Abstract
At Arctic and Antarctic latitudes, personnel are deprived of natural sunlight in winter and have continuous daylight in summer: light of sufficient intensity and suitable spectral composition is the main factor that maintains the 24-h period of human circadian rhythms. Thus, the status of the circadian system is of interest. Moreover, the relatively controlled artificial light conditions in winter are conducive to experimentation with different types of light treatment. The hormone melatonin and/or its metabolite 6-sulfatoxymelatonin (aMT6s) provide probably the best index of circadian (and seasonal) timing. A frequent observation has been a delay of the circadian system in winter. A skeleton photoperiod (2 × 1-h, bright white light, morning and evening) can restore summer timing. A single 1-h pulse of light in the morning may be sufficient. A few people desynchronize from the 24-h day (free-run) and show their intrinsic circadian period, usually >24 h. With regard to general health in polar regions, intermittent reports describe abnormalities in various physiological processes from the point of view of daily and seasonal rhythms, but positive health outcomes are also published. True winter depression (SAD) appears to be rare, although subsyndromal SAD is reported. Probably of most concern are the numerous reports of sleep problems. These have prompted investigations of the underlying mechanisms and treatment interventions. A delay of the circadian system with “normal” working hours implies sleep is attempted at a suboptimal phase. Decrements in sleep efficiency, latency, duration, and quality are also seen in winter. Increasing the intensity of ambient light exposure throughout the day advanced circadian phase and was associated with benefits for sleep: blue-enriched light was slightly more effective than standard white light. Effects on performance remain to be fully investigated. At 75°S, base personnel adapt the circadian system to night work within a week, in contrast to temperate zones where complete adaptation rarely occurs. A similar situation occurs on high-latitude North Sea oil installations, especially when working 18:00–06:00 h. Lack of conflicting light exposure (and “social obligations”) is the probable explanation. Many have problems returning to day work, showing circadian desynchrony. Timed light treatment again has helped to restore normal phase/sleep in a small number of people. Postprandial response to meals is compromised during periods of desynchrony with evidence of insulin resistance and elevated triglycerides, risk factors for heart disease. Only small numbers of subjects have been studied intensively in polar regions; however, these observations suggest that suboptimal light conditions are deleterious to health. They apply equally to people living in temperate zones with insufficient light exposure. (Author correspondence: [email protected])
INTRODUCTION
Living in polar regions entails an ability to adapt to extremes of cold, daylength, and in some circumstances isolation for long periods of time. Transport possibilities are often greatly restricted or impossible in winter due to weather conditions and/or distance. In many situations during the polar winter, temporary residents are few in number, restricted in movement, and at present external contacts depend on satellite communications. Thus, the psychology of over-wintering personnel has been the object of much investigation. The physiology of temporary polar residents is also of interest and concern. Initially, adaptation to the cold environment was a primary study objective. Later, it became apparent the lack of natural sunlight in winter was probably of greater importance. This perception was enhanced when seasonal affective disorder (SAD) was described in 1984, attributed to the long nights of winter, and successfully treated, initially, with extra bright light mimicking a long summer day (Rosenthal et al., Citation1984). Existing evidence suggests that the circadian system is disturbed during the polar winter due largely to insufficient bright light.
This review will consider current knowledge of human circadian, and to some extent seasonal function in polar regions. It will suggest areas that require further investigation and approaches to treatment of circadian problems. It will emphasize data from the Antarctic, since more in-depth circadian data appear to be available than for the Arctic, and the author has long-term personal experience of research on these problems in Antarctica with the British Antarctic Survey. It will not consider the situation of native Arctic residents, as temporary civilian and military residents constitute a very different population. For example, early work by Lobban (Citation1967) described marked differences between the circadian electrolyte rhythms of native Arctic residents and temporary personnel. Some important data have been obtained from Arctic Norway (Svalbard, 74–81°N) (e.g., Lewis & Lobban, Citation1957). Large numbers of personnel work in the Arctic and sub-Arctic industrial areas of Russia. They are likely to have similar circadian problems to North Sea oil rig workers, who will also be discussed here.
Literature was searched by Scopus, PubMed, MEDLINE, and ISI, using the search terms below and with no limits on the year of publication:
Circadian and human and (Arctic or Antarctic)
Circadian and human and light (Arctic or Antarctic)
Circadian and human and melatonin (Arctic or Antarctic)
Sleep and human and (Arctic or Antarctic)
Hormones and human and (Arctic or Antarctic)
Cardiovascular and human and (Arctic or Antarctic)
Cold exposure and circadian and human and (Arctic or Antarctic)
In total, 250 appropriate papers were initially retrieved. The author's personal collection of papers, together with a selection from those revealed by the search, forms the basis of this review. The selection was based on the degree of relevance to circadian function and existing evidence for the importance of seasonal effects in humans, for example, increased mortality from cardiovascular disease in the winter (Leppaluoto & Hassi, Citation1991). A few publications requiring translation from languages other than English are not covered.
GENERAL DESCRIPTION OF CONDITIONS
Personnel
Civilian and military personnel are subject to careful selection procedures. Some countries employ psychological profiling for selection purposes as well as the requirement to be medically and physically fit. Both women and men are given the opportunity to carry out polar duties, although until fairly recently (ca. 1995) only men were present on British bases in Antarctica, and men are still the most numerous over-wintering staff. Personnel in Antarctica consist largely of scientists and support staff. Personnel deployed to Halley Bay, 75°S (a British Antarctic Survey base), are required to accept the possibility that in case of illness it may not be possible to evacuate them. Again on British bases, a full-time base doctor is employed, who is also trained in dental procedures. The principal medical problem, in terms of mortality and morbidity, is trauma. In incidence, trauma (usually less serious), skin problems, minor infections, and mental health (also usually minor) are all close to being on a par, with trauma still probably in the lead (personal communication, Dr. I. Grant, Head, British Antarctic Survey Medical Unit). The base doctor carries out research projects as well as participates in general maintenance and other duties. On bases with year round access, Arctic and Antarctic, the situation is somewhat different, but the light conditions remain the same at similar latitudes, north or south.
Light-Dark Cycle
Above the Polar Circle latitudes of 66.33′, north or south, the sun is above the horizon for 24 h for at least 1 d/yr (midsummer day, midnight sun) and concomitantly below the horizon for 24 h for at least 1 d/yr (midwinter day). With increasing latitude, there will be more days with either no sunlight or continuous sunlight. For example, at Halley Bay, 75°S, the sun does not rise for 100 d in the winter and does not set for 110 d in summer. shows the actual personal light exposure recorded by ActiwatchL (AWL; Cambridge Neurotechnology, Cambridge, UK) at Halley. During the winter with only artificial light, and an orange glow on the horizon at noon, the maximum light exposure possible is ≈500–700 lux (Broadway et al., Citation1987b; Francis et al., Citation2008), whereas on a bright sunny day in summer in polar regions, the outdoor light intensity can exceed 40 000 lux (and in temperate/equatorial regions can exceed 100 000 lux). Measured personal exposure is somewhat less on average (). Thus, a strong light-dark zeitgeber for circadian entrainment is absent during the winter period when the sun is below the horizon.
FIGURE 1. Personal light exposure measured at 2-min intervals by ActiwatchL at Halley base, 75°S. Average number of subjects per time point = 7 ± 1 (SD). Data collected by Dr. Thomas Rieley, base doctor (Halley, Rieley, & Arendt, unpublished). Note low values for December 2001, due to no relief ship, and, thus, less outdoor work.
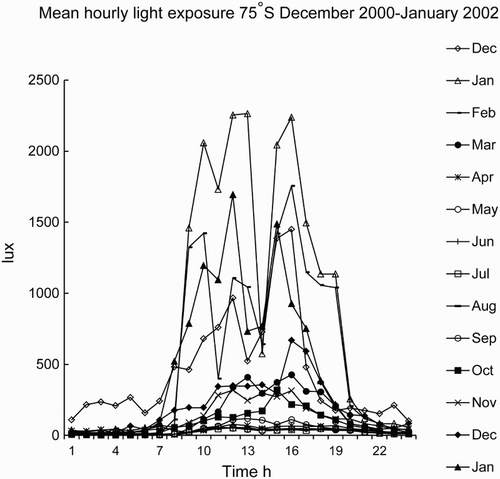
It is obviously possible to generate a light-dark cycle in summer in these conditions by using window blinds at the appropriate times, keeping a normal routine for bedtime/sleep, and wearing eye masks at night if necessary. Nonetheless, personnel working during the night shift can (unusually) experience bright light at night. The exposure to ultraviolet (UV) light is so strong that UK base personnel are required to wear sunglasses whenever outdoors in summer. To date, there is little information on what light intensity is actually experienced in polar regions wearing sunglasses outdoors. In winter, however, the light-dark cycle is dependent on artificial light. This will obviously depend on the equipment on a given base. Anecdotally, on some bases the lights may be dimmed deliberately in winter to conserve fuel.
Temperature
To date, the lowest recorded temperature on earth was −89.2°C, July 21, 1983, at Vostok, the Russian Antarctic Base (http://www.antarcticconnection. com/antarctic/weather/index.shtml). The lowest environmental temperature recorded in the Arctic was −68°C, (Verkhoyansk, Siberia; source: Wikipedia). The Arctic is generally warmer than the Antarctic due to ocean influences. The temperature recorded at 75°S can descend to less than −50°C. There are few publications concerning the circadian aspects of body temperature in polar conditions. Evidently, protective clothing and mostly indoor work in winter will reduce exposure to extreme cold.
Food
Food is of great importance in the circumstances under consideration. Evidently, it will vary with different installations, but, in general, it punctuates the day, providing a social framework, together with scheduled activity and sleep. From the point of view of morale, the cook is probably the most important person on the base, although there is no objective evidence to support this opinion. From a scientific point of view, the fact that on small bases personnel eat essentially the same food and in the same environment for long periods of time means that rather controlled clinical research can be carried out long term. On bases with year round access, the variety of food will be much greater than on those isolated during the winter.
Scheduled Activity
A structured day with set periods of eating, working, and sleeping is mandatory on British bases in Antarctica. A normal working day is 09:00–17:00 h, with breakfast, lunch, and dinner at approximately 08:00, 13:00, and 18:00 h. It is enforced, as far as possible, by a command structure and is of great importance, as will be evidenced later. Evidently, circumstances, such as meteorological observations, can change this timing for the individ-uals concerned.
Clock Time
All 24 time zones converge at the poles, and clock time may be manipulated for convenience. Halley base is on Greenwich Mean Time (GMT) during the period from February/March to November/December to facilitate communications with the UK, and on GMT − 3 for the rest of the year to facilitate communication with the larger Base of Rothera. The actual date of the change is somewhat variable, depending on air operations. So far, no research has been carried out specifically to investigate the physiological or psychological response to this large abrupt change in time cues during a period of rapid change in the environmental light-dark cycle. Other locations use various clock times; for example, McMurdo uses New Zealand Time (including Daylight Saving Time), and the South Pole is on GMT. A guide to time zones on different Antarctic Bases can be found at www.timegenie.com/country.time/aq. It is very important to bear any possible artificial changes in mind when conducting or evaluating medical research in polar regions.
This prolonged introduction to the polar situation is designed to underpin the following observations on human circadian rhythms, since all these factors have an influence on human biological timing.
POLAR STUDIES
The literature concerning human polar rhythm physiology is not vast, and there is little recent accessible data concerning circadian rhythms in the Arctic. In Antarctica, it is restricted by the number of temporary residents over-wintering who are available to participate in studies.
Importance of Light Intensity
From the beginning of polar exploration, anecdotes recall the enormous impact of the return of the sun in spring, thus underlining the problems of lack of daylight (e.g., http://www.royalcollection.org.uk/microsites/HOT GA/McroObject.asp?item=16&themeid=1190&object=25 80070&row=16&detail=about). Many early (and some more recent) studies did not report the artificial light levels in the polar winter. However, most lighting in internal workspaces generates an illumination that is rarely greater than 300–500 lux, wheras with the sun below the horizon (as at night or during a prolonged polar winter), the external illumination will not exceed 100 lux and is usually below 1 lux (e.g., Schylter, Citation2008). As noted previously, illumination with the sun directly overhead is around 100 000 lux, and on a heavily overcast day it will still be ∼1000 lux. In the Antarctic, there is some evidence for an increase in light sensitivity during the winter (Owen & Arendt, Citation1992); however, in a controlled environment with very weak nonphotic zeitgebers, between 200 and 1000 lux white light (measured in the angle of gaze, full 12-h photoperiod) is required to maintain circadian phase (Middleton et al., Citation2002).
Following the pioneering work of Lobban (Citation1967), a number of studies in the early 1980s described abnormalities of circadian rhythms during the Antarctic winter, with evidence for internal desynchrony of some cardiovascular variables due to late phase of some, notably the rate of rise of intraventricular pressure (D'Yachkov & Moshkin, 1981; Moshkin, Citation1984). A substantial delay in the core body temperature rhythm was seen during the polar winter, and the author linked changes in temperature, electrolytes, and keto-steroids to changes in luminosity (Moshkin, Citation1984). In the same study, evidence was found for two peaks in the rhythms of 17-ketosteroids and 17-ketogenic steroids, possibly suggesting a conflict between endogenous and exogenous factors influencing circadian rhythms.
Melatonin as a Rhythm Marker
The ability to measure the pineal hormone melatonin and its metabolite 6-sulfatoxymelatonin (aMT6s) as marker rhythms for circadian phase has provided further evidence of circadian changes in high latitudes. There is evidence that melatonin signals daylength to human physiology, as it does in other species (Arendt, Citation1999; Buresova et al., Citation1992; Wehr et al., Citation2001). Thus, a change in duration of its secretion might be expected in conditions of extreme daylength. If subjects are kept for 2 mo in artificial short days (10L:14D) and then for 2 mo in artificial long days (16L:8D) with complete darkness during the imposed night, there is a clear change in the duration of melatonin secretion (Wehr, Citation1991). In high-latitude natural environments, the observations are not so conclusive. There are reported seasonal variations in human melatonin (and aMT6s), with an earlier phase in summer (Bojkowski & Arendt, Citation1988; Broadway et al., Citation1987b), and, according to some reports, increased levels and duration of secretion in winter (Kauppila et al., Citation1987; Makkison & Arendt, Citation1991; Paakkonen et al., Citation2008). However, the nature and timing of daily activities and sleep will evidently influence exposure to ambient light conditions, whether natural or artificial. There is more evidence for seasonal changes in the circadian phase of melatonin than for changes in the duration of its secretion.
Phase Delay and Free-Running
Probably the best study to illustrate the effects of an unstructured life during the Antarctic winter is that of four Greenpeace volunteers over-wintering at Cape Evans Base 77°38′S in 1990 (Kennaway & Van Dorp, Citation1991). For the entire period of sundown (sun below the horizon for 126 d), all four (three men and one woman) showed free-running rhythms of all the variables studied: sleep, aMT6s, electrolytes, and cortisol. Each person free-ran with their own period initially, although two (a man and a woman) subsequently free-ran with the same period. With the return of the sun, all four resynchronized to the 24-h day. The lighting environment was not particularly dim; the hydroponics equipment reportedly produced 1000 lux. However, this base had no specific activity except to keep a radio watch. At Halley (75°S), without interventions, free-running does occur in winter, particularly after a week of night-shift work, but it is rare, one or sometimes two subjects in a complement of 15–16 over-winterers. However, delay of sleep timing and the melatonin rhythm is common in winter (see for a synchronized subject with major delays of sleep timing on weekends). The lit environment did not exceed 500 lux during these observations (); thus, the retained 24-h period is difficult to explain on the basis of light. Rather, in the author's opinion, it is the structured day that is imposed, with specific times for meals, work, leisure, and sleep, that maintained a 24-h period, albeit with a large phase delay on weekends. In these circumstances, all available time cues act together to maintain synchrony.
FIGURE 2. Sleep (black bars) at Halley base (75°S) of a representative subject showing synchronization of sleep (diaries): artificial wake-up for most week days with scheduled work and rest, but substantial phase delay on weekends in winter—May to September (O'Conor, Citation1989; redrawn from Arendt, Citation1995). Note three periods of night shift during the year.
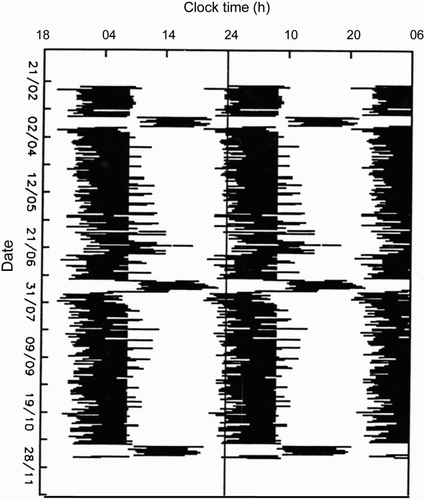
Sleep
In spite of a structured day on this polar base, and in “normal” working polar environments, a frequently reported problem with the winter is sleep. Early concerns were discussed in 1957 (Lewis & Masterton, Citation1957). Polysomnography was first used in the late 1960s and early 1970s in limited and small studies (Natani et al., Citation1970; Paterson, Citation1975; Popkin et al., Citation1974) and suggested a loss of Stage 4 sleep in the winter. One study with eight individuals concluded that sleep patterns between individuals were so different that no statistical conclusions could be drawn (Buguet et al., Citation1987). Recently, a detailed polysomnographic study of eight individuals during a year at Maitri research station (70°S) showed reduced sleep efficiency, total sleep time, and slow-wave sleep (Stages 3 and 4) during the winter months, consistent with the findings of the earlier smaller studies (Bhattacharyya et al., Citation2008). A questionnaire study (2822 participants) has described the dependence of sleep length (and chronotype, i.e., diurnal preference) on a number of factors in Arctic Russia. Notably, sleep was shorter and chronotype later during equinoctal periods compared to long days. The time of sunrise during lengthening days appeared to be a stronger predictor of sleep length and chronotype than daylength, itself (Borisenkov, Citation2011).
“Midwinter insomnia” has repeatedly been described for regions of northern Europe, particularly Norway, and is characterized by difficulties falling asleep. A large survey was conducted in Tromso, a Norwegian city north of the Arctic Circle. Of the 14 667 male and female respondents, 17.6% of women and 9.0% of men reported insomnia during the dark period of the year. Insomnia in the midnight-sun period, or in spring or autumn, was much less common. Late sleep onset was the most common type of insomnia, both in winter and summer. Overall, the frequency of insomnia increased with increasing age (Husby & Lingjaerde, Citation1990). A more recent fairly large study compared 453 Norwegians (319 males and 134 females) and 450 Russians (317 males and 133 females), all aged 18 yrs or older, living on Svalbard. The Russians, but not the Norwegians, reported more problems during the polar night. Of the Russians, 81% of the men and 77% of the women reported sleep problems (difficulty falling asleep, night awakenings, not feeling rested) lasting for at least 2 wks, nearly 4-fold higher than the Norwegians. The authors considered that this may have been due to the greater recruitment of Russians from lower latitudes compared to the Norwegians (Nilssen et al., Citation1997).
Hypnotic drugs were used quite successfully in the 1980s to counter this problem (Lingjaerde & Bratlid, Citation1981; Lingjaerde et al., Citation1983). However, it is undesirable to use pharmacological interventions if other approaches can be used. The difficulty falling asleep was hypothesized to be due to delayed-sleep phase in the low light levels of the Arctic winter. Interventions designed to advance circadian phase using treatment with bright light in the early morning (30 min, 07:30–08:30 h) were also successful in advancing sleep onset and reducing morning drowsiness in nine subjects. Support for the delayed-phase hypothesis was given by a probable advance in the melatonin rhythm (evening rise) after 5 d of light treatment (Hansen et al., Citation1987; Lingjaerde et al., Citation1985). Simultaneously, at the other end of the earth, a clear delayed phase of the entire 24-h profile of the melatonin rhythm was shown in the Antarctic winter on an underground base with controlled activity and rest. Treatment with a “skeleton photoperiod” of 1-h bright light (2000 lux broad-spectrum white light) in the morning (08:00–09:00 h) and in the evening (19.30–20.30 h) restored the earlier summer phase position (Broadway et al., Citation1987b); however, in this study, sleep was not assessed.
Further support for the delayed-phase hypothesis in winter is provided by the findings of other studies, such as a 4-h delay in the melatonin rhythm and a 2-h delay in core temperature during the winter compared to summer in nine men at Dome Fuji (77°S, 39°E). Illumination was measured at the beginning of the study in the living spaces (<300 lux) and in the living room (<500 lux). In this case, there was no delay in sleep and activity, and the authors concluded that the latter were reset by scheduled patterns of behavior, whereas the more endogenous rhythms responded to photoperiod/light intensity (Yoneyama et al., Citation1999). This situation is evidently one of internal desynchronization between sleep and more endogenous circadian rhythms. A trend towards delayed sleep was evident in a small, but thorough, study at 70.37°S (Wortmann et al., Citation1999). Usui et al (Citation2000) found evidence of desynchronization and phase-delayed sleep in the Antarctic midwinter in eight men of the Japanese Antarctic Research Expedition. Similarly, delayed sleep was reported in midwinter at 69°N by Bratlid and Wahlund (Citation2003).
However, the winter dim-light hypothesis for delayed sleep is confused by several reports of delayed sleep/free-running in summer. Free-running rhythms in body temperature, activity/rest time, electrolytes of urine samples, grip strength, and mood have been reported from Svalbard in the summer (Johnsson et al., Citation1979, Citation1983; Lobban, Citation1967). Steel et al. (Citation1995) described free-running rhythms of sleep in five of the six subjects (men) in a high Arctic research camp in natural conditions of constant daylight. Stokkan and Reiter (Citation1994) reported delayed melatonin phase in June at 70°N. Similarly, in a very small study of three people, Gander et al. (Citation1991) found a 2-h delay in the rhythms of temperature, activity, and heart rate in the Antarctic summer compared with New Zealand, without a delay in sleep; thus, internal desynchronization was present in summer (cf. Yoneyama et al., Citation1999). But, one report considered that although decrements in sleep could be observed, sleep appeared to be improved by prolonged Antarctic residence (Palinkas et al., Citation2000).
It would be reasonable to postulate that delayed phase in winter is due to the lack of a strong light zeitgeber in the morning, and that delayed phase in summer is probably due to exposure to bright light on the delayed part of the light phase response curve, if daylight is not artificially obscured in the evening/night, and if work and sleep periods are not scheduled. Such a situation may be found in field camps. It is also a great temptation in 24-h days to go skiing in the middle of the “night.” The type of built environment (under- or over-ground habitation, for example) will have substantial influence on exposure to natural light in the summer. Sampling conditions on polar bases in summer are more difficult to control than in winter; personnel are very busy, as most outdoor work is carried out during the 24 h of daylight, and, thus, the exposure to bright light is unpredictable and measurements are required. Even then, the use of sunglasses compromises the measurement of light exposure.
Other factors affect sleep, for example, exercise. During the polar winter, less exercise (physical labor) is taken outdoors, and possibly indoors, although the author is not aware of published objective assessments of exercise in winter and summer in polar regions. Activity recordings on Halley show a decline of movement, in general, during the winter (author's own data, unpublished), presumably because most outside physical work takes place in summer. Sleep was improved and the onset of melatonin secretion was earlier in two reports by Weydahl (Citation1991, Citation1994) after increased exercise during the Arctic autumn, and this observation may be pertinent. A gymnastic facility is provided on some Antarctic bases, but it is entirely up to the individual on British bases whether or not it is used.
Light Intervention to Manipulate Sleep and Circadian Phase
At Halley, 75°S, following a polysomnographic evaluation of sleep (Paterson, Citation1975) and treatment of delayed phase in winter by a skeleton bright light photoperiod (Broadway et al., Citation1987a) mentioned previously, we have carried out a series of sleep studies with and without intervention of bright light. Preliminary observations using sleep logs provided evidence of substantial winter delay, especially weekends when the requirement to get up and work is absent ( Arendt, Citation1995; O'Conor, Citation1989) (). During this year, one subject free-ran throughout the entire period of measurement. Anecdotally, this was behavioral and possibly an attempt to live an independent life in the confined environment.
With the availability of personal activity/sleep recording (actigraphy) enabling simultaneous light measurement (e.g., ActiwatchL), it has become possible to objectively assess sleep, circadian rhythms, and behavior long term and as a function of the lighting environment. Moreover, short-wavelength light (blue, ca. 460–480 nm) is now known to be the most powerful part of the spectrum with regard to phase-shifting the circadian system (Lockley et al., Citation2003; Revell et al., Citation2005). The availability of blue-enriched lamps (Philips Lighting, Eindhoven, The Netherlands) has enabled comparisons of standard white light with blue-enriched light in a polar environment with minimal natural light.
Using objective measures (see above), at 75°S sleep was less efficient, sleep onset was delayed, and sleep quality was worse in midwinter compared to control periods of 3 wks just after the autumn equinox and just before the spring equinox, even with an intervention of extra bright light (Philips Lighting) throughout the midwinter period from 08:00 to 18:00 h (2003: 10 subjects, one woman; 2006: 15 subjects, five women) (Francis et al., Citation2008; Mottram et al., Citation2010). In 2003, we compared alternating 4–5-wk periods of full-spectrum white light (4000K) with prototype blue-enriched light (10 000K) from ≈08:00 to 18:00 h with regards to sleep and circadian phase. There was slightly better sleep efficiency with blue-enriched light. The maximum exposure (average of all subjects) during the midwinter for both white and blue-enriched light was ca. 1000 lux (no difference between white and blue) compared to 570 lux with no intervention. In 2006, Activiva Active blue-enriched light was available (Philips Lighting), and the experiment was repeated. In this case, average maximum light intensity reached 1997 lux and 1840 lux (blue-enriched and white lights, respectively). Again, sleep (efficiency, timing, duration, and quality) was worse in winter than during control periods with natural light, but the light intervention greatly reduced the sleep delay in midwinter compared to 2003, and the blue-enriched light was significantly better than the white light with respect to the timing, latency, and actual length of sleep (Mottram et al., Citation2010). Moreover, a significant advance (∼1 h) of circadian phase was shown in the blue-light treatment periods, consistent with the effect on sleep timing (all the light equipment was kindly provided by Philips Lighting) (). Very recently, a preliminary conference report of similar work at the Concordia base (Dome C, 75°S) described an advance of aMT6s phase with blue-enriched light (17 000K) during the polar winter (Gronfier et al., Citation2010).
FIGURE 3. (a) Sleep timing in alternating 4–5-wk periods of standard white and blue-enriched lights at 75°S, 2006, with 3-wk controls (no extra light), before and after extra light, beginning in March and ending in October. The sun is below the horizon from May 2 to August 10. There were no significant differences in wake-up time between light conditions. Sleep start time was 19 min earlier in blue-enriched light than standard white light (p < .05). (Redrawn from data described in Mottram et al., Citation2010.) (b) Circadian phase assessed with the aMT6s rhythm in urine during the mid-winter periods B1, B2 (blue-enriched light, 1) and W2, W3 (standard white light, 2) as in part a. During sundown, the aMT6s acrophase was earlier in B1 and B2 than in W2 and W3 (paired t test of average individual values p < .03, n = 8). (Unpublished diagram from data described in Mottram et al., Citation2010.)
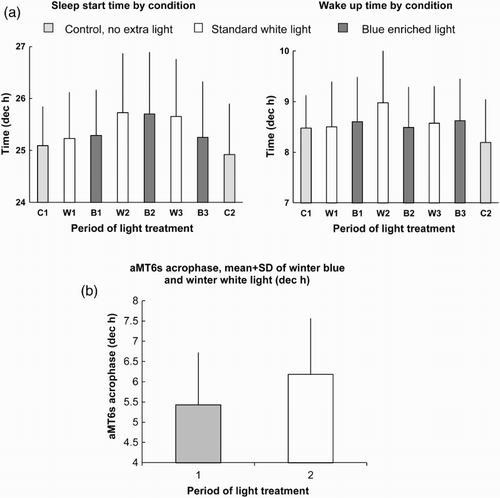
The question then arises as to whether or not it is necessary to increase the winter artificial light intensity for the full day in order to maintain circadian entrainment. The question is pertinent, since increasing the light environment demands extra energy and fuel, a critical commodity in regions where transport and fuel availability are not simple to arrange and cost more than in more accessible environments. The existing published evidence suggests that a short daily pulse of broad-spectrum white light (30 min to 1 h) of sufficient intensity (10 000 or 2000 lux, respectively) should maintain a reasonable phase position of the melatonin circadian rhythm. The evidence derives primarily from studies on SAD, for which morning-light treatment is the most efficient at reducing symptoms and advancing the melatonin rhythm (Takasu et al., Citation2006; Wirz-Justice et al., Citation2009). Whether or not this suffices to maintain sleep phase, quality, and alertness in polar regions remains to be seen, although sparse Arctic studies would argue in its favor (Hansen et al., Citation1987; Lingjaerde et al., Citation1985). Moreover, alertness and performance may not be optimized during the whole day if dim light succeeds the bright light pulse. Recent work from Halley (Corbett, Citation2011) does suggest that an improvement in alertness and performance is possible with such a light regime.
It is possible to devise an algorithm for the lighting environment, whereby light is increased on waking in the morning (to set phase and increase alertness), intensity is reduced through the morning, then increased after lunch (to combat postprandial sleepiness), then decreased during the afternoon until the end of the “day” (Philips Lighting, personal communication). Further dimming of the lights as the evening progresses should enable early onset of melatonin secretion and increase of sleep propensity. In this way fuel consumption could be minimized.
Mood
It is not the remit of this review to consider mood and/or the psychology of polar residence, the reader is referred to a recent review (Palinkas & Suedfeld, Citation2008). However, SAD, in particular, (but also some other psychiatric disorders) is closely associated with circadian rhythms.
The first theory as to the cause of SAD was based on animal photoperiodicity. The duration of melatonin secretion signals long (short-duration signal) or short (long-duration signal) days to organize the timing of seasonal rhythms (Arendt, Citation1986; Carter & Goldman, Citation1983; Rosenthal et al., Citation1984). The duration of melatonin secretion is dictated by the perceived photoperiod, and it was known by 1980 that light of sufficient intensity suppressed melatonin at night (Lewy et al., Citation1980; Terman et al., Citation2001). Thus, SAD patients were treated with 2500 lux white light for 3 h in the morning and 3 h in the evening to shorten the melatonin profile. The results were convincing with remission of symptoms in the majority of patients. However, it has never been proven that it is the long duration of melatonin that causes SAD. Subsequently, a number of studies have shown that morning light, alone, of sufficient intensity and duration (10 000 lux, 30 min recommended at present) was sufficient to produce clinically relevant remission, over and above a placebo effect. A simulated dawn with lower-intensity light is also effective (Terman & Jiuan Su, Citation2010; Terman et al., Citation2001; Wirz-Justice et al., Citation2009). Moreover, this was associated with a phase advance of melatonin secretion. This clearly suggested that a change in circadian phase (delay) was the root of the SAD problem. Yet, not all SAD patients are delayed in phase. Even more recently, it has been proposed that a specific phase relationship of sleep and circadian phase is the critical determinant of mood in SAD. Specifically, this was considered to be a difference of 6-h between mid-sleep and the dim light melatonin onset (DLMO; normally ca. 21:00 h) or 3 h between the core temperature minimum and wake up (Lewy et al., Citation2006). Thus, a current theory in this field is that SAD patients are particularly sensitive to disturbed internal circadian phase relationships between the more endogenous rhythms, such as melatonin, and the weakly endogenous rhythms, such as sleep.
There is certainly some evidence for subsyndromal SAD in polar regions based upon observations on reasonably large numbers of subjects of two studies (Palinkas et al., Citation1995, Citation2004)—119 men and women, and 220 men and 93 women, respectively. The incidence was greater in military than civilian personnel and in women. However, a small, but thorough, questionnaire study (Wortmann et al., Citation1999) found no evidence of low mood over the year at 70°S. Another self-reported questionnaire study using more than 50 subjects over several years on the British Antarctic bases of Rothera and Halley suggested that subjective sleep problems and tiredness, manifesting as depressive symptoms, remain a problem during winter (Harris et al., Citation2010), despite an unspecified improvement in ambient base lighting.
During supervision of research work at Halley since 1984, the author has not encountered overt SAD requiring specific treatment (information from base doctors), but, anecdotally, “mental health problems” and “low mood” are reported by some. It should be noted that women have a greater incidence of SAD than men; for example, a study of military and civilian personnel in Alaska (Fort Wainwright) found a 10.9% incidence of true SAD in active duty females when the average score using the Seasonal Pattern Assessment Questionnaire (SPAQ) was in the low range for subsyndromal SAD (Levine, Citation1995). The majority of temporary polar residents are robust healthy young men. But given the possibility of overt SAD, it is evidently important to ensure the normality of circadian phase relationships. Fortunately, the health of people who have spent time in Antarctica over-wintering appears to be generally better than the population in temperate zones (Palinkas, Citation1986, Citation1987). Interestingly, SAD appears to be rare in Iceland (Magnusson et al., Citation2000)
Performance
There are clear daily rhythms in physical performance, which appear related to the 24-h rhythm of core body temperature (e.g., Waterhouse et al., Citation2005), and in cognitive performance (e.g., Blatter & Cajochen, Citation2007) in temperate zones, and in the Arctic and Antarctic environments (Buck, Citation1980; Wortmann et al., Citation1999). Both positive and negative effects of external temperature have been described (Makinen, Citation2007). Internal desynchrony of sleep and the circadian system impairs cognitive performance (Wright et al., Citation2006), with evidence in athletes for impairment of physical performance (Reilly & Edwards, Citation2007; Reilly et al., Citation2005), although much remains to be learned in this area (Reilly & Edwards, Citation2007). As for other circadian rhythms, reestablishment of normal phase relationships, at least in controlled laboratory studies, improves function (Czeisler et al., Citation1990).
Assessment of performance rhythms in the blind provides some insight into the problem of weak zeitgebers (Lockley et al., Citation2008). A comparison of free-running, delayed phase, and entrained blind subjects demonstrated that in the entrained group, measures of alertness and performance, though initially poor, improved over the course of the day, before deteriorating again ∼6 to 9 h after waking. However, in the nonentrained group, who were either phase-delayed or free-running, the amplitude of the performance and alertness scores was decreased and failed to show the circadian variation noted in the control (entrained) group. A small group of nonentrained, but phase-advanced, individuals performed in a manner similar to the control group.
A small study has recently been completed at Halley assessing the effects of a single 1-h light pulse of high-intensity bright white light (5300K; Philips Bright Light Devices, HF3305) from 08:30 to 09:30 h daily for two periods of 2 wks on either side of the winter solstice, with two control periods before and after the light treatments. Cognitive performance, sleep (actigraphy), and circadian phase were measured (Corbett, Citation2011). There was an advance in circadian phase, with an accompanying change in sleep timing and improved objective measures of cognitive performance and alertness in response to exposure to this single 1 h of bright light. There was also a suggestion that subjective nocturnal sleep quality may be improved during morning light treatment, with an effect that persists following its removal. But, there was no evidence of improvement in subjective measures of alertness.
Metabolism and Food
A “food entrainable oscillator,” independent of the central pacemaker in the suprachiasmatic nucleus (SCN), has been demonstrated in animals. Specifically, it is the timing of available food that leads to entrainment (Fuller et al., Citation2008). Thus, a combination of timed food, scheduled sleep, and appropriate light-dark cycles is, in principle, a strong zeitgeber, which can be further strengthened by other known influences, such as exercise and possibly food content (Krauchi et al., Citation2002).
Since it is now evident that the circadian system has a profound influence on metabolism (Ruger & Scheer, Citation2009), therein may be a possible explanation for some of the gastrointestinal complaints of desynchrony (notably in shiftworkers). Early work clearly showed rhythms in the enteroinsular axis (Morgan et al., Citation2003; Van Cauter et al., Citation1989, 1997). Importantly, it is demonstrable that meals taken at an inappropriate phase with regard to circadian time (for example, in the middle of the biological night) are followed by abnormal postprandial metabolism, with evidence of insulin resistance and slow clearance of blood lipids, i.e., triglycerides (Hampton et al., Citation1996; Holmback et al., Citation2002; Ribeiro et al., Citation1998; Sopowski et al., Citation2001). These are risk factors for heart disease and are associated with obesity, metabolic syndrome, and diabetes—all considered health risks of night-shift work.
There are sparse metabolic data concerning rhythms from polar regions. Campbell et al. (Citation1975a, Citation1975b) performed oral glucose tolerance tests (12 young men) in the Antarctic, in the morning and afternoon at 3-mo intervals (March, June, September, December). The characteristic diurnal variation in glucose tolerance persisted throughout the year, but was greater in March and December. There were significant seasonal differences in glucose tolerance; blood glucose values were lowest, both morning and afternoon, in December (midsummer). When the sleep-activity pattern was reversed in one subject, the diurnal variation of glucose tolerance was also reversed. It is possible that light exposure may have rapid effects on glucose tolerance: in a single case report, treatment of SAD with light in a diabetic patient reduced the requirement for insulin (Allen et al., Citation1992), and, interestingly, there is a report of lowered insulin during the summer season in Antarctica (Malhotra et al., Citation1998). Periodicity of cholesterol synthesis did not differ during 18 d in 24-h Arctic daylight, and the authors concluded that meal timing and sleep-wake cycles were the dominant factors influencing periodicity (Biali et al., Citation1995).
To our knowledge only one study has examined the metabolic response to food with regard to defined circadian status in a polar region (75°S, i.e., Antarctica). This was in the context of adapting to night work and is discussed in the next section.
Shiftwork
There is little information on the circadian aspects of shiftwork in polar regions, although there are publications in Russian on Arctic industrial workers. The author presumes that night shift forms part of duties in most areas of the Far South and North and that this section is therefore relevant.
Two reports from Halley have shown clearly that the vast majority of night-shift workers adapt the circadian system within 1 wk to a 12-h change in the work-rest schedule, as evidenced using the rhythm of aMT6s in urine. One report described adaptation both in summer and in winter (Lund et al., Citation2001; Midwinter & Arendt, Citation1991; Ross et al., Citation1995). This is highly unusual, as shiftworkers in temperate zones rarely fully adapt to rotating and even permanent night-shift work (Folkard, Citation2008). It is ascribed to the lack of daylight countering adaptation in winter, together with the isolated environment, no social constraints regarding, for example, daytime childcare, and dark and quiet sleeping quarters. In summer, bright daylight during the night shift will evidently help shift the circadian system to the night mode during the hours of sleep and artificial darkness. Sleep during the Antarctic winter was actually better during the night than day shift, probably due to suboptimal entrainment (delayed phase) when performing day work (Ross et al., Citation1995). A similar report concerning shiftwork on Svalbard (Forberg et al., Citation2010) has recently appeared. Adaptation to night work occurred by delay of sleep and the aMT6s rhythm; thus, the delayed sleep time during and after adaptation may be better phased to ensure good sleep. Diurnal preference was closely related to the speed of adaptation to the night shift by delay, with evening types delaying faster than morning types, as would be expected (Ng et al., Citation2003) ().
FIGURE 4. Rate of adaptation to night shift (20:00–08:00 h) in individual subjects at 75°S in relation to their average Horne-Östberg (diurnal preference) score. The rate was derived by subtracting the average acrophase of aMT6s for the 3 d preceding the night shift from the acrophase on d 5 of the night shift. The greater the evening preference, the faster the rate of shift (p < .05). (Unpublished diagram from data described in Ng et al., Citation2003.)
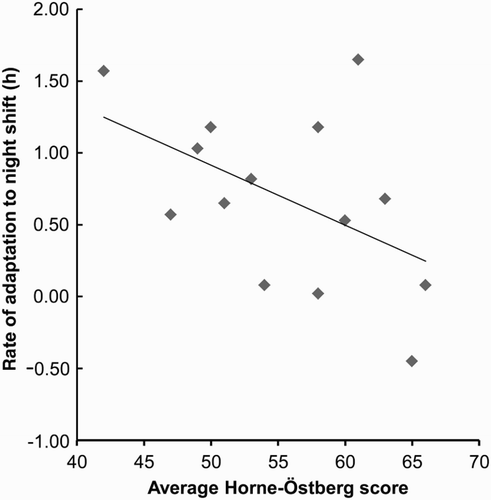
Problems occur, however, when adapted night-shift workers return to day work during the Antarctic winter. There is no strong light-dark cycle to help them return to a normal phase, and subjects can take up to 3 wks, and sometimes more, to realign the circadian system with day work (Broadway & Arendt, Citation1986; Midwinter & Arendt, Citation1991; Ng et al., Citation2003; Ross et al., Citation1995). Free-running may occur as a result of the delayed night-shift phase (), such that it appears easier to maintain entrainment to day work with weak time cues than it is to reestablish entrainment after free-run. This has also been observed in controlled laboratory conditions (Middleton et al., Citation2002).
FIGURE 5. Activity and light exposure (ActiwatchL) recorded at 30-s intervals at 75°S from May 19, 2006, to illustrate winter free-running following a period of night-shift work. Black is activity and gray is light exposure. (Unpublished diagram from data described in Mottram et al., Citation2010.)
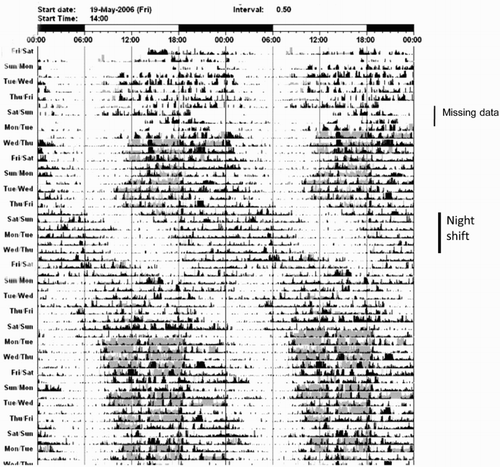
One study has evaluated the metabolic consequences of meals taken either during the day or night shift in Antarctica (75°S). Subjects were provided with a standard meal in the middle of the day shift, in the middle of an unadapted night shift, and in the middle of an adapted night shift. Circadian status (i.e., adaptation) was evaluated by measurement of the aMT6s circadian rhythm. Slow clearance of blood triglycerides and evidence of insulin resistance occurred during the unadapted night shift compared to after the day-shift meal. These problems partially resolved (insulin and glucose response quickly normalized) following return to the day shift, although blood triglycerides did not return rapidly to normal values (Lund et al., Citation2001).
Studies on North Sea oil rigs at Arctic latitudes (e.g., 64°N) are an approximate equivalent, and have a number of features in common with temporary polar service. Oil rig workers usually work offshore for 2–3 wks at a time doing 12-h shifts with various schedules. These shifts may be all nights, all days, or “swing shifts”/”roll-over shifts,” whereby a change from day to night work, or vice versa, is implemented during the time offshore (usually 1 wk of each). The workers are socially isolated and have extended days in summer and nights in winter. When working a night shift from 18:00 to 06:00 h, circadian adaptation (aMT6s rhythm) occurs, and is achieved within a week in most people (Barnes et al., Citation1998a, 1986b; Gibbs et al., Citation2002, 2004). There is, as in Antarctica (), a problem returning to day work (06:00–18:00 h) or to home time, with very large individual variation in response (Gibbs et al., Citation2007). Some people delay back to day work/home time, some advance, and some do not significantly shift at all for the first week after working adapted night shifts. The response appeared to be dependent on initial circadian phase (on d 2 of the night shift). Late-phase people delayed, early-phase people advanced, intermediate-phase people did not shift to readapt to days. Thus, during a week after full adaptation to the night shift, the majority were desynchronized. Similar data regarding individual response of the circadian system at the start of night shift have been reported from studies of nurses in Canada (Dumont et al., Citation2001).
Since initial phase is likely to depend on diurnal preference, it should be possible to predict to some extent the response to abrupt change from nights to days. There is good evidence that diurnal preference assessed by questionnaire (Horne & Östberg, Citation1976; Zavada et al., Citation2005) relates to sleep timing and adaptation to the night shift. The greater the evening preference, the more delayed is the sleep timing (Archer et al., Citation2003) and the more rapid is adaptation to the night shift by delay of the circadian system (). Diurnal preference is also closely concerned with tolerance of polar conditions, and according to one report using the Munich Chronotype Questionnaire (Borisenkov, Citation2010), evening preference is greater with more northerly latitude.
Reinforcing results in simulated shiftwork and in Antarctic shiftworkers, oil rig workers show metabolic abnormalities during desynchrony, which resolve, at least partially, when adapted (Gibbs et al., 2004; Lund et al., Citation2001).
The response to different shift schedules in the North Sea varies, with fewer people fully adapting to a 19:00–07:00 h night shift compared to a 18:00–06:00 h shift (Thorne et al., Citation2008), and with seasonal differences in response that have been attributed to different photoperiods in different seasons (Barnes et al., Citation1998b). The most time spent desynchronized was during a swing shift of 1 wk of 18:00–06:00 h followed by 1 wk of 06:00–18:00 h (Gibbs et al., 2004; see also http://www.hse.gov.uk/research/rrpdf/rr318.pdf for pdf file). It is, of course, likely that the more time spent desynchronized, the greater the risk to health and safety. Timed light treatment designed to hasten readaptation to day work has had some beneficial effects in both Antarctic shift- and oil rig workers. However, it should be emphasized that only small numbers of people have taken part in these studies (Bjorvatn et al., Citation2007, 2010; Midwinter & Arendt, Citation1991; Ross et al., Citation1995; Thorne et al., Citation2010). There is a need for more research involving greater numbers of real shiftworkers.
Overall, it is clear that in polar regions the delayed circadian phase related to the dim-light environment in winter, and probably present in field situations of natural light in summer, needs to be countered. The potential for free-running following night work should be a concern.
Miscellaneous Hormones
Many hormones have circadian rhythm components, but very few have been studied in polar regions from this point of view. Seasonal variations have been reported in a number of reproductive hormones, but few have reported the rhythm characteristics (e.g., Ruhayel et al., Citation2007). Early studies (Kauppila et al., Citation1987; Kivela et al., Citation1988) described an inverse relationship between melatonin and ovarian activity, with increased melatonin and lowered ovarian activity in the winter around 65°N. Frequent sampling to detect changes in circadian rhythms with sufficient resolution to detect small changes is rarely carried out. A large study observing a number of hormones, metabolites, and protein in the serum of 577 men working in Barentsburg (78°N) reported that the major changes observed occurred around the time of the maximum rate of change in photoperiod. Unfortunately, the investigators did not appear to take suitable samples for circadian rhythm analysis or measure personal light exposure (Boiko & Tkachev, Citation1995a, Citation1995b). A short-term study on six men before, at the start, and at the end of 40 d in the Antarctic, with sampling done over the 24 h, described reduced levels of anterior pituitary and adrenal hormones, but no change in circadian rhythms (Farrace et al., Citation2003). The rhythms of urinary free cortisol and salivary cortisol were maintained during the Antarctic year in a structured environment, but these studies had low resolution to detect small shifts in phase (Griffiths et al., Citation1986; Harris et al., Citation2010). Given the changes seen in sleep timing and sleep characteristics, it is surprising that no 24-h changes in growth hormone or prolactin have been reported to the author's knowledge, both of these hormones being strongly influenced by sleep.
Most attention has centered on a seasonal variation in thyroid function, referred to as the polar triiodothyronine syndrome (Palinkas et al., Citation2001; Reed et al., Citation1986, Citation1988, Citation2001). There is some inconsistency in the data, but the consensus would be that thyroid-stimulating hormone (TSH) is elevated and thyroid products (usually free triiodothyronine [fT3]) are lowered during the polar winter. In some reports, total free thyroxine [fT4], total T4, and T3 are lowered. These changes have been associated with decrements in mood and performance (Palinkas et al., Citation2001; Reed et al., Citation2001). In general, the samples that were taken for analysis do not permit circadian evaluation of thyroid hormone status. TSH has a marked circadian rhythm, normally peaking at night. If this rhythm is delayed, as might be expected during the winter, morning levels are likely to be raised simply because the peak has shifted to later in the night, spilling over to the morning. The question is not resolved as to whether the overall level of TSH is higher or the rhythm has shifted. However, interestingly when bright light treatment was compared with thyroxine/triiodothyronine supplementation, the bright light was efficient at decreasing TSH, increasing fT3, and prevented anger and depressive symptoms in winter (Palinkas et al., Citation2010). A change in rhythm is likely to have been evoked by the light treatment and may be the primary explanation for these observations. This conclusion is reinforced by another study (Hassi et al., Citation2001) that showed fT3 levels did not correlate with TSH, but that TSH levels were strongly correlated with luminosity. On the other hand, outdoor temperature correlated with fT3, and the authors suggest that disposal of thyroid products is accelerated in winter, leading to high urinary fT3.
Cardiovascular Rhythms
Cardiovascular functions are affected not only by the sleep-wake cycle but are also directly controlled by the central circadian clock (SCN) (Ruger & Scheer, Citation2009). Following early work describing desynchrony in cardiac functions in the Antarctic (D'Yachkov & Moshkin, 1981; Moshkin, Citation1984), more recent literature provides contradictory findings. Cugini and colleagues (Citation1997) reported perfect synchrony of the blood pressure rhythm to the 24-h day during ambulatory monitoring in the Antarctic summer in clinically healthy subjects. In contrast, Gapon and colleagues (Citation2005, 2009) report desynchrony in cardiac functions and structural changes in the myocardium of hypertensive patients in the Russian North. Exposure to cold climate increases blood pressure (Leppaluoto & Hassi, Citation1991) and a further brief scan of the literature suggests that hypertension is of concern in Arctic Russia.
Sex Considerations
There is far more information concerning men's than of women's physiology in polar regions. The available information suggests that women have more sleep and mood problems than men (Husby & Lingjaerde, Citation1990; Palinkas et al., Citation2004), and based on data from the Rand 36-item general health questionnaire at 75°S that they suffer more than men from prolonged Antarctic residence (Mottram et al., Citation2010). SAD afflicts women more than men for unknown reasons. Reproductive health requires study. It is imperative to gather more information regarding women's physiology and pathology during residence in polar regions.
CONCLUSIONS
The lack of natural bright light during the polar winter means that the main time cue for the organization of circadian rhythms is greatly diminished. In summer with daylight for 24-h, light exposure can adversely affect the circadian system by undesired forced phase shift, but the use of blinds, eye masks, and suitably scheduled sleep should counteract this effect. Circadian rhythms are frequently and significantly delayed during the lack of natural daylight in winter (and also in some conditions in summer), such that the clock time for scheduled sleep is too early and sleep is attempted at a suboptimal phase (internal desynchronization). The main objective outcomes are late sleep timing, with lower sleep efficiency. Subjectively, it is difficult to get to sleep, and sleep is of lower quality. Decrements in sleep lead to poor alertness and performance, with possible long-term health consequences. Delayed circadian rhythms are often associated with SAD, and, although SAD does not appear to be very prevalent in temporary polar residents, low mood (subsyndromal SAD) is reported. If more women are to be deployed to polar regions, it is likely that the incidence of SAD will increase.
The available information suggests that internal desynchrony will be associated with metabolic abnormalities, particularly risk factors for heart disease, and possibly cancer. Similarly, cardiac function and, indeed, all physiology governed by circadian rhythms (Hastings et al., Citation2003) is likely to be affected. Subjects free-run infrequently in a structured polar environment in winter, but this does happen, especially after a period of night shift. Most readily adapt to the night shift, but the readaptation to day work is often very slow in winter. All of these problems can be addressed by maintaining a consolidated and suitable circadian phase. The treatment of choice, both in winter and summer, is carefully timed light exposure and avoidance as a function of endogenous phase, with or without carefully timed melatonin treatment.
Relevance of Polar Observations to Other Regions
Polar regions provide a useful environment for optimizing the intensity and spectral composition of ambient light. Many circumstances, e.g., high latitudes in winter and short photoperiods together with an interior workplace, are associated with a lack of bright natural light. In midwinter in the UK, for example, London at 52°N, travel to and from work will often be in artificial light, since from 4 December to 7 January the sun rises after 08:00 h and sets before 16:00 h. Workstations may well have no windows, and outdoor activity at lunchtime or during daylight hours may be difficult, if not undesirable in poor weather conditions. Thus, data from polar regions are likely to have applicability in urban environments with winter daylengths shorter than the working day.
FUTURE WORK
It is essential to define the optimum conditions for maintaining circadian phase in Antarctic and Arctic deployments. Specifically, ambient light in the different environments, appropriate light treatment, and use of melatonin need to be considered. There is little objective information on personal light exposure, sleep, and circadian status during temporary residence in the Arctic. Conditions in the built environment will differ from those in the field, and data are needed from both situations. Fortunately, the availability of automatic personal activity/sleep and light recording devices with large memories provides the opportunity to acquire sleep/activity and light-exposure information. Circadian phase can to some extent be gauged by the time of mid-sleep, or natural, i.e., not with an alarm, wake-up time. But, it is more accurate to measure the phase and, preferably, also the amplitude of the melatonin rhythm. Both saliva and urine sampling can provide suitable information, and both are possible in polar environments. Measurement of aMT6s in urine provides amplitude as well as phase information without disturbance of sleep. Saliva samples provide a better resolution with regard to phase, but overnight sampling, requiring the waking of subjects, is essential for a measure of amplitude.
Several features of the use of light and melatonin to treat circadian desynchrony and maintain optimal sleep and alertness remain unclear and require further study. These include the exact duration of light exposure, which may be amenable to further reduction, the optimal spectral composition of light treatment, and the “hangover” or residual effect on some parameters when the effects of light may persist after its withdrawal. Existing evidence suggests that short-wavelength light (blue/green) should be most effective and possibly more economical with regard to fuel requirements. Anecdotally (author's experience), blue-enriched light was disliked by some Antarctic subjects, but appreciated by others. Monochromatic green light as a 2-h pulse from a light tower, for melatonin suppression and phase shifting, was best tolerated in laboratory experiments (Paul et al., Citation2007), but in another study its effects were short-lived compared to monochromatic blue light (Gooley et al., Citation2010). The authors of the latter report suggest that light treatment should be directed to both cone photoreceptors and melanopsin cells for maximum effect.
Similarly, the dose and timing of melatonin have never been studied, to the author's knowledge, in polar regions. Laboratory experiments suggest that a small dose (.5–3 mg) of sustained or fast-release melatonin should be appropriate to phase advance delayed circadian rhythms (Burgess et al., Citation2010; Deacon & Arendt, Citation1995), and an even lower dose (.5 mg or less) of fast-release melatonin should be appropriate for phase delay (Paul et al., Citation2011). It is difficult to judge timing correctly in conditions of circadian desynchrony, which vary from one individual to another. Thus, a knowledge of circadian status during temporary Arctic or Antarctic residence is important. In fact, it may be possible to avoid desynchrony by initiating treatment just prior to exposure to polar conditions. Since there is good evidence the effects of light and melatonin reinforce each other when correctly timed (e.g., Paul et al., 2010, Citation2011; Revell et al., Citation2006), the use of both, in combination, is likely to be the most efficient treatment for maintaining circadian phase.
ACKNOWLEDGMENTS
Studies by the author, referred to in this review, were supported throughout by the British Antarctic Survey, and in part by the Antarctic Funding Initiative, the Medical Research Council, the Institute of Petroleum, and The Energy Institute. Philips Lighting B.V. Eindhoven provided lighting equipment and an unrestricted research grant. Dr. Benita Middleton (Stockgrand Ltd., University of Surrey) supplied reagents, expertise, advice, and unlimited support. In addition to those mentioned by publication herein, the author wishes to thank all the Halley base doctors who have carried out chronobiology research projects at the ends of the Earth and all the willing (and unpaid) volunteers who took part in these studies. She would also like to thank Dr. Iain Grant, British Antarctic Survey Medical Unit, UK, Dr. Benita Middleton, University of Surrey, UK, and Michel Paul, Defense Research and Development, Canada, for their expert review of the manuscript.
Declaration of Interest: The author is a director and major shareholder of Stockgrand Ltd., which supplied reagents and expertise for Antarctic studies. This review was written during the tenure of a consultancy with the Canadian Ministry of Defense. The author reports no conflicts of interest. The author alone is responsible for the content and writing of the paper.
REFERENCES
- Allen NH, Kerr D, Smythe PJ, Martin N, Osola K, Thompson C. (1992). Insulin sensitivity after phototherapy for seasonal affective disorder. Lancet 339:1065–1066.
- Archer SN, Robilliard DL, Skene DJ, Smits M, Williams A, Arendt J, Von Schantz M. (2003). A length polymorphism in the circadian clock gene Per3 is linked to delayed sleep phase syndrome and extreme diurnal preference. Sleep 26:413–415.
- Arendt J. (1986). Role of the pineal gland and melatonin in seasonal reproductive function in mammals. Oxford Rev. Rreprod. Biol. 8:266–320.
- Arendt J. (1995). Melatonin and the mammalian pineal gland. London: Chapman Hall, 331 pp.
- Arendt J. (1999). Is melatonin a photoperiodic signal in humans? Adv. Exp.Med. Biol. 460:417–424.
- Barnes RG, Deacon SJ, Forbes MJ, Arendt J. (1998a). Adaptation of the 6-sulphatoxymelatonin rhythm in shiftworkers on offshore oil installations during a 2-week 12-h night shift. Neurosci. Lett. 241:9–12.
- Barnes RG, Forbes MJ, Arendt J. (1998b). Shift type and season affect adaptation of the 6-sulphatoxymelatonin rhythm in offshore oil rig workers. Neurosci. Lett. 252:179–182.
- Bhattacharyya M, Pal MS, Sharma YK, Majumdar D. (2008). Changes in sleep patterns during prolonged stays in Antarctica. Int. J. Biometeorol. 52:869–879.
- Biali S, Jones PJ, Pederson RA, Iqbal I, Suedfeld P. (1995). Influence of a perpetual-daylight Arctic environment on periodicity in human cholesterol synthesis. Arctic Med. Res. 54:134–144.
- Bjorvatn B, Kecklund G, Åkerstedt T. (1999). Bright light treatment used for adaptation to night work and re-adaptation back to day life. A field study at an oil platform in the North Sea. J. Sleep Res. 8:105–112.
- Bjorvatn B, Stangenes K, Oyane N, Forberg K, Lowden A, Holsten F, Åkerstedt T. (2007). Randomized placebo-controlled field study of the effects of bright light and melatonin in adaptation to night work. Scand. J. Work Environ. Health 33:204–214.
- Blatter K, Cajochen C. (2007). Circadian rhythms in cognitive performance: methodological constraints, protocols, theoretical underpinnings. Physiol. Behav. 90:196–208.
- Boiko ER, Tkachev AV. (1995a). [The effect of the duration of daylight on human hormonal and biochemical indices in the north]. Fiziologicheskii zhurnal imeni I.M. Sechenova/Rossiiskaia akademiia nauk 81:86–92.
- Boiko YR, Tkachev AV. (1995b). The effect of daylength on hormonal and biochemical indicators in humans in the North. Sechenov Physiol. J. 81:86–92.
- Bojkowski CJ, Arendt J. (1988). Annual changes in 6-sulphatoxymelatonin excretion in man. Acta Endocrinol. (Copenh.) 117:470–476.
- Borisenkov MF. (2010). Human chronotypes in the north. Hum. Physiol. 36:348–352.
- Borisenkov MF. (2011). The pattern of entrainment of the human sleep-wake rhythm by the natural photoperiod in the north. Chronobiol. Int. 28:921–929.
- Bratlid T, Wahlund B. (2003). Alterations in serum melatonin and sleep in individuals in a sub-arctic region from winter to spring. Int. J. Circumpolar Health 62:242–254.
- Broadway J, Arendt J. (1986). Delayed recovery of sleep and melatonin rhythms after nightshift work in Antarctic winter. Lancet 2:813–814.
- Broadway J, Arendt J, Folkard S. (1987a). Bright light phase shifts the human melatonin rhythm during the Antarctic winter. Neurosci. Lett. 79:185–189.
- Broadway J, Arendt J, Folkard S. (1987b). Bright light phase shifts the human melatonin rhythm during the Antarctic winter. Neurosci. Lett. 79:185–189.
- Buck L. (1980). Circadian rhythms of performance among workers in the Arctic. Aviat. Space Environ. Med. 51:805–808.
- Buguet A, Rivolier J, Jouvet M. (1987). Human sleep patterns in Antarctica. Sleep 10:374–382.
- Buresova M, Dvorakova M, Zvolsky P, Illnerova H. (1992). Human circadian rhythm in serum melatonin in short winter days and in simulated artificial long days. Neurosci. Lett. 136:173–176.
- Burgess HJ, Revell VL, Molina TA, Eastman CI. (2010). Human phase response curves to three days of daily melatonin: 0.5 mg versus 3.0 mg. J. Clin. Endocrinol. Metab. 95:3325–3331.
- Campbell IT, Jarrett RJ, Keen H. (1975a). Diurnal and seasonal variation in oral glucose tolerance: studies in the Antarctic. Diabetologia 11:139–145.
- Campbell IT, Jarrett RJ, Rutland P, Stimmler L. (1975b). The plasma insulin and growth hormone response to oral glucose: diurnal and seasonal observations in the Antarctic. Diabetologia 11:147–150.
- Carter DS, Goldman BD. (1983). Antigonadal effects of timed melatonin infusion in pinealectomized male Djungarian hamsters (Phodopus sungorus sungorus): duration is the critical parameter. Endocrinology 113:1261–1267.
- Corbett R. (2011). Timing of artificial light exposure on sleep and circadian rhythm during Antarctic winter. MSc thesis in Remote Health Care, University of Plymouth, Plymouth, UK, 63 pp.
- Cugini P, Camillieri G, Alessio L, Cristina G, Petrangeli CM, Capodaglio PF. (1997). Ambulatory blood pressure monitoring in clinically healthy subjects adapted to living in Antarctica. Aviat. Space Environ. Med. 68:795–801.
- Czeisler CA, Johnson MP, Duffy JF, Brown EN, Ronda JM, Kronauer RE. (1990). Exposure to bright light and darkness to treat physiologic maladaptation to night work. N. Engl. J. Med. 322:1253–1259.
- D'yachkov VA, Moshkin MP. (1981). Circadian organization of the human circulatory and respiratory systems in Antarctica. Hum. Physiol. 7:414–417.
- Deacon S, Arendt J. (1995). Melatonin-induced temperature suppression and its acute phase-shifting effects correlate in a dose-dependent manner in humans. Brain Res. 688:77–85.
- Dumont M, Benhaberou-Brun D, Paquet J. (2001). Profile of 24-h light exposure and circadian phase of melatonin secretion in night workers. J. Biol. Rhythms 16:502–511.
- Farrace S, Ferrara M, De Angelis C, Trezza R, Cenni P, Peri A, Casagrande M, De Gennaro L. (2003). Reduced sympathetic outflow and adrenal secretory activity during a 40-day stay in the Antarctic. Int. J. Psychophysiol. 49:17–27.
- Folkard S. (2008). Do permanent night workers show circadian adjustment? A review based on the endogenous melatonin rhythm. Chronobiol. Int. 25:215–224.
- Forberg K, Waage S, Moen B, Bjorvatn B. (2010). Subjective and objective sleep and sleepiness among tunnel workers in an extreme and isolated environment: 10-h shifts, 21-day working period, at 78 degrees north. Sleep Med. 11:185–190.
- Francis G, Bishop L, Luke C, Middleton B, Williams P, Arendt J. (2008). Sleep during the Antarctic winter: preliminary observations on changing the spectral composition of artificial light. J. Sleep Res. 17:354–360.
- Fuller PM, Lu J, Saper CB. (2008). Differential rescue of light- and food-entrainable circadian rhythms. Science 320:1074–1077.
- Gander PH, Macdonald JA, Montgomery JC, Paulin MG. (1991). Adaptation of sleep and circadian rhythms to the Antarctic summer: a question of zeitgeber strength. Aviat. Space Environ. Med. 62:1019–1025.
- Gapon LI, Shurkevich NP, Vetoshkin AS. (2005). [Structural changes of the myocardium and 24-hour blood pressure profile in subjects with hypertension studied during expedition work in the Far North]. Kardiologiia 45:51–56.
- Gapon LI, Shurkevich NP, Vetoshkin AS. (2009). [Structural and functional changes in the heart and 24-hour arterial pressure profile in patients with arterial hypertension in the Far North]. Klin. Med. (Mosk.) 87:23–29.
- Gibbs M, Hampton S, Morgan L, Arendt J. (2002). Adaptation of the circadian rhythm of 6-sulphatoxymelatonin to a shift schedule of seven nights followed by seven days in offshore oil installation workers. Neurosci. Lett. 325:91–94.
- Gibbs M, Hampton SH. (2004). Effect of shift schedule on offshore shift workers' circadian rhythms and health. Accessed on February 2005 from http://www.hse.gov.uk/research/rrpdf/rr318.pdf.
- Gibbs M, Hampton S, Morgan L, Arendt J. (2007). Predicting circadian response to abrupt phase shift: 6-sulphatoxymelatonin rhythms in rotating shift workers offshore. J. Biol. Rhythms 22:368–370.
- Gooley JJ, Rajaratnam SM, Brainard GC, Kronauer RE, Czeisler CA, Lockley SW. (2010). Spectral responses of the human circadian system depend on the irradiance and duration of exposure to light. Sci. Transl. Med. 2:31ra33.
- Griffiths PA, Folkard S, Bojkowski C, English J, Arendt J. (1986). Persistent 24-h variations of urinary 6-hydroxy melatonin sulphate and cortisol in Antarctica. Experientia 42:430–432.
- Gronfier C, Wolf L, Schlangen L, Naijar R, Taillard J, Cajochen C. (2010). The Concordia Antarctic Project. J. Sleep Res. 19:37.
- Hampton SM, Morgan LM, Lawrence N, Anastasiadou T, Norris F, Deacon S, Ribeiro D, Arendt J. (1996). Postprandial hormone and metabolic responses in simulated shift work. J. Endocrinol. 151:259–267.
- Hansen T, Bratlid T, Lingjarde O, Brenn T. (1987). Midwinter insomnia in the subarctic region: evening levels of serum melatonin and cortisol before and after treatment with bright artificial light. Acta Psychiatr. Scand. 75:428–434.
- Harris A, Marquis P, Eriksen HR, Grant I, Corbett R, Lie SA, Ursin H. (2010). Diurnal rhythm in British Antarctic personnel. Rural Remote Health 10:1351–1363.
- Hassi J, Sikkila K, Ruokonen A, Leppaluoto J. (2001). The pituitary-thyroid axis in healthy men living under subarctic climatological conditions. J. Endocrinol. 169:195–203.
- Hastings MH, Reddy AB, Maywood ES. (2003). A clockwork web: circadian timing in brain and periphery, in health and disease. Nat. Rev. Neurosci. 4:649–661.
- Holmback U, Forslund A, Forslund J, Hambraeus L, Lennernas M, Lowden A, Stridsberg M, Åkerstedt T. (2002). Metabolic responses to nocturnal eating in men are affected by sources of dietary energy. J. Nutr. 132:1892–1899.
- Horne JA, Östberg O. (1976). A self-assessment questionnaire to determine morningness-eveningness in human circadian rhythms. Int. J. Chronobiol. 4:97–110.
- Husby R, Lingjaerde O. (1990). Prevalence of reported sleeplessness in northern Norway in relation to sex, age and season. Acta Psychiatr. Scand. 81:542–547.
- Johnsson A, Engelmann W, Klemke W, Ekse AT. (1979). Free-running human circadian rhythms in Svalbard. Z. Naturforsch. C 34C:470–473.
- Johnsson A, Engelmann W, Pflug B, Klemke W. (1983). Period lengthening of human circadian rhythms by lithium carbonate, a prophylactic for depressive disorders. Int. J. Chronobiol. 8:129–147.
- Kauppila A, Kivela A, Pakarinen A, Vakkuri O. (1987). Inverse seasonal relationship between melatonin and ovarian activity in humans in a region with a strong seasonal contrast in luminosity. J. Clin. Endocrinol. Metab. 65:823–828.
- Kennaway DJ, Van Dorp CF. (1991). Free-running rhythms of melatonin, cortisol, electrolytes, and sleep in humans in Antarctica. Am. J. Physiol. 260:R1137–R1144.
- Kivela A, Kauppila A, Ylostalo P, Vakkuri O, Leppaluoto J. (1988). Seasonal, menstrual and circadian secretions of melatonin, gonadotropins and prolactin in women. Acta Physiol. Scand. 132:321–327.
- Krauchi K, Cajochen C, Werth E, Wirz-Justice A. (2002). Alteration of internal circadian phase relationships after morning versus evening carbohydrate-rich meals in humans. J. Biol. Rhythms 17:364–376.
- Leppaluoto J, Hassi J. (1991). Human physiological adaptations to the Arctic climate. Arctic 44:139–145.
- Levine ME. (1995). Seasonal symptoms in the sub-Arctic. Mil. Med. 160:110–114.
- Lewis HE, Masterton JP. (1957). Sleep and wakefulness in the arctic. Lancet 269:1262–1266.
- Lewis PR, Lobban MC. (1957). Dissociation of diurnal rhythms in human subjects living on abnormal time routines. Q. J. Exp. Physiol. Cogn. Med. Sci. 42:371–386.
- Lewy AJ, Wehr TA, Goodwin FK, Newsome DA, Markey SP. (1980). Light suppresses melatonin secretion in humans. Science 210:1267–1269.
- Lewy AJ, Lefler BJ, Emens JS, Bauer VK. (2006). The circadian basis of winter depression. Proc. Natl. Acad. Sci. U. S. A. 103:7414–7419.
- Lingjaerde O, Bratlid T. (1981). Triazolam (Halcion) versus flunitrazepam (Rohypnol) against midwinter insomnia in northern Norway. Acta Psychiatr. Scand. 64:260–269.
- Lingjaerde O, Bratlid T, Westby OC, Gordeladze JO. (1983). Effect of midazolam, flunitrazepam, and placebo against midwinter insomnia in northern Norway. Acta Psychiatr. Scand. 67:118–129.
- Lingjaerde O, Bratlid T, Hansen T. (1985). Insomnia during the “dark period” in northern Norway. An explorative, controlled trial with light treatment. Acta Psychiatr. Scand. 71:506–512.
- Lobban MC. (1967). Daily rhythms of renal excretion in Arctic-dwelling Indians and Eskimos. Q. J. Exp. Physiol. Cogn. Med. Sci. 52:401–410.
- Lockley SW, Brainard GC, Czeisler CA. (2003). High sensitivity of the human circadian melatonin rhythm to resetting by short wavelength light. J. Clin. Endocrinol. Metab. 88:4502–4505.
- Lockley SW, Dijk DJ, Kosti O, Skene DJ, Arendt J. (2008). Alertness, mood and performance rhythm disturbances associated with circadian sleep disorders in the blind. J. Sleep Res. 17:207–216.
- Lund J, Arendt J, Hampton SM, English J, Morgan LM. (2001). Postprandial hormone and metabolic responses amongst shift workers in Antarctica. J. Endocrinol. 171:557–564.
- Magnusson A, Axelsson J, Karlsson MM, Oskarsson H. (2000). Lack of seasonal mood change in the Icelandic population: results of a cross-sectional study. Am. J. Psychiatry 157:234–238.
- Makinen TM. (2007). Human cold exposure, adaptation, and performance in high latitude environments. Am. J. Hum. Biol. 19:155–164.
- Makkison I, Arendt J. (1991). Melatonin secretion on two different Antarctic Bases (68°S and 75°S). J. Interdiscipl. Cycle Res. 22:149–150.
- Malhotra AS, Pal K, Prasad R, Bajaj AC, Kumar R, Sawhney RC. (1998). Plasma insulin and growth hormone during antarctic residence. Jpn. J. Physiol. 48:167–169.
- Middleton B, Stone BM, Arendt J. (2002). Human circadian phase in 12:12 h, 200:<8 lux and 1000:<8 lux light-dark cycles, without scheduled sleep or activity. Neurosci. Lett. 329:41–44.
- Midwinter MJ, Arendt J. (1991). Adaptation of the melatonin rhythm in human subjects following night-shift work in Antarctica. Neurosci. Lett. 122:195–198.
- Morgan L, Hampton S, Gibbs M, Arendt J. (2003). Circadian aspects of postprandial metabolism. Chronobiol. Int. 20:795–808.
- Moshkin MP. (1984). Effect of natural light-dark cycle on biorhythms in polar scientists. Hum. Physiol. 10:63–66.
- Mottram V, Middleton B, Williams P, Arendt J. (2010). The impact of bright artificial white and ‘blue-enriched’ light on sleep and circadian phase during the polar winter. J. Sleep Res. 20:154–161.
- Natani K, Shurley JT, Pierce CM, Brooks RE. (1970). Long-term changes in sleep patterns in men on the South Polar Plateau. Arch. Intern. Med. 125:655–659.
- Ng L, Morgan L, Arendt J. (2003). Circadian adaptation in Antarctic shift workers: relationship to diurnal preference, season and subjective sleep. Chronobiol. Int. 20:1162–1164
- Nilssen O, Lipton R, Brenn T, Hoyer G, Boiko E, Tkatchev A. (1997). Sleeping problems at 78 degrees north: the Svalbard Study. Acta Psychiatr. Scand. 95:44–48.
- O'Conor R. (1989). British Antarctic Survey medical report. Cambridge, UK: British Antarctic Survey.
- Owen J, Arendt J. (1992). Melatonin suppression in human subjects by bright and dim light in Antarctica: time and season-dependent effects. Neurosci. Lett. 137:181–184.
- Paakkonen T, Leppaluoto J, Makinen TM, Rintamaki H, Ruokonen A, Hassi J, Palinkas LA. (2008). Seasonal levels of melatonin, thyroid hormones, mood, and cognition near the Arctic Circle. Aviat. Space Environ. Med. 79:695–699.
- Palinkas LA. (1986). Health and performance of Antarctic winter-over personnel: a follow-up study. Aviat. Space Environ. Med. 57:954–959.
- Palinkas LA. (1987). A longitudinal study of disease incidence among Antarctic winter-over personnel. Aviat. Space Environ. Med. 58:1062–1065.
- Palinkas LA, Suedfeld P. (2008). Psychological effects of polar expeditions. Lancet 371:153–163.
- Palinkas LA, Cravalho M, Browner D. (1995). Seasonal variation of depressive symptoms in Antarctica. Acta Psychiatr. Scand. 91:423–429.
- Palinkas LA, Houseal M, Miller C. (2000). Sleep and mood during a winter in Antarctica. Int. J. Circumpolar Health 59:63–73.
- Palinkas LA, Reed HL, Reedy KR, Do NV, Case HS, Finney NS. (2001). Circannual pattern of hypothalamic-pituitary-thyroid (HPT) function and mood during extended Antarctic residence. Psychoneuroendocrinology 26:421–431.
- Palinkas LA, Glogower F, Dembert M, Hansen K, Smullen R. (2004). Incidence of psychiatric disorders after extended residence in Antarctica. Int. J. Circumpolar Health 63:157–168.
- Palinkas LA, Reedy KR, Shepanek M, Reeves D, Samuel Case H, Van Do N, Lester Reed H. (2010). A randomized placebo-controlled clinical trial of the effectiveness of thyroxine and triiodothyronine and short-term exposure to bright light in prevention of decrements in cognitive performance and mood during prolonged Antarctic residence. Clin. Endocrinol. 72:543–550.
- Paterson RA. (1975). Letter: Seasonal reduction of slow-wave sleep at an Antarctic coastal station. Lancet 1:468–469.
- Paul MA, Miller JC, Gray G, Buick F, Blazeski S, Arendt J. (2007). Circadian phase delay induced by phototherapeutic devices. Aviat. Space Environ. Med. 78:645–652.
- Paul MA, Gray GW, Lieberman HR, Love RJ, Miller JC, Trouborst M, Arendt J. (2011). Phase advance with separate and combined melatonin and light treatment. Psychopharmacology 214:515–523.
- Popkin MK, Stillner V, Osborn LW, Pierce CM, Shurley JT. (1974). Novel behaviors in an extreme environment. Am. J. Psychiatry 131:651–654.
- Reed HL, Burman KD, Shakir KM, O'brian JT. (1986). Alterations in the hypothalamic-pituitary-thyroid axis after prolonged residence in Antarctica. Clin. Endocrinol. 25:55–65.
- Reed HL, Ferreiro JA, Shakir KMM, Burman KD, O'brian JT. (1988). Pituitary and peripheral hormone responses to T3 administration during Antarctic residence. Am. J. Physiol. Endocrinol. Metab. 254:E733–E739.
- Reed HL, Reedy KR, Palinkas LA, Van Do N, Finney NS, Case HS, Lemar HJ, Wright J, Thomas J. (2001). Impairment in cognitive and exercise performance during prolonged antarctic residence: effect of thyroxine supplementation in the polar triiodothyronine syndrome. J. Clin. Endocrinol. Metab. 86:110–116.
- Reilly T, Edwards B. (2007). Altered sleep-wake cycles and physical performance in athletes. Physiol. Behav. 90:274–284.
- Reilly T, Waterhouse J, Edwards B. (2005). Jet lag and air travel: implications for performance. Clin. Sports Med. 24:367–380.
- Revell VL, Arendt J, Terman M, Skene DJ. (2005). Short-wavelength sensitivity of the human circadian system to phase-advancing light. J. Biol. Rhythms 20:270–272.
- Revell VL, Burgess HJ, Gazda CJ, Smith MR, Fogg LF, Eastman CI. (2006). Advancing human circadian rhythms with afternoon melatonin and morning intermittent bright light. J. Clin. Endocrinol. Metab. 91:54–59.
- Ribeiro DC, Hampton SM, Morgan L, Deacon S, Arendt J. (1998). Altered postprandial hormone and metabolic responses in a simulated shift work environment. J. Endocrinol. 158:305–310.
- Rosenthal NE, Sack DA, Gillin JC, Lewy AJ, Goodwin FK, Davenport Y, Mueller PS, Newsome DA, Wehr TA. (1984). Seasonal affective disorder. A description of the syndrome and preliminary findings with light therapy. Arch. Gen. Psychiatry 41:72–80.
- Ross JK, Arendt J, Horne J, Haston W. (1995). Night-shift work in Antarctica: sleep characteristics and bright light treatment. Physiol. Behav. 57:1169–1174.
- Ruger M, Scheer FA. (2009). Effects of circadian disruption on the cardiometabolic system. Rev. Endocrinol. Metab. Dis. 10:245–260.
- Ruhayel Y, Malm G, Haugen TB, Henrichsen T, Bjorsvik C, Grotmol T, Saether T, Malm J, Figenschau Y, Rylander L, Levine RJ, Giwercman A. (2007). Seasonal variation in serum concentrations of reproductive hormones and urinary excretion of 6-sulfatoxymelatonin in men living north and south of the Arctic Circle: a longitudinal study. Clin. Endocrinol. 67:85–92.
- Schylter P. (2008). Radiometry and photometry in astronomy. Accessed on September 10, 1997 from stjarnhimlen.se/comp/radfaq.html.
- Sopowski MJ, Hampton SM, Ribeiro DC, Morgan L, Arendt J. (2001). Postprandial triacylglycerol responses in simulated night and day shift: gender differences. J. Biol. Rhythms 16:272–276.
- Steel GD, Callaway M, Suedfeld P, Palinkas L. (1995). Human sleep-wake cycles in the high Arctic: effects of unusual photoperiodicity in a natural setting. Biol. Rhythm Res. 26:582–592.
- Stokkan KA, Reiter RJ. (1994). Melatonin rhythms in Arctic urban residents. J. Pineal Res. 16:33–36.
- Takasu NN, Hashimoto S, Yamanaka Y, Tanahashi Y, Yamazaki A, Honma S, Honma K. (2006). Repeated exposures to daytime bright light increase nocturnal melatonin rise and maintain circadian phase in young subjects under fixed sleep schedule. Am. J. Physiol. Regul. Integr. Comp. Physiol. 291:R1799–R1807.
- Terman M, Jiuan Su T. (2010). Circadian rhythm phase advance with dawn simulation treatment for winter depression. J. Biol. Rhythms 25:297–301.
- Terman JS, Terman M, Lo ES, Cooper TB. (2001). Circadian time of morning light administration and therapeutic response in winter depression. Arch. Gen. Psychiatry 58:69–75.
- Thorne H, Hampton S, Morgan L, Skene DJ, Arendt J. (2008). Differences in sleep, light, and circadian phase in offshore 18:00–06:00 h and 19:00–07:00 h shift workers. Chronobiol. Int. 25:225–235.
- Thorne H, Hampton S, Morgan L, Skene DJ, Arendt J. (2010). Returning from night shift to day life; beneficial effects of light on sleep. Sleep Biol. Rhythms 8:212–221.
- Usui A, Obinata I, Ishizuka Y, Okado T, Fukuzawa H, Kanba S. (2000). Seasonal changes in human sleep-wake rhythm in Antarctica and Japan. Psychiatry Clin. Neurosci. 54:361–362.
- Van Cauter E, Desir D, Decoster C, Fery F, Balasse EO. (1989). Nocturnal decrease in glucose tolerance during constant glucose infusion. J. Clin. Endocrinol. Metab. 69:604–611.
- Van Cauter E, Polonsky KS, Scheen AJ. (1997). Roles of circadian rhythmicity and sleep in human glucose regulation. Endocr. Rev. 18:716–738.
- Waterhouse J, Drust B, Weinert D, Edwards B, Gregson W, Atkinson G, Kao S, Aizawa S, Reilly T. (2005). The circadian rhythm of core temperature: origin and some implications for exercise performance. Chronobiol. Int. 22:207–225.
- Wehr TA. (1991). The durations of human melatonin secretion and sleep respond to changes in daylength (photoperiod). J. Clin. Endocrinol. Metab. 73:1276–1280.
- Wehr TA, Duncan WC, Sher L, Aeschbach D, Schwartz PJ, Turner EH, Postolache TT, Rosenthal NE. (2001). A circadian signal of change of season in patients with seasonal affective disorder. Arch. Gen. Psychiatry 58:1108–1114.
- Weydahl A. (1991). Sleep-quality among girls with different involvement in competitive sports during fall in the Arctic Circle. Percept. Mot. Skills 73:883–892.
- Weydahl A. (1994). Evening melatonin in January after changes in hours of habitual exercise during fall among youths living in the subarctic. Arctic Med. Res. 53:146–151.
- Wirz-Justice A, Benedetti F, Terman M. (2009). Chronotherapeutics for affective disorders: a clinician's manual for light and wake therapy. Basel: Karger, 116 pp.
- Wortmann H, Knauth P, Schweflinghaus W. (1999). Schlafverhalten und zirkadiane variation der befindlichkeit unter dem einfluss unterschiedlicher tageslichtverhaltnisse in der antarktis. [Effects of different daylight conditions in the Antarctic on sleeping behaviour, circadian variations of fatigue, alertness and extraversion/introversion.] Somnologie 3:289–299.
- Wright KPJ, Hull JT, Hughes RJ, Ronda JM, Czeisler CJ. (2006). Sleep and wakefulness out of phase with internal biological time impairs learning in humans. Cogn. Neurosci. 18:508–521.
- Yoneyama S, Hashimoto S, Honma K. (1999). Seasonal changes of human circadian rhythms in Antarctica. Am. J. Physiol. 277:R1091–R1097.
- Zavada A, Gordijn MC, Beersma DG, Daan S, Roenneberg T. (2005). Comparison of the Munich Chronotype Questionnaire with the Horne-Östberg's Morningness-Eveningness Score. Chronobiol. Int. 22:267–278.