Abstract
Introduction. Low birth-weight is associated with an increased risk of cardiovascular disease, hypertension, and the metabolic syndrome (MetS) in adulthood. Resting metabolic rate (RMR) has been suggested to be associated with the development of obesity as well as MetS and might be an indirect indicator of sympathetic activity. This study's aim was to examine the association between birth-weight and adult RMR.
Methods. A total of 896 men and women from the Helsinki Birth Cohort Study born 1934–44, for whom a detailed set of birth records were available, underwent measurement of body composition and RMR in adulthood.
Results. Among women, birth-weight adjusted for age and fat-free mass (FFM) was inversely associated with RMR (r = −0.12; P < 0.01). For men, a u-shaped relationship was observed, both independently and after adjustment for age, fat mass, and FFM (P = 0.05 for final model).
Discussion. The sex-specific differences for the association between birth-weight and adult RMR might partly be explained by differences in the developmental programming of the sympathetic nervous system between men and women. The higher adjusted RMR among those with the lowest birth-weights is consistent with previous evidence of higher sympathetic drive among these individuals.
Abbreviations | ||
ACTH | = | adrenocorticotropic hormone |
AIC | = | Akaike's information criterion |
BMI | = | body mass index |
DXA | = | dual-energy absorptiometry |
FFM | = | fat-free mass |
HPAA | = | hypothalamic-pituitary-adrenal axis |
MetS | = | metabolic syndrome |
RMR | = | resting metabolic rate |
Key messages
Resting metabolic rate has been suggested to be associated with the development of obesity as well as the metabolic syndrome and might be an indirect indicator of sympathetic activity.
In this study we show that the association between birth-weight and adult resting metabolic rate differs between the sexes.
The sex-specific differences might partly be explained by differences in the developmental programming of the sympathetic nervous system between men and women.
Introduction
A small body size at birth is associated with an increased risk of the metabolic syndrome, cardiovascular disease, hypertension, and type 2 diabetes in adult life (Citation1–4). The underlying mechanisms are complex and still not fully understood. A small birth size indicates that the fetal intrauterine environment may in some way have been suboptimal. The intrauterine environment is, among other things, influenced by the mother's nutritional (Citation5), hormonal, and metabolic state. Epigenetic (Citation6), hormonal, and metabolic pathways influence the programming of organ size, metabolism, and function which in turn influences adult susceptibility to chronic disease (Citation7).
A mediating factor explaining the association between intrauterine programming and disease risk in adulthood could be the association between birth-weight and fat-free mass (FFM) in adulthood (Citation8,Citation9). Fat-free mass is primarily made up of muscle tissue, which plays an important role in the pathogenesis of type 2 diabetes and related metabolic disorders. It is well known that FFM is positively related to resting metabolic rate (RMR), an association explored in several studies (Citation10–12).
It has been proposed that a low RMR predicts weight gain both in children (Citation13) as well as among adults (Citation14,Citation15). Furthermore, a low RMR might be associated with the metabolic syndrome (Citation16). However, the overall contribution of RMR in the pathogenesis of obesity still remains unclear, with some studies (Citation17,Citation18) dismissing its role entirely. Studies of the origins of RMR in early life are few and contradictory, with results suggesting either a negative (Citation19,Citation20) or a positive (Citation21) association.
The overall goal of this study was to examine the relationship between birth size, body composition, and resting metabolic rate in adult life. Previous studies have shown that the associations between birth size and health in later life often vary according to sex (Citation22,Citation23). For this reason, one aim of the study was to explore possible sex-specific differences in the programming of resting metabolic rate.
Materials and methods
The subjects participating in this study belong to an original cohort of 8760 men and women born at the Helsinki University Central Hospital between 1934 and 1944 who attended child welfare clinics in the city and who were still resident in Finland in 1971, when each inhabitant of the country was allocated a unique identification number. Their birth records include a detailed set of measures, among them weight and length at birth and the mother's last menstrual period before giving birth, as well as maternal age, height, and weight measured at the birth hospital. Birth records have previously been described in more detail (Citation24). Being an unselected birth cohort, the study population includes healthy individuals as well as those with various degrees of disease burden.
From the original study cohort, 2902 men and women (chosen through use of random-number tables) were invited to attend a clinical examination. Of the 2003 who did so, 1918 underwent measurement of body composition, and 931 underwent indirect calorimetry. The number of subjects that underwent calorimetry was restricted by availability of equipment. The subjects for whom measurements of both body composition and calorimetry were available (n = 896; 408 men and 488 women) were included in this study.
Height was measured to the nearest 0.1 cm and weight to the nearest 0.1 kg. BMI was calculated as kg/m2. Body compostion was measured through bioelectrical impedance using the Inbody 3.0 eight-polar tactile electrode system (Biospace Co Ltd, Seoul, Korea) which estimates fat-free mass and percentage body fat through segmental impedance measurement for each limb as well as the trunk at four different electrical frequencies (5, 50, 250, and 500 kHz). The method has been validated against dual-energy absorptiometry (DXA) as well as against measurements of total body water and extracellular water by deuterium and Br dilution (Citation25–28) and has been shown to offer accurate estimates of body composition. A previous article describes the use of this method on this particular cohort in more detail (Citation29).
Resting metabolic rate, expressed as total kilocalories expended/24 hours, was determined through the use of indirect calorimetry. After an overnight fast, the Datex Deltatrac™ device (Helsinki, Finland) (Citation30) was used to measure oxygen consumption and carbon dioxide production through a transparent plastic canopy. From these results, respiratory quotient and energy expenditure were calculated.
The Deltatrac calorimeter was calibrated against a calibration gas of 96% oxygen and 4% carbon dioxide as well against ethanol burning. Both methods of calibration have been described previously in detail (Citation30). The test was recorded for 40 minutes. The first 10 minutes were eliminated as acclimatization artifact. The Deltatrac calculates RMR using the abbreviated Weir equation (Citation31). The device has been shown to offer precise measurements with low coefficients of variation, both regarding within-subject, within-measurement variation as well as within-subject, between-measurement variation (Citation32,Citation33).
Ethics and consent
Approval for the study was obtained from the Ethics Committee of Epidemiology and Public Health of the Hospital District of Helsinki and Uusimaa. All subjects provided written informed consent before any examination took place.
Statistical methods
The relationship between RMR and birth-weight was examined separately for men and women using regression analysis. Preliminary data analysis suggested a quadratic (u-shaped) relationship for men and a linear relationship for women. Therefore, a quadratic term of birth-weight was added to the model for men, which also improved the model fit in terms of Akaike's information criterion (AIC) (Citation34). The AIC criterion is a widely used measure of goodness-of-fit which properly accounts for the number of fitted parameters, thus making it suitable for comparisons between regression models with an unequal number of explanatory variables. A smaller AIC corresponds to a better fit. The models for both men and women were adjusted for age and either fat-free mass, fat mass, or both. Adjustments were made by adding the variables as covariates in the models. All analyses were done in the R environment for statistical computing (Citation35).
Results
Base-line characteristics of the 896 study participants are shown in . These characteristics were compared to the characteristics of those in the original cohort who had not undergone both calorimetry and measurement of body composition (n = 1107). No statistically significant differences were seen between the groups. Since the study population included a small number of outliers (birth-weight and BMI) the analyses were run both including and excluding individuals with a very high BMI (>40 kg/m2; n = 19), a very low birth-weight (<2 kg; n = 4), and a very high birth-weight (>5 kg; n = 2). The results remained the same regardless of inclusion or exclusion of these individuals, and therefore all 896 subjects who underwent calorimetry were included in the study.
Table I. Clinical characteristics for 408 men and 488 women participating in the study.
Body composition
Fat-free mass correlated positively with birth-weight among both sexes combined (r = 0.27; P < 0.001) as well as separately (r = 0.27; P < 0.001 for both men and women, respectively). The correlation between birth-weight and fat mass was weak but significant (r = 0.06; P < 0.01) for both sexes. When studying the association separately for men and women it remained significant for men (r = 0.15; P < 0.001) but lost significance for women (r = 0.06; P = 0.2).
The association between fat mass and FFM was stronger in women than in men (r = 0.61; P < 0.001 versus r = 0.45; P < 0.001; r = 0.52; P < 0.001 for both sexes combined, adjusted for sex).
Energy expenditure
A strong and positive correlation between fat-free mass and resting metabolic rate, expressed as kcal/24 h, was observed in the whole study population (r = 0.88; P < 0.001 for both sexes combined) as well as separately for men (r = 0.89; P < 0.001) and women (r = 0.86; P < 0.001 for women). Fat mass was also positively associated with RMR for both sexes combined (r = 0.81; P < 0.001) as well as separately (r = 0.78, P < 0.001 for men; r = 0.91, P < 0.001 for women).
Among men the relationship between age-adjusted resting metabolic rate and birth-weight was quadratic (P < 0.001), i.e. the highest values for RMR were seen in the subjects with the lowest and the highest birth-weights. For women the relationship between age-adjusted RMR and birth-weight was positive and linear (P < 0.001) (). The trends remained unchanged after further adjustment for gestational age.
Figure 1. (A) The association between age-adjusted resting metabolic rate and birth-weight for men. (B) The association between age-adjusted resting metabolic rate and birth-weight for women.
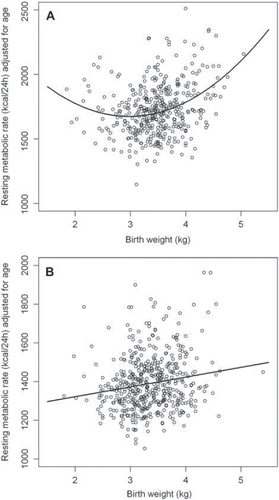
After further adjustment for either fat-free mass or fat mass, the relationship between resting metabolic rate and birth-weight remained quadratic for men (P < 0.01 after adjustment for fat-free mass; P < 0.001 after adjustment for fat mass). For women the relationship stayed positive linear after further adjustment for fat-mass (r = 0.25; P < 0.001) but became negative when instead adjusting for FFM (r = −0.12; P < 0.01). The results were not affected by further adjustment for gestational age. When age, FFM and fat mass were simultaneously included in the regression equation, the associations were no longer statistically significant for the women (P = 0.16). Among men, the association remained quadratic, albeit less significant (P = 0.05). Effect sizes and their 95% confidence intervals estimated for the final models as well as AICs are provided in , which also includes alternative models for comparison. For women, all linear models were superior to the quadratic ones regarding model fit as defined by a lower AIC. The opposite was true for the male subjects. shows the age-adjusted associations between birth-weight and RMR while shows the associations after further adjustment for FFM.
Figure 2. (A) The association between RMR adjusted for fat-free mass and age and birth-weight for men. (B) The association between RMR adjusted for fat-free mass and age and birth-weight for women.
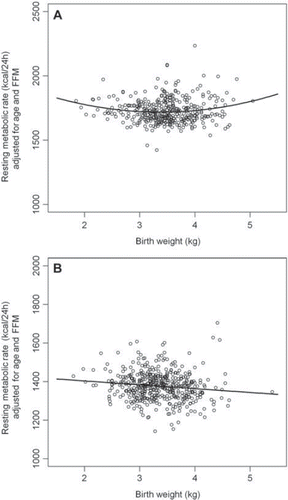
Table II. Estimated regression effects of birth-weight (kg) on RMR (kcal/24 h) adjusted for age and FM, FFM, or both. A smaller Akaike's information criterion (AIC) corresponds to a better model fit.
Discussion
In accordance with previous studies we found that a small body size at birth is associated with less fat free mass in adult life. A small body size at birth has previously been suggested to be associated with an increased resting metabolic rate (Citation20). We extended previous findings by showing that this relationship differed between the sexes. In men the association between birth size and resting metabolic rate was u-shaped while in women it was linear. This suggests that different mechanisms might program metabolism in men and women.
It is well known that birth-weight correlates positively with adult fat-free mass (Citation36,Citation37). Fat-free mass is mainly made up of muscle tissue, which, however, accounts for only 20%–30% of total RMR. Conversely, the liver, heart, brain, and kidneys contribute to 60%–70% of total RMR but account for less than 6% of total body weight (Citation38). Muscle tissue is a key player in the pathogenesis of type 2 diabetes, and the association between RMR and the amount of muscle mass could partly explain the inverse association between birth size and impaired glucose regulation in adult life. In the present study, a strong positive relationship was shown for both men and women between birth size and fat-free mass, consistent with previous studies.
As expected, we found a strong association between fat-free mass and resting metabolic rate. FFM is the most important determinant of RMR, explaining around 60%–80% of interindividual variations (Citation39,Citation40). A low RMR has been suggested to be associated with obesity and weight gain. When studying preadolescent children, individual variations in energy metabolism (including RMR) predicted up to 7% of the variance in changes in body fat percentage over a 2-year period (Citation13). When formerly obese subjects (Citation41) were studied, a correlation was observed between former obesity and a lower RMR compared to never-obese controls. This could explain the tendency for weight regain in this group. Another study (Citation15) has suggested that a low resting metabolic rate as well as a low 24-hour energy expenditure, in relation to body size and body composition, is associated with weight gain and obesity.
Fat mass also contributes to RMR, albeit to a lower degree than FFM. According to one study (Citation40), the importance of fat mass on RMR depends on the degree of adiposity, with an increasing impact up until a fat mass of 40%. At higher grades of adiposity the importance of fat mass on RMR was reduced. In the current study, fat mass was positively associated with RMR. Furthermore, FFM and fat mass were highly correlated, especially in women. Compared to other studies (Citation42), this association was surprisingly strong. Due to the strong observed association between fat mass and FFM in our cohort, it is difficult to assess how much of the association between fat mass and RMR indicates a ‘true’ metabolic influence of fat mass on RMR and how much is caused by overlapping with FFM.
It has previously been proposed that individuals with a small body mass have a higher RMR per unit FFM than those with a larger body mass. Recent studies, however, indicate that body mass does not influence specific internal organ metabolism in healthy individuals (Citation43), suggesting that a potential body mass dependency of RMR would probably have to be associated with the muscle mass compartment of FFM. A previous study from our group (Citation20) showed a negative correlation between resting metabolic rate expressed per kg of fat-free mass and birth-weight, i.e. the highest values for RMR/FFM were seen in those with the lowest birth-weights. Theoretically, this finding could imply a higher metabolic activity per unit muscle tissue in those with the lowest birth-weights, which in turn could be explained by a higher sympathetic drive. The higher RMR per unit FFM observed in those born with a low birth-weight could also explain why a small body size at birth is not associated with obesity in later life despite being associated with some obesity-related disorders e.g. type 2 diabetes, coronary heart disease, and stroke. However, the findings could also be due to a lower muscle mass in the subjects with the lowest birth-weights and a subsequently higher relative contribution from the more metabolically active compartments of FFM (i.e. internal organs) to RMR when expressed as RMR per unit FFM (Citation44).
In the present study, when comparing resting metabolic rate with birth-weight, sex-specific differences were observed. Females born with higher birth-weights had a slightly higher age-adjusted RMR compared to those born with lower birth-weights. Males showed a u-shaped association where the highest values for RMR were seen in those with the lowest and highest birth-weights. To avoid one potential source of confounding, we adjusted resting metabolic rate for fat-free mass and fat mass through regression analysis rather than studying ratios. After adjustment for fat-free mass, the linear trend for women became negative whereas the association for men remained u-shaped. When adjusting for both fat-free mass and fat mass, significance was lost for women but stayed quadratic and marginally significant for men.
In this study, we show that birth-weight predicts adult RMR in men, independently of FFM and fat mass. In women, the positive association between birth-weight and age-adjusted RMR, as well as after further adjustment for fat mass, can most likely be explained by energy contribution from the fat-free mass. When instead adjusting for FFM, a negative association between birth-weight and RMR is revealed. Based upon these findings we propose that some other factor than body composition alone influences adult resting metabolism and furthermore that this factor has its developmental origin in early life.
One possible explanation for a relatively higher adjusted RMR in those with the lowest birth-weights could be an elevated sympathetic drive among these individuals. There is indeed evidence for a direct influence of tonic sympathetic activity on RMR (Citation45). A low birth-weight has been shown to be associated with higher plasma cortisol concentrations (Citation46) and an enhanced response of plasma cortisol to adrenocorticotropic hormone (ACTH) (Citation47), findings which support the suggested relationship between low birth-weight and an increased activity in the hypothalamic-pituitary-adrenal axis (HPAA). As an indicator of an elevated sympathetic drive, previous studies (Citation48) have shown that those born with a low birth-weight have a higher resting heart rate in adult life. However, no such relationship was found in our material (data not shown). Insulin resistance and hypertension on the other hand, which can be considered as indirect indicators of an elevated sympathetic activity, are more common among individuals with the lowest birth-weights in this particular study cohort (Citation49,Citation50).
Several studies have shown sex-specific differences in the programming of the autonomic nervous system and HPAA (Citation22). If an increased sympathetic drive is indeed the reason behind the elevated RMR in those with the lowest birth-weights, the sex-specific differences (i.e. quadratic association in men versus linear in women) might be explained by differences in developmental programming of the autonomic nervous system and HPAA between men and women.
Potential weaknesses in the study design includes survivor bias, i.e. only the healthiest subjects were included in the clinical study as those with a higher morbidity had already died prior to the examination. Furthermore, the cohort is comprised of subjects born at the Helsinki University Central Hospital and might not represent the population born in Finland or even Helsinki during the period. Finally, almost all the subjects in the study were born during World War II, with the potential of elevated maternal stress levels during pregnancy (Citation51) and some degree of food shortages during childhood. Some issues regarding the methodology should also be mentioned. There is some concern that bioimpedance in certain cases might measure body fat percentage imprecisely. For example, when studying men and women from diverse racial-ethnic backgrounds, body fat percentage was under-estimated by 2.5%–3% in women when compared to a four-component model including DXA (Citation52). Consequently, some studies stress the importance of using population-specific algorithms when studying individuals from different racial-ethnic groups (Citation53,Citation54). In the present study, with subjects with homogeneous ethnicity, we chose to use the bioimpedance algorithms provided by the manufacturer. This could potentially be a weakness, as a population-specific algorithm might have resulted in more accurate results. However, bioimpedance remains a practical tool in the epidemiological setting, with haste and facility of use as compared to other methods, among them DXA.
In conclusion, we found that men with the lowest and highest birth-weights have the highest resting metabolic rates even after adjustment for fat-free mass and fat mass. In women, we found a negative linear association after adjustment for fat-free mass. The sex-specific differences for the association between birth-weight and adult RMR might theoretically be explained by differences in the developmental programming of the sympathetic nervous system between men and women. The relatively higher adjusted RMR found both among men and women with the lowest birth-weights is consistent with previous evidence of higher sympathetic drive among these individuals. A higher sympathetic drive might also be a contributor to an elevated risk of cardiovascular disease, type 2 diabetes, and hypertension in these individuals. To our knowledge, this is the first study which has shown a sex-specific difference in the association between birth-weight and adult resting metabolic rate.
Declaration of interest: Supported by the Academy of Finland, Finnish Foundation for Cardiovascular Research, Finnish Foundation for Pediatric Research, Finska Läkaresällskapet, Juho Vainio Foundation, Folkhälsan, Signe and Ane Gyllenberg Foundation, and the Yrjö Jahnsson Foundation. The authors declare no conflict of interest.
References
- Barker DJP. The Wellcome Foundation Lecture, 1994. The fetal origins of adult disease. Proc Biol Sci. 1995;262: 37–43.
- Barker DJP. Mothers, babies and health in later life. Churchill Livingstone, Edinburgh. 1998.
- Huxley RR, Shiell AW, Law CM. The role of size at birth and postnatal catch-up growth in determining systolic blood pressure: a systematic review of the literature. J Hypertens. 2000;18:815–31.
- Whincup PH, Kaye SJ, Owen CG, Huxley R, Cook DG, Anazawa S, . Birth weight and risk of type 2 diabetes: a systematic review. JAMA. 2008;300:2886–97.
- Christian P, Stewart CP. Maternal micronutrient deficiency, fetal development, and the risk of chronic disease. J Nutr. 2010;140:437–45.
- Waterland RA, Michels KB. Epigenetic epidemiology of the developmental origins hypothesis. Annu Rev Nutr. 2007; 27:363–88.
- Bruce KD, Hanson MA. The developmental origins, mechanisms, and implications of metabolic syndrome. J Nutr. 2010;140:648.
- Singhal A, Wells J, Cole TJ, Fewtrell M, Lucas A. Programming of lean body mass: a link between birth weight, obesity, and cardiovascular disease? Am J Clin Nutr. 2003;77: 726–30.
- Sayer A. Birth weight, weight at 1 y of age, and body composition in older men: findings from the Hertfordshire Cohort Study. Am J Clin Nutr. 2004;80:199–203.
- Weinsier RL, Schutz Y, Bracco D. Reexamination of the relationship of resting metabolic rate to fat-free mass and to the metabolically active components of fat-free mass in humans. Am J Clin Nutr. 1992;55:790–4.
- Wang Z, Heshka S, Gallagher D, Boozer CN, Kotler DP, Heymsfield SB. Resting energy expenditure-fat-free mass relationship: new insights provided by body composition modeling. Am J Physiol Endocrinol Metab. 2000;279: E539–45.
- Cunningham JJ. Body composition as a determinant of energy expenditure: a synthetic review and a proposed general prediction equation. Am J Clin Nutr. 1991;54:963–9.
- DeLany JP, Bray GA, Harsha DW, Volaufova J. Energy expenditure and substrate oxidation predict changes in body fat in children. Am J Clin Nutr. 2006;84:862–70.
- Tataranni PA, Harper IT, Snitker S, Del Parigi A, Vozarova B, Bunt J, . Body weight gain in free-living Pima Indians: effect of energy intake vs expenditure. Int J Obes Relat Metab Disord. 2003;27:1578–83.
- Ravussin E, Lillioja S, Knowler WC, Christin L, Freymond D, Abbott WG, . Reduced rate of energy expenditure as a risk factor for body-weight gain. N Engl J Med. 1988;318:467.
- Buscemi S, Verga S, Caimi G, Cerasola G. A low resting metabolic rate is associated with metabolic syndrome. Clin Nutr. 2007;26:806–9.
- Weinsier RL, Nelson KM, Hensrud DD, Darnell BE, Hunter GR, Schutz Y. Metabolic predictors of obesity. Contribution of resting energy expenditure, thermic effect of food, and fuel utilization to four-year weight gain of post-obese and never-obese women. J Clin Invest. 1995;95:980–5.
- Flatt JP. Differences in basal energy expenditure and obesity. Obesity (Silver Spring). 2007;15:2546–8.
- Weyer C, Pratley RE, Lindsay RS, Tataranni PA. Relationship between birth weight and body composition, energy metabolism, and sympathetic nervous system activity later in life. Obesity (Silver Spring). 2000;8:559–65.
- Eriksson J, Forsen T, Tuomilehto J, Osmond C, Barker D. Size at birth, fat-free mass and resting metabolic rate in adult life. Horm Metab Res. 2002;34:72–6.
- Kensara OA, Wooton SA, Phillips DIW, Patel M, Hoffman DJ, Jackson AA, . Substrate-energy metabolism and metabolic risk factors for cardiovascular disease in relation to fetal growth and adult body composition. Am J Physiol Endocrinol Metab. 2006;291:E365–71.
- Kajantie E, Räikkönen K. Early life predictors of the physiological stress response later in life. Neurosci Biobehav Rev. 2010;35:23–32.
- Gilbert JS, Nijland MJ. Sex differences in the developmental origins of hypertension and cardiorenal disease. Am J Physiol Regul Integr Comp Physiol. 2008;295:R1941–52.
- Eriksson J, Forsen T, Osmond C, Barker D. Obesity from cradle to grave. Int J Obes Relat Metab Disord. 2003; 27:722–7.
- Sartorio A, Malavolti M, Agosti F, Marinone PG, Caiti O, Battistini N, . Body water distribution in severe obesity and its assessment from eight-polar bioelectrical impedance analysis. Eur J Clin Nutr. 2005;59:155–60.
- Kushner RF, Kunigk A, Alspaugh M, Andronis PT, Leitch CA, Schoeller DA. Validation of bioelectrical-impedance analysis as a measurement of change in body composition in obesity. Am J Clin Nutr. 1990;52:219–23.
- Malavolti M, Mussi C, Poli M, Fantuzzi AL, Salvioli G, Battistini N, . Cross-calibration of eight-polar bioelectrical impedance analysis versus dual-energy X-ray absorptiometry for the assessment of total and appendicular body composition in healthy subjects aged 21–82 years. Ann Hum Biol. 2003;30:380–91.
- Demura S, Sato S, Kitabayashi T. Percentage of total body fat as estimated by three automatic bioelectrical impedance analyzers. J Physiol Anthropol Appl Human Sci. 2004;23: 93–9.
- Ylihärsilä H, Kajantie E, Osmond C, Forsen T, Barker DJP, Eriksson JG. Birth size, adult body composition and muscle strength in later life. Int J Obes (Lond). 2007;31:1392–9.
- Meriläinen PT. Metabolic monitor. Int J Clin Monit Comput. 1987;4:167–77.
- Weir JB. New methods for calculating metabolic rate with special reference to protein metabolism. J Physiol. 1949; 109:1–9.
- Alam DS, Hulshof PJM, Roordink D, Meltzer M, Yunus M, Salam MA, . Validity and reproducibility of resting metabolic rate measurements in rural Bangladeshi women: comparison of measurements obtained by Medgem™ and by Deltatrac™ device. Eur J Clin Nutr. 2005;59: 651–7.
- Haugen HA, Melanson EL, Tran ZV, Kearney JT, Hill JO. Variability of measured resting metabolic rate. Am J Clin Nutr. 2003;78:1141–5.
- Akaike H. Information theory and an extension of the maximum likelihood principle. Proceedings of 2nd International Symposium on Information Theory. BN Petrov, F Csaki. Akademiai Kiado. Budapest, Hungary. 1973;267–281.
- Rdevelopment C. TEAM (2007): R: A language and environment for statistical computing. R Foundation for Statistical Computing (Vienna) (http://www.R-project.org).
- Syddall HE, Aihie Sayer A, Dennison EM, Martin HJ, Barker DJP, Cooper C. Cohort profile: the Hertfordshire cohort study. Int J Epidemiol. 2005;34:1234–42.
- Gale CR, Martyn CN, Kellingray S, Eastell R, Cooper C. Intrauterine programming of adult body composition. J Clin Endocrinol Metab. 2001;86:267–72.
- Javed F, He Q, Davidson LE, Thornton JC, Albu J, Boxt L, . Brain and high metabolic rate organ mass: contributions to resting energy expenditure beyond fat-free mass. Am J Clin Nutr. 2010;91:907–12.
- Johnstone AM, Murison SD, Duncan JS, Rance KA, Speakman JR. Factors influencing variation in basal metabolic rate include fat-free mass, fat mass, age, and circulating thyroxine but not sex, circulating leptin, or triiodothyronine. Am J Clin Nutr. 2005;82:941–8.
- Bosy-Westphal A, Müller MJ, Boschmann M, Klaus S, Kreymann G, Lührmann PM, . Grade of adiposity affects the impact of fat mass on resting energy expenditure in women. Br J Nutr. 2008;101:474–7.
- Astrup A, Gotzsche PC, van de Werken K, Ranneries C, Toubro S, Raben A, . Meta-analysis of resting metabolic rate in formerly obese subjects. Am J Clin Nutr. 1999;69: 1117–22.
- Nelson KM, Weinsier RL, Long CL, Schutz Y. Prediction of resting energy expenditure from fat-free mass and fat mass. Am J Clin Nutr. 1992;56:848–56.
- Later W, Bosy-Westphal A, Hitze B, Kossel E, Gluer CC, Heller M, . No evidence of mass dependency of specific organ metabolic rate in healthy humans. Am J Clin Nutr. 2008;88:1004–9.
- Heymsfield SB, Gallagher D, Kotler DP, Wang Z, Allison DB, Heshka S. Body-size dependence of resting energy expenditure can be attributed to nonenergetic homogeneity of fat-free mass. Am J Physiol Endocrinol Metab. 2002; 282:E132–8.
- Monroe MB, Seals DR, Shapiro LF, Bell C, Johnson D, Jones PP. Direct evidence for tonic sympathetic support of resting metabolic rate in healthy adult humans. Am J Physiol Endocrinol Metab. 2001;280:E740–4.
- Phillips DIW, Walker BR, Reynolds RM, Flanagan DEH, Wood PJ, Osmond C, . Low birth weight predicts elevated plasma cortisol concentrations in adults from 3 populations. Hypertension. 2000;35:1301.
- Reynolds RM, Walker BR, Syddall HE, Andrew R, Wood PJ, Whorwood CB, . Altered control of cortisol secretion in adult men with low birth weight and cardiovascular risk factors. J Clin Endocrinol Metab. 2001;86:245–50.
- Phillips DIW, Barker DJP. Association between low birthweight and high resting pulse in adult life: is the sympathetic nervous system involved in programming the insulin resistance syndrome? Diabet Med. 1997;14:673–7.
- Eriksson JG, Osmond C, Kajantie E, Forsen TJ, Barker DJP. Patterns of growth among children who later develop type 2 diabetes or its risk factors. Diabetologia. 2006;49: 2853–8.
- Barker DJP, Forsen T, Eriksson JG, Osmond C. Growth and living conditions in childhood and hypertension in adult life: a longitudinal study. J Hypertens. 2002;20:1951–6.
- Schreier N, Moltchanova E, Blomstedt P, Kajantie E, Eriksson J. Prenatal exposure to wartime stress—long-term effect on coronary heart disease in later life. Ann Med. 2010 Oct 26 (Epub ahead of print).
- Gibson AL, Holmes JC, Desautels RL, Edmonds LB, Nuudi L. Ability of new octapolar bioimpedance spectroscopy analyzers to predict 4-component-model percentage body fat in Hispanic, black, and white adults. Am J Clin Nutr. 2008;87:332–8.
- Dehghan M, Merchant AT. Is bioelectrical impedance accurate for use in large epidemiological studies? Nutr J. 2008;7:26.
- Aleman-Mateo H, Rush E, Esparza-Romero J, Ferriolli E, Ramirez-Zea M, Bour A, . Prediction of fat-free mass by bioelectrical impedance analysis in older adults from developing countries: A cross-validation study using the deuterium dilution method. J Nutr Health Aging. 2010;14:418–26.