Abstract
Background: Severe acute radiation-induced skin toxicity (RIST) after breast irradiation is a side effect impacting the quality of life in breast cancer (BC) patients. The aim of the present study was to develop normal tissue complication probability (NTCP) models of severe acute RIST in BC patients.
Patients and methods: We evaluated 140 consecutive BC patients undergoing conventional three-dimensional conformal radiotherapy (3D-CRT) after breast conserving surgery in a prospective study assessing acute RIST. The acute RIST was classified according to the RTOG scoring system. Dose-surface histograms (DSHs) of the body structure in the breast region were extracted as representative of skin irradiation. Patient, disease, and treatment-related characteristics were analyzed along with DSHs. NTCP modeling by Lyman-Kutcher-Burman (LKB) and by multivariate logistic regression using bootstrap resampling techniques was performed. Models were evaluated by Spearman’s Rs coefficient and ROC area.
Results: By the end of radiotherapy, 139 (99%) patients developed any degree of acute RIST. G3 RIST was found in 11 of 140 (8%) patients. Mild-moderate (G1-G2) RIST was still present at 40 days after treatment in six (4%) patients. Using DSHs for LKB modeling of acute RIST severity (RTOG G3 vs. G0-2), parameter estimates were TD50=39 Gy, n=0.38 and m=0.14 [Rs = 0.25, area under the curve (AUC) = 0.77, p = 0.003]. On multivariate analysis, the most predictive model of acute RIST severity was a two-variable model including the skin receiving ≥30 Gy (S30) and psoriasis [Rs = 0.32, AUC = 0.84, p < 0.001].
Conclusions: Using body DSH as representative of skin dose, the LKB n parameter was consistent with a surface effect for the skin. A good prediction performance was obtained using a data-driven multivariate model including S30 and a pre-existing skin disease (psoriasis) as a clinical factor.
Most patients with early breast cancer (BC) are treated with conservative surgery followed by radiation therapy (RT) on the residual ipsilateral breast and, if appropriate, on nodal sites. During or after breast RT, radiation-induced skin toxicity (RIST) is one of the predominant side effects. Skin erythema, breast edema and breast fibrosis are common side effects of external beam RT to the breast cumulatively occurring in more than 90% of all patients [Citation1]. The skin is an organ characterized by a continuous renewing of its components. Germinative epidermal cells, hair matrix cells and fibroblasts, and endothelial cells are the main targets damaged by ionizing radiation, with subsequent inflammation, apoptosis and vascular alterations that result in acute skin toxicity [Citation2].
The incidence of RIST may be increased by concomitant chemotherapy [Citation3]. Breast target volume and smoking habit have been shown [Citation4] to be associated with acute skin toxicity after a multivariate analysis. Phototype has been related to late alterations of skin pigmentation rather than acute toxicity [Citation5].
The knowledge of factors affecting acute RIST may help to prevent and manage many of the complications linked to the local treatment of BC and impacting the quality of life of the patients. Furthermore, severe acute skin reactions may be prodromal of consequential late effects [Citation6,Citation7]. Prediction and, possibly, prevention of these acute reactions may also avoid late effects. Prediction of acute RIST may be even more important considering the frequent use of hybrid surgery techniques (oncoplastics) aimed to preserve the esthetical appearance of the breast.
Modeling of normal tissue complication probability (NTCP) has been performed with different techniques for many organs and end-points. However, such formalism was not commonly adopted for evaluating toxicity and dose to the skin [Citation8] and all the available NTCP modeling studies for breast fibrosis are based on the dose-volume histogram from the breast volume [Citation9–11].
In the present study, we prospectively evaluated acute RIST in a cohort of patients undergoing breast irradiation after conserving surgery for BC. Clinical and dosimetric predictors of acute RIST were evaluated. We adopted two different approaches for NTCP modeling: Lyman-Kutcher-Burman (LKB) and a data-driven multivariate analysis. Dose-surface histograms (DSHs) of the body structure (Body) in the breast region were assumed as representative of the irradiation in thin skin layers.
Material and methods
Patients and treatments
Sequential patients affected by localized BC and undergoing radiotherapy after breast conserving surgery at the Department of Radiation Oncology of the University “Federico II” of Naples were prospectively enrolled in the study. History and physical examination, coupled to a dermatologic check assessing phototype and possible pathologic skin conditions were performed.
The phototype classification was recorded according to Fitzpatrick methodology [Citation12] which defines six different phototypes, one and six being the most and the less sensitive to sun exposure, respectively. Briefly, the patients were inspected for eyes, hair and skin color, presence of freckles and asked about skin reactions to the sun exposure. On the basis of the collected information, a score was generated. The diagnosis of any pre-existing skin diseases was based on history and skin inspection of the patient. In particular, the presence of skin alterations characterized by erythema, plaques, scaling and pruritus in body sites, such as elbow, knees, scalp, and sacral region were the criteria used to define patients affected by psoriasis [Citation13]. The diagnosis of psoriasis was subsequently clinically confirmed by a dermatologist to whom the patients have been referred. Information on adjuvant pharmacological treatment and the therapy administered to the patient was registered. Additional data like smoking habit and breast target volume were also collected. All participants gave written informed consent and the patient data were analyzed anonymously.
The radiation treatment to the breast was delivered as previously described by three-dimensional conformal radiation (3D-CRT) therapy using two tangent 6 MV fields [Citation14]. Plans were generated using a commercial treatment planning system (XiO 4.64, Elekta CMS) with the multigrid superposition dose calculation algorithm incorporating electron contamination component in the build-up region. Field segments were used if needed to optimize dose distribution, so as 95% of the prescription dose was delivered to at least 95% of the planning target volume and, at the same time, with a maximum dose less than 107%. The dose prescribed to the whole breast was of 50 Gy with 2 Gy daily fractions in five weeks. A boost of 10 Gy with electrons was delivered to the tumor bed in one week. When appropriate, the supraclavicular nodal sites were irradiated by a direct 6 MV field for a total dose of 50 Gy in 25 fractions.
RIST evaluation
The acute skin toxicity was registered according to the RTOG scoring system in the following groups [Citation15]:
Grade 0: No change over baseline;
Grade 1: Follicular, faint or dull erythema/epilation/dry desquamation/decreased sweating;
Grade 2: Tender or bright erythema, patchy moist desquamation/moderate edema;
Grade 3: Confluent, moist desquamation other than skin folds, pitting edema;
Grade 4: ulceration, hemorrhage, necrosis.
All patients were weekly checked for RIST by the same physicians (F.P. and R.P.) The persistence of RIST within 40 days after the end of RT treatment was also recorded.
Dosimetric analysis
For each patient, individual DICOM RT plans [computed tomography (CT) scans, doses and contoured organ structures] were converted into Matlab-readable format (MathWorks, Natick, MA, USA) using the CERR (Computational Environment for Radiotherapy Research) software [Citation16].
DSHs of the Body were considered as representative of the irradiation in thin cutaneous layers and extracted using an in-house developed library for Matlab. To calculate absolute DSH, we extracted the relative complement in the Body of its 3D erosion defined by a spherical structuring element of radius r=3 mm (i.e. the order of magnitude of mean skin thickness). On such shell, the absolute dose-volume histogram was regularly computed and then divided by r to obtain the DSH (). To calculate the relative DSH, we used the surface of patient receiving at least 5 Gy to delimit the skin area belonging to the breast region and to normalize the absolute DSH.
Figure 1. A pictorial representation of the Body dose-surface histogram extraction from the external body shell dose-volume histogram.
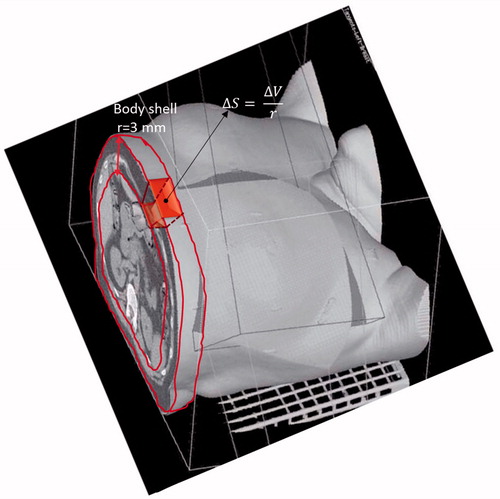
The following DSH metrics were then extracted: the absolute/relative skin surface receiving at least x dose (ASx/Sx) in step of 5 Gy; the maximum and the mean dose (Dmax and Dmean) to the skin.
Statistical analysis
Acute RIST was analyzed according to its severity, i.e. RTOG G3 vs. G0-G2. Skin dose parameters along with the patient (i.e. age, breast volume, skin disease, smoking), disease, and treatment-related (i.e. chemotherapy, hormone therapy, RT on drainage nodes) factors were included in the analysis. Univariate logistic analysis for each parameter was performed using the Spearman’s rank correlation (Rs) coefficient to assess correlation with RIST severity.
Normal tissue complication probability models
In this study, we have analyzed two NTCP models: the LKB model recast for DSHs and the multivariate logistic model. Mathematical modeling formulations are described in detail in the Supplementary Material (available online at http://www.informahealthcare.com.section).
The NTCP LKB model was fitted as previously described in [Citation17]. The maximum likelihood method was used to find the best-fit values of the LKB parameters (TD50, m and n) by maximizing the logarithm of the likelihood (LLH). TD50 is the value of the uniform dose given to the entire organ surface corresponding to the 50% probability to induce toxicity; m is inversely proportional to the slope of dose-response curve; and n accounts, in this specific case, for surface effect. The LLH function was numerically maximized using an in-house developed library for Matlab. Ninety-five percent confidence intervals for parameters estimates were obtained using the profile likelihood method. To perform an internal validation of the fitting results and to test the fit robustness, the bootstrap method was employed to determine the spread in the estimation of NTCP parameters.
The NTCP logistic regression model is based on the sigmoidal relationship between dose and response endpoint. In order to identify combinations of variables that were likely to be most predictive of RIST severity, we used automated logistic regression with bootstrap technique for variable selection, and bootstrap resampling to test selection stability [Citation18]. Briefly, only the variables most highly correlated with RIST severity (Rs > 0.2) were included in the subsequent analysis. Highly inter-correlated variables (correlation ≥0.75) were further removed keeping only the variables with the higher correlation with RIST to avoid a collinearity problem. Data analysis was performed by the DREES (Dose Response Explorer System) software [Citation19].
Model evaluation
The Rs model coefficients were analyzed to test the models’ correlation with acute RIST severity. The receiver operating characteristic (ROC) analysis was performed to evaluate the discriminating ability of model fits, and the area under the ROC curve (AUC) was calculated. The discrimination value on the ROC curve was determined by Youden’s J statistic. ROC curve comparison was performed by a Z-test. Calibration plots were also generated for graphical assessment of the agreement between observed outcomes and predictions.
Results
A total of 140 consecutive BC patients were evaluated. Their median age was 55 years (range 32–84). All patient, disease and treatment characteristics are described in . Psoriasis was diagnosed in 18 of 140 (13%) patients while atopic dermatitis in 11 patients (8%).
Table I. Clinical variables and correlation coefficient (Rs) with RIST incidence.
By the end of treatment, all patients but one developed some degree of acute RIST. Forty-four of 140 (31%) patients developed RTOG G1 toxicity, 84 (60%) G2 while G3 skin toxicity was registered in 11 (8%) patients. In almost all cases, there was an agreement in the toxicity grading evaluation by the two assessors. In only three cases, the agreement was reached after discussion. However, the disagreement regarded only G1 versus G2 toxicity. At 40 days after treatment, mild-moderate toxicity (G1-G2) was still present in six (4%) patients.
Body DSHs were generated for all patients. In , we reported the comparison between the mean cumulative percentage DSHs in patients who developed severe RIST and who did not. A significant difference (p < 0.05) between the two curves was found in the 15–45 Gy dose range as illustrated in .
Figure 2. (a) Mean cumulative patient DSHs. Red (gray, in black and white print version) lines: patients who developed severe radiation-induced skin toxicity ± SEM (Standard Error of the mean); black lines: patients who did not develop severe radiation-induced skin toxicity ± SEM. (b) Semi-logarithmic plot for the two-sample t-test between surface dose values for the two patient groups (with and without toxicity) at each dose point. The horizontal line represents the significance level of 0.05.
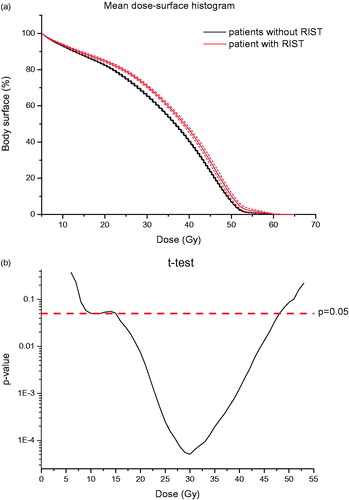
LKB NTCP model
Using DSHs, best-fit parameter estimates for LKB model of acute RIST severity were TD50=39 Gy (95% CI 37–41 Gy), m=0.14 (95% CI 0.10–0.17), and n=0.38 (95% CI 0.31–0.44). Maximum likelihood estimation values plotted in each Cartesian plane of the parameters space (TD50, m, n) are illustrated in . The 95% CI values obtained for all three parameters of LKB model showed the very good fit result. The corresponding bundle of NTCP curves are plotted in . The mean and the standard deviation (SD) of LKB model parameters obtained for bootstrap samples were TD50=39 Gy (SD = 4 Gy), m=0.13 (SD = 0.08), n=0.36 (SD = 0.05) thus showing the robustness of LKB fit procedures on body DSHs.
Figure 3. Likelihood estimation values plotted as a function of heart LKB parameters. (a) m and TD50 for a fixed value of n=0.38; (b) TD50 and n for a fixed value of m=0.14; (c) n and m for a fixed value of TD50=39 Gy; (d) NTCP bundle of curves showing 95% confidence interval region for the model fit. The red point corresponds to the optimum LLH. LKB, Lyman-Kutcher-Burman; LLH, log-likelihood; NTCP, normal tissue complication probability; TD50, uniform dose given to the entire organ volume that results in 50% complication probability.
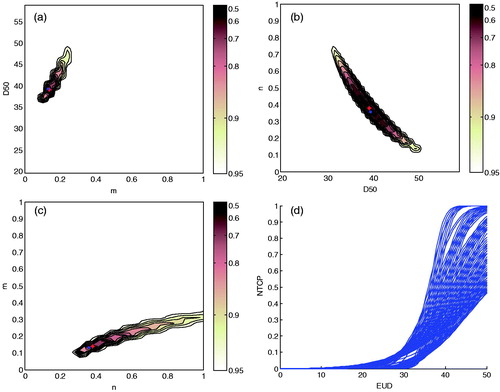
Logistic NTCP model
At the univariate analysis, psoriasis was the only clinical factor significantly associated with RIST (). Several dosimetric variables were highly correlated with RIST. A summary of dosimetric statistics is reported in Supplementary Table S1 (available online at http://www.informahealthcare.com). Dosimetric variables demonstrated strong multiple correlations. Accordingly, redundant variables (correlation ≥0.75) were not included in multivariate analysis. The bootstrap method suggested a two-variable model as the optimal one for acute RIST prediction (Supplementary Figure S1, available online at http://www.informahealthcare.com). The optimal model included S30 and psoriasis. The best-fitted regression coefficient and the odds ratios are presented in . The cut-off value for S30 was 67.8% (0.95% CI 65.1–70.6).
Table II. NTCP models: parameters estimates and 95% confidence intervals of LKB model and best-fit coefficients for logistic model with odds ratios (OR). Performance measures for LKB and logistic NTCP models.
Models’ evaluation and comparison
We obtained similar good prediction performances for both NTCP modeling approaches as shown by Rs and AUC values reported in and by ROC curves in . The logistic regression model including a dosimetric factor and psoriasis as a clinical variable has a slightly better performance. However, the difference between the AUC values has not statistical significance at the Z-test. The good performance of both LKB and logistic NTCP models can also be observed from where the comparison between the predicted incidence of RIST by each NTCP model and the actuarial incidence in the population is shown.
Discussion
BC patients treated with conserving surgery (i.e. lumpectomy or quadrantectomy) constitute a large fraction of the patients undergoing RT. Breast irradiation is generally well accepted. However, external beam RT inevitably involves skin irradiation with a consequent different dose-dependent degree of acute cutaneous toxic effects. Such acute toxicity may impact on how the treatment is psychologically tolerated and consequently on patient quality of life. In addition, acute reactions tend to progress during the treatment and can persist beyond the conclusion of it.
Ionizing radiation principally damages the mitotic ability of clonogenic or stem cells within the basal layer of epidermis, thus preventing the process of repopulation and weakening the integrity of the skin. Basal cell loss was described at radiation dose levels of 20–25 Gy, with a maximum depletion of basal cells at a dose of 50 Gy [Citation20]. Emami et al. reported a TD5/5 of 60 Gy for a 30 cm2 irradiation field and 70 Gy for a 10 cm2 irradiation field [Citation21]. However, models for prediction of acute skin toxicity are poorly researched [Citation8].
In this framework, our study aimed to find NTCP models for robust prediction of acute radiation-induced cutaneous toxicity in BC patients. Such models may help to better evaluating preventive interventions and planning oncoplastics procedures.
We prospectively evaluated 140 patients undergoing radiotherapy for BC assessing acute RIST. All patients were treated with conventionally fractionated regimens with a dose of 50 Gy to the whole breast and an electron boost of 10 Gy to the tumor bed. We found an incidence of RTOG-G3 acute skin toxicity of 8%. Using the severity of acute RIST as endpoint and individual dosimetric data from 3D-CRT plans, two different modeling approaches were considered: the LKB and the data-driven multivariate logistic NTCP models. In our modeling exercise, we analyzed the DSHs of the Body to evaluate the dose distribution to the thin skin layer. The DSHs were calculated starting from a 3D erosion algorithm that applies a spherical structuring element of radius r to extract the external shell of the Body (). Of note, the choice of radius r does not sensibly influence the resulting DSH as the dose distribution does not show large variation on sub-centimeter scales. Finally, we used the relative DSHs to fit LKB parameters or to extract dosimetric factors for logistic modeling of skin toxicity.
We estimated the best-fit values for the parameters of the traditional LKB model by the maximum likelihood and we found a tolerance dose for the breast skin that results in 50% complication probability (TD50) of 39 Gy. The estimated n value of 0.4 is consistent with a “surface” effect for the breast cutaneous tissue. When we applied a data-driven approach for NTCP modeling, the best model was a two-variable model including the percentage of breast skin receiving a dose greater than 30 Gy (S30) and a pre-existing skin disease, such as psoriasis. We found a threshold value for S30 of 68%.
Overall, most studies have reported a small volume effect for breast tissue late effects (fibrosis) [Citation11]. All those studies used breast volume for NTCP modeling. In contrast, in the present study we modeled acute RIST severity using the dose to the Body in the irradiated breast region. We found in both modeling approaches a significant effect of the amount of irradiated surface on the prediction of acute skin toxicity (surface effect). This result is in agreement with animal experiments in which the size of the radiation field was associated with the intensity of the skin reactions [Citation22].
We obtained comparable prediction performances for both NTCP modeling approaches (), with a higher Rs and only a slightly higher AUC value for the logistic model. However, according to , the logistic model seems to be more predictive as it assigns patients to high or low risk more effectively than LKB models. Interestingly, the logistic NTCP model show also a very good performance when applied to predict the persistence of skin toxicity at 40 days after treatment (Rs = 0.30 and AUC = 0.892), although, given the low number of events, this preliminary finding needs to be explored.
In the present multivariate analysis, clinically diagnosed psoriasis was found as the only clinical factor predictive for acute RIST with an odds ratio equal to 15. At our best knowledge, there are no data about the incidence of psoriasis among BC patients and its influence on the acute toxicity of RT. Psoriasis is a multi-factorial autoimmune disease with many complex immunogenetic contributions. In genome-wide association studies, predisposing genetic risk factors in the form of single nucleotide polymorphism were identified. Several of the genetic predispositions were found to potentially alter immune cell or keratinocyte behavior, but also the signaling cascades of several cytokines, such as IL-6 and IL-8 [Citation23]. However, these cytokines are known to have a role in the regulation of response of skin cells to ionizing radiation [Citation8]. As a consequence, it could be hypothesized that a genetic variant of psoriasis may influence cancer patient’s skin reaction to radiation. During recent years, increasing efforts have been taken to establish possible associations between genetic variants and BC patients’ susceptibility to develop radiation-induced side effects, such as fibrosis and skin toxicities. A genetic polymorphism of the BER gene XRCC1 has been recently associated with lower risk of skin toxicity in BC patients [Citation24]. Along with treatment and patient parameters, identification of genetic profiles of radiosensitivity in BC patients may be used to effectively individualize the management of patients at higher risk for skin toxicity and improve risk prediction capability [Citation25].
In our analyzed cohort of BC patients, we found an incidence of psoriasis of 13% which is higher than that described in the general population (1–5%) [Citation23]. However, this incidence may be influenced by the fact that the studied patients were purposely “screened” for skin diseases. Of note, only 5% of patients were known to be affected by psoriasis in a previous diagnosis. Data about the incidence of psoriasis in a population screened for skin diseases are missing and a comparison is unfortunately not possible.
It must be pointed out that one of the limitations of the present study could be the limited accuracy in calculating surface (skin) doses in tangential-like irradiation by commercial treatment planning system. As a consequence, NTCP estimates have to be considered with an unavoidable uncertainty. Further studies performed to include supplementary in vivo skin dosimetry are warranted. Under this caveat, we have performed a NTCP modeling study with the aim to provide an explanatory tool to predict severe acute skin toxicity intended for treatment plan optimization with the commonly available dose calculation algorithms.
In conclusion, we found that the calculation of DSHs represents an extremely valuable tool to take into account the dose received by the skin. Robust NTCP models for skin toxicity can be derived using the body DSHs of the irradiated area. A good prediction performance for acute RIST was obtained using a data-driven multivariate model including breast skin S30 and psoriasis.
Declaration of interest
The authors report no conflicts of interest. The authors alone are responsible for the content and writing of the paper.
References
- Lopez E, Nunez MI, Guerrero MR, del Moral R, de Dios Luna J, del Mar Rodriguez M, et al. Breast cancer acute radiotherapy morbidity evaluated by different scoring systems. Breast Can Res Treat 2002;73:127–34.
- Hymes SR, Strom EA, Fife C. Radiation dermatitis: clinical presentation, pathophysiology, and treatment 2006. J Am Acad Dermatol 2006;54:28–46.
- Leonardi MC, Morra A, Santoro L, Balduzzi A, Ivaldi GB, Vischioni B, et al. Nonrandomized comparison between concomitant and sequential chemoradiotherapy with anthracyclines in breast cancer. Tumori 2015;101:64–71.
- Kraus-Tiefenbacher U, Sfintizky A, Welzel G, Simeonova A, Sperk E, Siebenlist K, et al. Factors of influence on acute skin toxicity of breast cancer patients treated with standard three-dimensional conformal radiotherapy (3D-CRT) after breast conserving surgery (BCS). Radiat Oncol. 2012;7:217.
- Yamazaki H, Yoshida K, Nishimura T, Kobayashi K, Tsubokura T, Kodani N, et al. Association between skin phototype and radiation dermatitis in patients with breast cancer treated with breast-conserving therapy: suntan reaction could be a good predictor for radiation pigmentation. J Radiat Res. 2011;52:496–501.
- Bentzen SM, Overgaard M. Relationship between early and late normal-tissue injury after postmastectomy radiotherapy. Radiother Oncol 1991;20:159–65.
- Dorr W, Hendry JH. Consequential late effects in normal tissues. Radiother Oncol. 2001;61:223–31.
- Rubin P, Milano MT, Constine LS. ALERT - Adverse Late Effects of Cancer Treatment: Volume 2: Normal Tissue Specific Sites and System. Luther W, Brady H-PH, Michael Molls, Carsten Nieder, editor 2013.
- Alexander MA, Brooks WA, Blake SW. Normal tissue complication probability modelling of tissue fibrosis following breast radiotherapy. Phys Med Biol. 2007;52:1831–43.
- Avanzo M, Stancanello J, Trovo M, Jena R, Roncadin M, Trovo MG, et al. Complication probability model for subcutaneous fibrosis based on published data of partial and whole breast irradiation. Phys Med. 2012;28:296–306.
- Mukesh MB, Harris E, Collette S, Coles CE, Bartelink H, Wilkinson J, et al. Normal tissue complication probability (NTCP) parameters for breast fibrosis: pooled results from two randomised trials. Radiother Oncol 2013;108:293–8.
- Fitzpatrick TB. The validity and practicality of sun-reactive skin types I through VI. Arch Dermatol 1988;124:869–71.
- Kupetsky EA, Keller M. Psoriasis vulgaris: an evidence-based guide for primary care. J Am Board Fam Med. 2013;26:787–801.
- Pacelli R, Conson M, Cella L, Liuzzi R, Troncone G, Iorio V, et al. Radiation therapy following surgery for localized breast cancer: outcome prediction by classical prognostic factors and approximated genetic subtypes. J Radiat Res. 2013;54:292–8.
- Cox JD, Stetz J, Pajak TF. Toxicity criteria of the Radiation Therapy Oncology Group (RTOG) and the European Organization for Research and Treatment of Cancer (EORTC). Int J Radiat Oncol Biol Phys 1995;31:1341–6.
- Deasy JO, Blanco AI, Clark VH. CERR: a computational environment for radiotherapy research. Med Phys 2003;30:979–85.
- Cella L, Palma G, Deasy JO, Oh JH, Liuzzi R, D'Avino V, et al. Complication probability models for radiation-induced heart valvular dysfunction: do heart-lung interactions play a role? PLoS One. 2014;9:e111753.
- Cella L, Liuzzi R, Conson M, D'Avino V, Salvatore M, Pacelli R. Development of multivariate NTCP models for radiation-induced hypothyroidism: a comparative analysis. Radiat Oncol. 2012;7:224.
- El Naqa I, Suneja G, Lindsay PE, Hope AJ, Alaly JR, Vicic M, et al. Dose response explorer: an integrated open-source tool for exploring and modelling radiotherapy dose-volume outcome relationships. Phys Med Biol 2006;51:5719–35.
- Archambeau JO, Pezner R, Wasserman T. Pathophysiology of irradiated skin and breast. Int J Radiat Oncol Biol Phys 1995;31:1171–85.
- Emami B, Lyman J, Brown A, Coia L, Goitein M, Munzenrider JE, et al. Tolerance of normal tissue to therapeutic irradiation. Int J Radiat Oncol Biol Phys 1991;21:109–22.
- Hopewell JW. Mechanisms of the action of radiation on skin and underlying tissues. Br J Radiol Suppl. 1986;19:39–47.
- Perera GK, Di Meglio P, Nestle FO. Psoriasis. Ann Rev Pathol. 2012;7:385–422.
- Seibold P, Behrens S, Schmezer P, Helmbold I, Barnett G, Coles C, et al. XRCC1 polymorphism associated with late toxicity after radiation therapy in breast cancer patients. Int J Radiat Oncol Biol Phys 2015;92:1084–92.
- Andreassen CN. Can risk of radiotherapy-induced normal tissue complications be predicted from genetic profiles? Acta Oncol. 2005;44:801–15.