the past two decades [Citation1,Citation2]. A considerable fraction of these patients show biochemical failure with varying recurrence patterns [Citation3–5]. For patients with locally advanced prostate cancer, radiotherapy (RT) is an important part of the loco-regional treatment, with radiation also prescribed to the pelvic lymph nodes (LNs) [Citation3,Citation6,Citation7]. Currently, intensity-modulated radiotherapy (IMRT) is often used to deliver doses to the pelvic LNs, however, there appears to be a considerable normal tissue (bowel and bladder) sparing potential if treating these large volumes with proton therapy [Citation8–11].
Due to the favorable depth dose distribution compared to conventional photon-based RT, proton therapy has in general a potential for better sparing of normal tissues. Proton therapy for locally advanced prostate cancer in particular has been shown to decrease the doses delivered to the organs at risk (ORs) compared to contemporary photon-based therapy [Citation9,Citation12–14]. However, the finite range of protons in the tissue (referred to as the Bragg peak) makes the treatment sensitive to any changes in patient anatomy that modulates the density of tissue in the beam path towards the target volumes, including organ motion or in particular changes in bony anatomy [Citation10]. In addition to introducing positional variations, these changes lead to modifications in the water equivalent path length (WEPL) which translate into range uncertainties for each (part of the) beam. These uncertainties may be difficult to account for using current image guidance strategies; treatment plans for proton therapy should therefore be made robust to internal motion.
Several studies have addressed the issue of improving plan and outcome robustness of pelvic tumor sites for photon-based RT [Citation11,Citation15,Citation16]. For proton therapy, the effects of pelvic organ motion may be more pronounced, leading to target dose degradation with respect to the plan [Citation10,Citation17]. In order to minimize dose degradation, several groups have investigated variations in WEPL as input to proton planning, as well as delivery and verification strategies to improve robustness towards intra- and inter-fractional changes. A pre-defined range uncertainty interval has been included directly into the plan optimization [Citation18–20]. For scattering techniques methods to reduce or account for dose degradation have included both range compensators and in vivo measurements [Citation21,Citation22]. However, little work has been done to investigate whether beam angle selection can be used to identify robust angles and also whether these angles would be patient- or population-specific. The aim of this study was therefore to develop a method to explore robustness of pelvic LN irradiation using proton beams with different orientation, with respect to anatomical variations (in the target shapes, differences in the contents of the GI tract and in bladder filling). Patient- versus population-specific patterns in the resulting dose deterioration were also explored.
Material and methods
Patient cohort and image material
The study included three patients with locally advanced prostate cancer treated with IMRT at Haukeland University Hospital, Bergen, Norway, in 2007. Each patient underwent computed tomography (CT) scanning (Prospeed SX Power, GE Medical Systems, Milwaukee, WI, USA) for treatment planning (pCT) as well as 7–9 additional CTs (rCTs) evenly distributed along the RT course. All CT scans were acquired with 2–3 mm slice thickness, image size of 512 × 512 pixels and extended from L4 vertebrae to the anal vertex. All image acquisitions were performed with the patient in supine position, and using the same fixation device as in the treatment. Further details of the treatment protocol were described by Muren et al. [Citation23].
For the purpose of this study, a radiation oncologist delineated the involved clinical target volumes (CTVs, i.e. the prostate, seminal vesicles and the pelvic LNs) as well as the rectum and bladder in all CT scans. Delineations were performed using the treatment planning system (TPS) Eclipse v.11 (Varian Medical Systems, Palo Alto, CA, USA). Further information of the contouring process was published by Thörnqvist et al. [Citation24].
Treatment planning and beam angle evaluation
To evaluate LN dose coverage we separated the left and right part of the LN targets. For each patient and each volume, we optimized single beam spot scanning proton plans using the TPS TRiP v.1001c [Citation25,Citation26]. Gantry angles covered in 5° steps the ranges from 0° to 180° for the left LN volume and from 180° to 360° for the right LN volume (avoiding contralateral radiation). Couch angles covered the interval between -90° and 90°, in 5° steps. Finally a 55° cone was excluded at the cranial-caudal ends, corresponding to the CT limits. To optimize the single beam proton plans we used pencil beams with 5 mm spot size (full width at half maximum) and 2 mm spot spacing. We applied the Gaussian blurred pencil beam method without any correction for internal scattering. Different isotropic margins from the LN CTV to the planning target volume (PTV) of 0, 3, 5 and 7 mm were added [Citation27], and the above planning process was repeated for each margin alternative. All treatment planning operations were performed in PyTRiP [Citation28].
To assess target (physical) dose coverage for the LNs for the explored beam angle configurations, the optimized fluence maps from the pCT were transferred onto the respective rCTs to perform dose re-calculations for all relevant volumes. For each patient, a rCT-pCT alignment was performed for each rCT using the center of mass of the prostate as treatment isocenter, prior to plan transfers and dose re-calculations. Dose distributions for each beam angle configuration at the pCT and at rCT were represented as dose-volume histograms (DVHs). Finally, dose coverage for optimized and recalculated plans were compared by the differences in the V99 (volume percentage receiving 99% of the dose) and V95 for the LN target volumes; dose evaluations for the normal tissue and the ORs (rectum and bladder) were done using V10 [Citation27].
WEPL variations
We evaluated inter-fractional WEPL variations (between pCT and each rCT) for each beam angle configuration. For each gantry-couch angle configuration, the WEPL was calculated at the pCT and rCTs for each beam path. WEPL values were projected (sum values for all voxels along the ray path) into a normal plane to the beam, placed at the center of the target. The standard deviation of the projected WEPL was then computed for all beams to account for WEPL variations between the pCT and rCTs [Citation25]; finally, the mean variation over the whole beam was calculated. WEPL variations were calculated for the same beam angle combinations used for the treatment plan calculations, i.e. gantry angles varying from 0° to 180° or 180° to 360° (left and right LN, respectively) and couch angles varying from −90° to 90°, in 5° steps; again excluding the cranial-caudal limits of the CTs. Finally, mean WEPL variations across the CT data sets and at all beam angle configurations were shown in two-dimensional (2D) maps for each patient. All LN target dose coverage changes as well as WEPL variations were analyzed using in-house routines developed in Matlab v.R2015a (The MathWorks Inc., Natick, MA, USA).
Patient clustering comparison
To make an overall analysis across the three patients concerning the association between dose deviation endpoints and WEPL variation maps, normalized log2 transformation of the data was done. These maps were calculated to illustrate the correlation between WEPL variations and dose target degradation in order to identify robust beam angles.
To investigate variation between CTs and patients across all angles for each volume of interest (VOI) (and margin choice) of each patient, a cluster comparison analysis was made [Citation29] by normalizing the log2 transformed volume percentages for the given dose percentages along each angle between all CTs; then, the most significant angles were selected by an F-test with p < 0.001 significance level. These angles were compared using Pearson's correlation, both across CTs of each patient and across angles. This method determined potential patient and inter- fractional patterns in the similar angles, which can be interpreted as a measure of robustness. This analysis was performed in the open-source software package R (www.r-project.org).
Results
Overall we found beam angles with the least dose degradation for both the right and left LNs at the most anterior and posterior angles (). For the three patients included in this study, dose degradation of the LN targets at the V99 level across all beam angles was found to range between 58% and 96%, 80–100%, 83–100% and 82–100% for the 0, 3, 5 and 7 mm margin alternatives, respectively (). For normal tissue overall V10 was found to range from 3% to 9%, 4–10%, 4–12% and 4–11% for the four margins (0, 3, 5 and 7 mm, respectively). Corresponding numbers for rectum V10 ranged from 0% to 12%, 0–19% 0–18% and 0–7%, while they were generally larger for the bladder, with V10 ranging from 0% to 11%, 0–16%, 1–25% and 2–30%. For lateral angles we found the highest dose delivered to normal tissue and rectum, ranging between 9% and 12% and 7–19% for V10 at angles between 60° and 120° and at around 180°, respectively (couch angle = 0°). The largest doses delivered to the bladder were found for anterior angles (gantry-couch angle = 0°) with the V10 ranging between 11% and 30%, increasing with increasing margin.
Figure 1. DVH of one patient (referred to as Patient 1), for the left LNs with a 5 mm margin. (Left panel) DVHs for single beam proton plans optimized on the pCT and normalized to give the D99% at V99% for the left side of the lymph node volume. (Middle panels) DVHs for the pCT fluence maps applied over the 10 different rCTs. (Right panel) All DVHs in one plot.
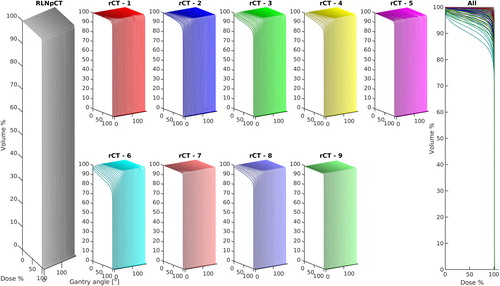
Figure 2. Boxplots of V99% for the LNs and V10% for the NT, rectum and bladder for isotropic margins of 0, 3, 5 and 7 mm for all CTs. For each margin and volume, the mean, quartiles, confidence bounds with outliers as well as the minimum and maximum are shown along with the corresponding angle.
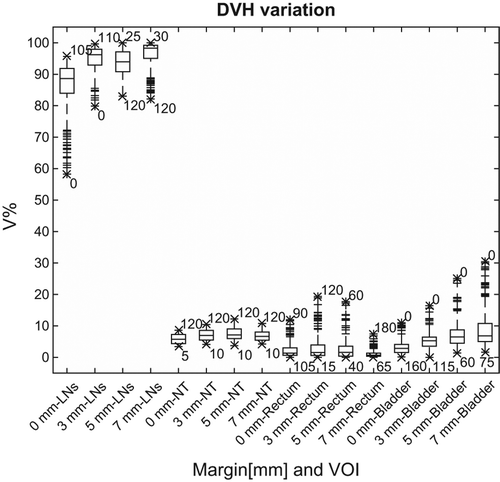
The WEPL maps for the three patients showed similar patterns as the dose deterioration, with the largest WEPL variation identified at the lateral angles. The mean variation corresponded to a systematic difference in the WEPL, and was found to vary from 0.5 mm up to 6 mm for angles on the same side as the LNs and up to 7 mm for contralateral angles ( and Supplementary Figures 1 and 2, available online at http://informahealthcare.com/doi/abs/10.3109/0284186X.2015.1067720).
Figure 3. Mean variation in WEPL for one of the patients (Patient 1). Angles lower than 55° from the patient axis centered in the prostate were excluded, according to CT limits and physically possible angles. The variation is given in (mm) of water.
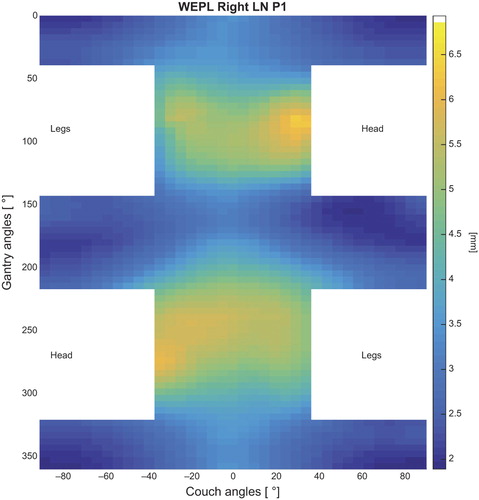
The complete data comparison showed all data of one patient for the different volume percentages (V10 for ORs and normal tissue, and V99 for target volumes) and the WEPL maps log2 transformed and normalized (). The most robust gantry angles for these three patients were at 25°–35° and 160°–165° for the left LNs and at 325°–340° and 185°–205° for the right LNs.
Figure 4. WEPL data and DVH data at V99% for the right LNs and at V10% for NT, rectum and bladder. All data normalized to units of standard deviation per dataset, the V% of the LNs are plotted in negative to be comparable on the same scale, meaning the lower value the “better”. At the bottom is the mean of all data, thus in units of mean standard deviation.
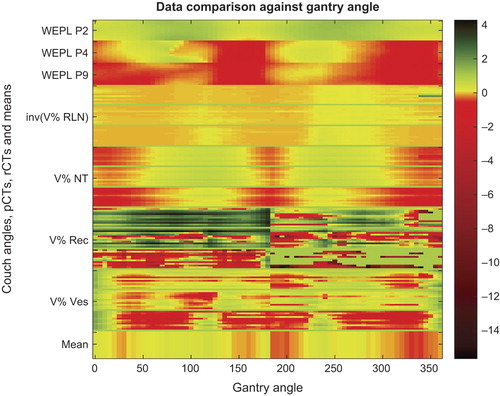
The clustering analysis identified similarities between patients across the rCTs. For the left LNs Patients 1 and 2 were more alike for angles between 105° and 140°, which also was the region with most variation between patients. For angles 50°–100° and 170°–180° Patients 1 and 3 were closer related. However, angles between 0° and 45° and 145°–165° was more consistent across all rCTs of the three patients (). For the right LNs a symmetric pattern of similarity was observed, although with a lower level of variance around the angles consistent across all rCTs.
Figure 5. Data clustering, at V99% of three patients on the left LNs after log2 transformation, normalization, and selection of most significant angles for p < 0.001 sorted with Pearson's correlation on the y-axis, and all CTs sorted by Pearson's correlation on the x-axis. The branch length in the trees corresponds to the Pearson distance.
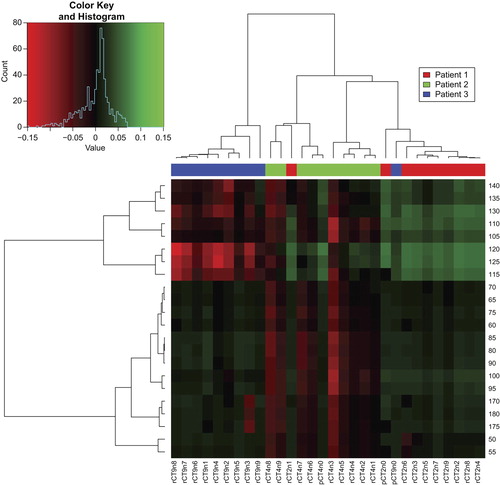
Discussion
In this study we have presented a method to evaluate the robustness of proton therapy beams towards inter-fractional changes during the course of therapy, for all relevant beam orientations, and for four different CTV-to-PTV margin alternatives. Using this method we could identify both patient- and population-specific patterns in where the most robust (as well as sensitive) beam directions are.
In proton therapy for prostate cancer (so far mostly used to treat localized disease) most protocols have used lateral opposing beams [Citation10,Citation30]. However, in this study we have seen that these angles might be less robust to the density/WEPL changes caused by internal organ motion during the therapy course involving LN irradiation. Posterior-oblique beams (10°–20° from vertical) and anterior-oblique beams (15°–25° from vertical), to the respective lymph node volume, might be better to use in order to minimize dose variation. These beams showed less degradation for target doses and less dose delivered to the ORs and normal tissue compared to lateral angles. This is caused by small variations in the ray paths going through high-density structures being translated into large differences in the proton beam range, such as lateral beams through the pelvic bones. In the study of Thörnqvist et al. [Citation10], the plans consisted of two lateral opposing beams covering the prostate, seminal vesicles and LNs. These plans were found to be sensitive to inter-fractional changes, in particular for cases with pronounced bladder and rectum variations. Cuaron et al. [Citation31] used anterior beam trajectories for proton therapy for prostate cancer patients with hip replacement or previously irradiated hips, obtaining similar volume percentages for ORs.
Our results also indicate that a 7 mm margin may be sufficient for some patients to secure full/adequate target coverage regarding inter-fractional movement. The 5 mm margins also showed good target coverage, except for the most anterior angles. For these angles, and for this margin alternative, the bladder filling and LN shape and size have the largest influence on the proton range. The 0 mm margin alternative was also explored, showing large dose degradations, where the most sensitive angles were found to be at the lateral positions due to beam paths through the pelvic bones (see Supplementary Material for details available online at http://informa healthcare.com/doi/abs/10.3109/0284186X.2015.1067720).
The WEPL maps showed large variation around the lateral angles, which is in agreement with the beam configurations presenting the highest dose degradation for targets and normal tissue/ORs. Indeed, this variation pattern was similar across the three patients (Supplementary Figure 1 available online at http://informahealthcare.com/doi/abs/10.3109/0284186X.2015.1067720). No clear pattern differentiating patients were found, however there seems to be more similarity between Patients 1 and 2 in the WEPL analysis, these patients have a steeper WEPL ‘valley’ around beams at 0° couch angle for contralateral gantry angles than Patient 3 (see Supplementary Figure 2 available online at http://informahealthcare.com/doi/abs/10.3109/0284186X.2015.1067720). Exploring a larger patient cohort might show correlation with WEPL pattern and patient population-specific angles.
The WEPL differences seem to have both a ‘random’ and a ‘systematic’ error, the latter indicated by the mean variation, although clear conclusions can only be drawn from analyzing a larger number of patients. The symmetry and small change in the WEPL variation for beam angles from the opposite site indicate that the bony structures have the largest influence on the WEPL, as these are almost symmetric, while the soft tissue difference is more variable when using angles from the opposite side.
The patient comparison by clustering of Pearson's correlation between angles identified the same pattern as suggested by the WEPL maps. Where Patients 1 and 2 seem to be in the same subtree for all tissues, Patient 3 primarily stands alone in another subtree (). For instance, we found the 0°–45° and 145°–165° gantry angles to have the lowest variance in the F-test – they are consistent across all CTs, however only 145°–165° is robust as the more anterior angles 0°–45° have a large dose degradation. This pattern might also indicate that patient sub-population-specific angles might be considered as there are visible differences in the dose variation for these subgroups.
Our results are also dependent on the image-guidance simulation process. Dose re-calculations over the rCTs were based on placing the beam isocenter at the center of the prostate to simulate clinical practice (as these plans would also irradiate the prostate and seminal vesicles). The robustness for the LN target would potentially be improved with positioning on bony anatomy, at the cost of a larger prostate CTV margin to cover prostate motion relative to bony anatomy [Citation10,Citation32]. It is also important to point out that the assumption taken in photon-based RT of the invariance of the dose distribution to any (clinically relevant) isocenter shift is unlikely to be valid in proton therapy [Citation33–35]. In future work we will also investigate approaches for dose- and image-guided proton therapy.
One the same note; this study has been based on a rCT data set. However, image-guidance for proton therapy based on on-board cone beam computed tomography (CBCT) imaging is emerging. Apart from the ongoing technical development of this area (with first prototypes currently being introduced into clinics by the main proton therapy vendors), there are also important challenges related to the suitability of CBCT scans for dose-based image guidance. Solutions such as deformable image registration based stopping power ratio propagation from pCTs to CBCTs should be further investigated.
The present results showed some beam directions more robust to inter-fractional motion when treatment optimizations were performed using single beams. With this method and by using WEPL calculations on a larger group of patients we will investigate more in depth the population- versus patient-specific patterns of robustness with respect to gantry/couch angles and margin. In these future steps we will also study the robustness of multiple beam plans with respect to inter-fractional motion. Finally, we will explore patient-specific methods for directional/spot-specific margins in LN irradiation with proton therapy.
In conclusion, we have established and explored a method to identify beam angle robustness to inter-fractional motion of single beam proton plans treating the pelvic LNs from different gantry/couch angle combinations. This method may be used to identify robust beam angles and margin configurations suitable for either individual or groups of patients in proton therapy involving pelvic LN irradiation.
Supplementary material available online
Supplementary Figure 1–2 available online at http://informahealthcare.com/doi/abs/10.3109/0284186X.2015.1067720
ionc_a_1067720_sm3646.pdf
Download PDF (484.9 KB)Acknowledgments
Bjarne Thomsen, Dept. of Physics and Astronomy, Aarhus University, is thanked for providing cluster computing time. Niels Bassler, Dept. of Physics and Astronomy, Aarhus University, is thanked for his advice and maintenance on the cluster computer system. Michael Krämer, GSI Biophysik, Darmstadt, is acknowledged for his advice and for providing TRiP98, the treatment planning system used in this study.
Declaration of interest: The authors report no conflicts of interest. The authors alone are responsible for the content and writing of the paper.
References
- Delaney G, Jacob S, Featherstone C, Barton M. The role of radiotherapy in cancer treatment: Estimating optimal utilization from a review of evidence-based clinical guidelines. Cancer 2005;104:1129–37.
- Bray F, Lortet-Tieulent J, Ferlay J, Forman D, Auvinen A. Prostate cancer incidence and mortality trends in 37 European countries: An overview. Eur J Cancer 2010;46: 3040–52.
- Créhange G, Chen CP, Hsu CC, Kased N, Coakley FV, Kurhanewicz J, et al. Management of prostate cancer patients with lymph node involvement: A rapidly evolving paradigm. Cancer Treat Rev 2012;38:956–67.
- Heemsbergen WD, Al-Mamgani A, Slot A, Dielwart MFH, Lebesque JV. Long-term results of the Dutch randomized prostate cancer trial: Impact of dose-escalation on local, biochemical, clinical failure, and survival. Radiother Oncol 2014;110:104–9.
- Kok D, Gill S, Bressel M, Byrne K, Kron T, Fox C, et al. Late toxicity and biochemical control in 554 prostate cancer patients treated with and without dose escalated image guided radiotherapy. Radiother Oncol 2013;107:140–6.
- Fonteyne V, Lumen N, Ost P, Van Praet C, Vandecasteele K, De Gersem Ir W, et al. Hypofractionated intensity-modulated arc therapy for lymph node metastasized prostate cancer: Early late toxicity and 3-year clinical outcome. Radiother Oncol 2013;109:229–34.
- McDonald AM, Jacob R, Dobelbower MC, Kim RY, Fiveash JB. Efficacy and toxicity of conventionally fractionated pelvic radiation with a hypofractionated simultaneous versus conventionally fractionated sequential boost for patients with high-risk prostate cancer. Acta Oncol 2013; 52:1181–8.
- Vargas C, Wagner M, Mahajan C, Indelicato D, Fryer A, Falchook A, et al. Proton therapy coverage for prostate cancer treatment. Int J Radiat Oncol Biol Phys 2008;70: 1492–501.
- Widesott L, Pierelli A, Fiorino C, Lomax AJ, Amichetti M, Cozzarini C, et al. Helical tomotherapy vs. intensity-modulated proton therapy for whole pelvis irradiation in high-risk prostate cancer patients: Dosimetric, normal tissue complication probability, and generalized equivalent uniform dose analysis. Int J Radiat Oncol Biol Phys 2011;80:1589–600.
- Thörnqvist S, Muren LP, Bentzen L, Hysing LB, Høyer M, Grau C, et al. Degradation of target coverage due to inter-fraction motion during intensity-modulated proton therapy of prostate and elective targets. Acta Oncol 2013;52:521–7.
- Thörnqvist S, Bentzen L, Petersen JBB, Hysing LB, Muren LP. Plan robustness of simultaneous integrated boost radiotherapy of prostate and lymph nodes for different image-guidance and delivery techniques. Acta Oncol 2011; 50:926–34.
- Fellin F, Azzeroni R, Maggio A, Lorentini S, Cozzarini C, Di Muzio N, et al. Helical tomotherapy and intensity modulated proton therapy in the treatment of dominant intraprostatic lesion: A treament planning comparison. Radiother Oncol 2013;107:207–12.
- Chera BS, Vargas C, Morris CG, Louis D, Flampouri S, Yeung D, et al. Dosimetric study of pelvic proton radiotherapy for high-risk prostate cancer. Int J Radiat Oncol Biol Phys 2009;75:994–1002.
- Henderson RH, Hoppe BS, Marcus RB, Mendenhall WM, Nichols RC, Li Z, et al. Urinary functional outcomes and toxicity five years after proton therapy for low- and intermediate-risk prostate cancer: Results of two prospective trials. Acta Oncol 2013;52:463–9.
- Thor M, Apte A, Deasy JO, Muren LP. Statistical simulations to estimate motion-inclusive dose-volume histograms for prediction of rectal morbidity following radiotherapy. Acta Oncol 2013;52:666–75.
- Thörnqvist S, Hysing LB, Zolnay AG, Söhn M, Hoogeman MS, Muren LP, et al. Treatment simulations with a statistical deformable motion model to evaluate margins for multiple targets in radiotherapy for high-risk prostate cancer. Radiother Oncol 2013;109:344–9.
- Vees H, Dipasquale G, Nouet P, Zilli T, Cozzi L, Miralbell R. Pelvic lymph node irradiation including pararectal sentinel nodes for prostate cancer patients: Treatment optimization comparing intensity modulated x-rays, volumetric modulated arc therapy, and intensity modulated proton therapy. Technol Cancer Res Treat 2015;14:181–9.
- Trofimov A, Nguyen PL, Efstathiou JA, Wang Y, Lu H-M, Engelsman M, et al. Interfractional variations in the setup of pelvic bony anatomy and soft tissue, and their implications on the delivery of proton therapy for localized prostate cancer. Int J Radiat Oncol Biol Phys 2011;80:928–37.
- Lu H-M. A potential method for in vivo range verification in proton therapy treatment. Phys Med Biol 2008;53:1413–24.
- Fredriksson A, Forsgren A, Hårdemark B. Minimax optimization for handling range and setup uncertainties in proton therapy. Med Phys 2011;38:1672–84.
- Unkelbach J, Chan TCY, Bortfeld T. Accounting for range uncertainties in the optimization of intensity modulated proton therapy. Phys Med Biol 2007;52:2755–73.
- Unkelbach J, Bortfeld T, Martin BC, Soukup M. Reducing the sensitivity of IMPT treatment plans to setup errors and range uncertainties via probabilistic treatment planning. Med Phys 2009;36:149–63.
- Muren LP, Wasbø E, Helle SI, Hysing LB, Karlsdottir A, Odland OH, et al. Intensity-modulated radiotherapy of pelvic lymph nodes in locally advanced prostate cancer: Planning procedures and early experiences. Int J Radiat Oncol Biol Phys 2008;71:1034–41.
- Thörnqvist S, Petersen JBB, Høyer M, Bentzen LN, Muren LP. Propagation of target and organ at risk contours in radiotherapy of prostate cancer using deformable image registration. Acta Oncol 2010;49:1023–32.
- Casares-Magaz O, Toftegaard J, Muren LP, Kallehauge JF, Bassler N, Poulsen PR, et al. A method for selection of beam angles robust to intra-fractional motion in proton therapy of lung cancer. Acta Oncol 2014;53:1058–63.
- Krämer M, Jäkel O, Haberer T, Rietzel E, Schardt D, Scholz M, et al. Treatment planning for scanned ion beams. Radiother Oncol 2004;73(Suppl 2):S80–5.
- Adamczyk M, Piotrowski T, Adamiak E, Malicki J. Dosimetric consequences of prostate-based couch shifts on the precision of dose delivery during simultaneous IMRT irradiation of the prostate, seminal vesicles and pelvic lymph nodes. Phys Med 2014;30:228–33.
- Toftegaard J, Petersen JBB, Bassler N. PyTRiP – a toolbox and GUI for the proton/ion therapy planning system TRiP. J Phys Conf Ser 2014;489:012045.
- Chaikh A, Giraud J-Y, Perrin E, Bresciani J-P, Balosso J. The choice of statistical methods for comparisons of dosimetric data in radiotherapy. Radiat Oncol 2014;9:205.
- Trofimov A, Nguyen PL, Coen JJ, Doppke KP, Schneider RJ, Adams JA, et al. Radiotherapy treatment of early-stage prostate cancer with IMRT and protons: A treatment planning comparison. Int J Radiat Oncol Biol Phys 2007;69:444–53.
- Cuaron JJ, Harris AA, Chon B, Tsai H, Larson G, Hartsell WF, et al. Anterior-oriented proton beams for prostate cancer: A multi-institutional experience. Acta Oncol 2015;54:868–74.
- Meijer GJ, de Klerk J, Bzdusek K, van den Berg HA, Janssen R, Kaus MR, et al. What CTV-to-PTV margins should be applied for prostate irradiation? Four-dimensional quantitative assessment using model-based deformable image registration techniques. Int J Radiat Oncol Biol Phys 2008;72:1416–25.
- Engelsman M, Schwarz M, Dong L. Physics controversies in proton therapy. Semin Radiat Oncol 2013;23:88–96.
- Cheung JP, Park PC, Court LE, Ronald Zhu X, Kudchadker RJ, Frank SJ, et al. A novel dose-based positioning method for CT image-guided proton therapy. Med Phys 2013;40: 051714.
- Park PC, Cheung JP, Zhu XR, Lee AK, Sahoo N, Tucker SL, et al. Statistical assessment of proton treatment plans under setup and range uncertainties. Int J Radiat Oncol Biol Phys 2013;86:1007–13.