Abstract
Introduction. Insulin metabolism has been previously linked to oxidized low-density lipoproteins (ox-LDL), but corroborating intervention studies are lacking. We investigated whether changes in ox-LDL levels are accompanied by changes in insulin sensitivity in a 32-month life-style intervention study.
Materials and methods. A 2-month weight reduction was followed by 6-month diet and exercise counselling and a 2-year follow-up period. Men of 35–50 years of age, BMI ≥ 30 kg/m2, and waist circumference > 100 cm were recruited via newspapers in the city of Tampere, Finland. Of the 90 men meeting the inclusion criteria, 67 (76%) completed the study. Ox-LDL was estimated as the presence of oxidized lipids in LDL. Homeostasis model assessment of insulin resistance (HOMA-IR), ox-LDL, and ratio of ox-LDL and high-density lipoprotein cholesterol (ox-LDL/HDL-c) were used as the main outcome measures.
Results. The detected changes in HOMA-IR were strikingly similar to those in ox-LDL and ox-LDL/HDL-c. Compared to the first HOMA-IR quartile, the fourth quartile had 23%–51% higher concentrations in ox-LDL and ox-LDL/HDL-c at all time points (P < 0.05 for all).
Conclusion. This weight reduction intervention study adds evidence to support the connection between insulin metabolism and oxidized LDL, possibly contributing to the higher incidence of atherosclerotic cardiovascular diseases among diabetic patients.
While some previous data suggest that insulin action and oxidation of LDL lipids may be associated, there is a lack of intervention studies.
This 32-month intervention plus follow-up study in middle-aged men shows that levels of ox-LDL and ox-LDL/HDL-c are consistently and strongly connected to impaired insulin sensitivity measured by the homeostasis model assessment of insulin resistance (HOMA-IR).
These findings further illuminate the increased atherosclerosis risk mechanisms characteristic of diabetes and the contributing effect thereon of atherogenic (oxidative) modifications in LDL lipids.
Introduction
Atherosclerosis is a multidimensional entity, in which plasma lipids constitute an outstanding key factor. Diabetic individuals have been shown to have significantly worse lipid profiles than non-diabetics (Citation1). This higher risk for cardiovascular diseases (CVD) related to diabetes has been an impetus to explore the role of insulin metabolism in vascular health.
There is an increasing body of evidence to substantiate the important role of oxidized low-density lipoproteins (ox-LDL) in the development of atherosclerosis (Citation2). It has been observed that arterial foam cell formation, characteristic of atherosclerosis, is triggered by receptor-mediated recognition of modified LDL particles by macrophages. The recognition site is on the lipid compartment of LDL particles (Citation3).
CD36 is a class B scavenger receptor which is known to play a central role in ox-LDL-initiated foam cell formation in atherogenesis (Citation4,Citation5). Recent studies have shown that the CD36-mediated pathophysiological effects of ox-LDL are not limited to atherogenesis, but are also implicated in impaired insulin response (Citation6,Citation7). Interestingly, the plasma level of oxidized LDL lipids is directly related to CD36 expression in subjects with type 2 diabetes (Citation8). Considering the previous data, it seems reasonable to assume that circulating oxidized LDL lipids may contribute to this CD36-mediated biological response in vivo.
We have previously shown that the serum concentration of oxidized LDL lipids is elevated in obese subjects and can be lowered by weight reduction (Citation9). The connection between LDL oxidation and insulin metabolism is a topic in need of results from prospective intervention studies with follow-up (Citation10). We hypothesized that changes in oxidized LDL lipids would be reflected in changes in insulin resistance. Therefore, in the present life-style intervention study we investigated whether changes in oxidized LDL lipid levels are accompanied by changes in insulin sensitivity after weight reduction, weight maintenance, and 2-year follow-up among obese, middle-aged male subjects.
Materials and methods
Subjects
Study subjects were recruited via newspaper advertisements in the city of Tampere, Finland. The inclusion criteria were male sex, age 35–50 years, body mass index (BMI, weight divided by the height squared) 30–40 kg m− 2, and waist circumference over 100 cm. A total of 214 men responded. Subjects with regular medication, physical activity ≥ 1 per week, smoking habit, or suspected binge eating disorder were excluded. Finally, 90 men met the inclusion criteria. Of these, 67 men (76%) completed the study. For the 23 men not completing the study, lack of time or interest was the main reason for dropping out; none dropped out due to serious illness or injury related to the intervention programme.
Experimental design
The study design has been published earlier (Citation11). In brief, the duration of the study was 32 months and consisted of three phases: weight reduction, weight maintenance, and unsupervised follow-up. The first phase, the weight reduction phase (WRP), lasted for 2 months when the subjects reduced weight by very-low-energy diet (VLED, 2 MJ a day; Nutrilett®, Leiras Oy, Turku, Finland). During WRP, the men met in small groups led by a trained nutritionist. The WRP was followed by a 6-month life-style counselling period of weight maintenance (WMP), including diet and exercise. During WMP, men were randomized into exercise and control groups. In the exercise groups, a supervised session of 45 min three times a week was carried out (Citation11). Subjects in the control group were instructed not to increase the level of physical activity. After the WMP came a 2-year unsupervised follow-up period. Assessments of serum lipoproteins and anthropometry were carried out on five occasions: at baseline (0 months), after weight reduction (2 months), after the weight maintenance period (8 months), and after the first (20 months) and second follow-up year (32 months). All participants gave their written informed consent. The Research Ethics Committee of the UKK Institute approved of the study. This study conforms to the principles outlined in the Declaration of Helsinki.
Laboratory measurements
Blood glucose was measured from fresh venous samples between 08.00 and 09.00 after a 12-h fast. Plasma glucose was assessed by the glucose dehydrogenase method (Merck Diagnostica, Darmstadt, Germany). Plasma insulin determinations were done by radioimmunoassay (Phadeseph Insulin; Pharmacia, Uppsala, Sweden). Serum concentrations of cholesterol and triglycerides (TG) were analysed by enzymatic methods (CHOL-PAP for cholesterol and GPO-PAP for TG; Boehringer Mannheim, Mannheim, Germany). High-density lipoprotein cholesterol (HDL-c) was measured by selective precipitation (dextran sulfate) (Citation12).
Oxidized LDL lipids
The assay was based on analysis of oxidized lipids in isolated LDL (Citation13,Citation14). LDL was isolated by precipitation with buffered heparin. The isolation procedure was validated for the purpose and did not affect the level of oxidized lipids (Citation13). Lipids were extracted from isolated LDL by chloroform-methanol, dried under nitrogen, and redissolved in cyclohexane. The amount of peroxidized lipids in LDL was assessed spectrophotometrically at 234 nm. Validation studies for the assay have ruled out interference by non-specific substances and shown that the assay is a measure of oxidative modifications found in all LDL lipid classes. The coefficient of variation (CV) for within-assay precision for determination of oxidized LDL lipids was 4.4%, and the CV for the between-assay precision was 4.5%. The isolated LDL fraction was used for direct measurement of LDL-cholesterol.
Insulin resistance
For estimation of the degree of insulin resistance we used the homeostasis model assessment method (HOMA-IR). This measure shows strong associations to the traditional clamp techniques and has considerable practical advantages (Citation15). In this study protocol, altogether five measurements were collected (see above, under ‘Experimental design’), decreasing the acknowledged challenges of interpretations made based on a single measurement (Citation16).
Statistical methods
For statistical analyses we used Statistical Package for Social Sciences (SPSS) versions 14.0 and 22.0. We used logarithmically transformed values of the variables that had significantly skewed distribution. The differences between first and fourth quartiles at each time point were analysed by independent samples t test. Partial correlation coefficients were calculated to demonstrate further possible (independent) associations between parameters of ox-LDL and HOMA-IR, simultaneously controlling for potential confounding effects of changes in waist and BMI. A P value of < 0.05 was considered as statistically significant.
Results
When the participants were divided by quartiles of HOMA-IR values in each measurement, during WRP, mean (SD) weight reductions in the first and fourth HOMA quartiles were 15.7 (3.1) and 12.6 (4.4) kg, respectively. Corresponding changes in waist circumference were 17.2 (5.6) and 15.7 (4.2) cm, respectively. During follow-up, regains were 4.9 (5.5) and 14.0 (5.7) kg in weight, and 5.9 (6.1) and 13.5 (6.8) cm in waist (). Throughout the study, the detected changes in HOMA-IR appeared strikingly similar to those in ox-LDL and ox-LDL/HDL-c seen in these two quartiles ( and ). In comparison to the first quartile, the fourth quartile had 23% to 37% higher concentrations of ox-LDL throughout the study (P < 0.05 for all; ). Likewise, the ox-LDL/HDL-c ratio was 27% to 51% higher in the fourth quartile at all measurement points (P < 0.05 for all; ).
Figure 1. Oxidized LDL (A) and oxidized LDL/HDL-c (B) in the first and fourth quartiles of HOMA-IR during a 32-month intervention study in middle-aged, originally obese men.
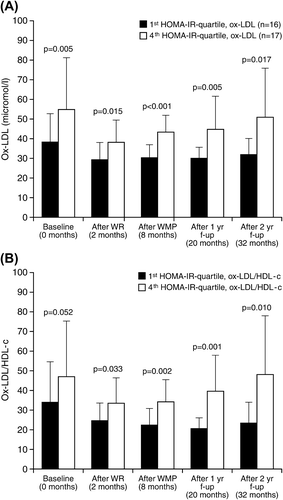
Table I. Means (SD) of study variables according to first and fourth HOMA quartiles at each time point during the 32-month lifestyle intervention study.
To establish a more thorough metabolic context, indicates the measurements in conventional blood lipids, anthropometric measures, and HOMA-IR cut-offs in the first and fourth quartiles of HOMA-IR.
The main results of the correlation data are given in , showing associations between HOMA-IR and ox-LDL parameters. The correlation analyses showed that concentrations of both ox-LDL and ox-LDL/HDL-c associated significantly with both fasting insulin and HOMA-IR levels in each of the five sampling times (ρ = 0.304–0.550, P < 0.05 for ox-LDL; ρ = 0.274–0.538, P < 0.05 for ox-LDL/HDL-c, ). The changes in ox-LDL and ox-LDL/HDL-c during follow-up correlated with the corresponding change in HOMA-IR (ρ = 0.330, P = 0.007; ρ = 0.424, P < 0.001 respectively) and insulin (ρ = 0.349, P = 0.004; ρ = 0.455, P < 0.001, respectively), but not with fasting glucose. Even after controlling for changes in BMI and waist during the 2.5-year follow-up, both ox-LDL and ox-LDL/HDL-c correlated with both insulin (ρ = 0.289, P = 0.021; ρ = 0.382, P = 0.002, respectively) and HOMA-IR (ρ = 0.277, P = 0.026; ρ = 0.365, P = 0.003, respectively), but not with fasting glucose.
Table II. Pearson correlations between HOMA-IR and ox-LDL parameters at each time point during the study.
Discussion
In this study, measures of ox-LDL were significantly connected to HOMA-IR throughout the intervention and follow-up study protocol. The highest HOMA-IR quartile consistently had the highest concentrations of ox-LDL and ox-LDL/HDL-c. As expected, the other more conventional lipid parameters showed a similar trend. These results indicate a strong association between HOMA-IR, ox-LDL, and ox-LDL/HDL-c, further highlighting the association between insulin metabolism and oxidative damage in lipids. Additionally, as changes in fasting glucose did not correlate with corresponding changes in ox-LDL or ox-LDL/HDL-c, whereas respective correlations for fasting insulin did, the importance of insulin in the homeostasis equation is highlighted. Therefore, from the perspective of atherogenic modifications in LDL composition, indicated as oxidation in the lipid compartment of LDL, these findings suggest that insulin is more crucial than glucose. The foundation for the study was the acknowledged lack of intervention plus follow-up studies addressing the potential connection between insulin metabolism and oxidized LDL (Citation10), and between modifiable, novel cardiovascular risk factors and weight management (Citation17). The clinical implications of this study suggest a mechanism partly explaining the increased atherosclerosis risk in diabetic patients.
It was found earlier in a population-based observational study that evolving insulin resistance (HOMA-IR) increases with rising level of ox-LDL (Citation18). In accordance, Rector et al. (Citation19) reported that aerobic exercise training, accompanied by a modest energy restriction, decreased both ox-LDL and insulin resistance among sedentary overweight and obese subjects. In the above studies the measurement of ox-LDL was based on detection of modified lysine residues in apolipoprotein B, which is known to be secondary to LDL oxidation (Citation18,Citation19). In the present study the determination of ox-LDL was based on analysis of oxidized lipids in LDL. Reverse-phase high-performance liquid chromatography (HPLC) and electrospray ionization-mass spectrometry analyses have demonstrated that these modified LDL lipids consist of hydroxyl-, epoxy-, and keto-derivatives of fatty acids, and of triacylglycerol core aldehydes (Citation20). It is noteworthy that, of the constituents of LDL particles, oxidized lipids are known to be the crucial species for the recognition and binding of ox-LDL by the CD36 receptor (Citation3).
Oxidized lipids in LDL are well known to have widespread biological effects. In particular, the association between LDL oxidized lipids and atherosclerosis is strong. LDL oxidized lipids are involved in activation of atherosclerosis-related gene groups (Citation21) and in macrophage accumulation and regulation of their activity and foam cell formation in vessel walls (Citation22). Oxidized lipids in circulating LDL are strongly associated with coronary, carotid, and brachial atherosclerosis, hypertension, and arterial functions (Citation14), and serum concentrations of LDL oxidized lipids can be reduced by the common risk management programmes of cardiovascular diseases (Citation14).
In previous studies we have shown that weight reduction among overweight and obese adults results in decreased concentrations of LDL oxidized lipids, and that regain of weight after the supervised weight reduction period is accompanied by re-elevation of the oxidized LDL lipid levels (Citation9,Citation23). A 10-month exercise programme resulting in 2–3 kg weight reduction is also demonstrated to decrease the concentration of LDL oxidized lipids (Citation24). These results together with the studies using different analytical methods for measuring ox-LDL (Citation18,Citation19) underline the potential of life-style intervention utilizing weight loss and physical activity to improve insulin sensitivity and to decrease ox-LDL. Therefore, the supervised weight reduction, followed by the weight maintenance and long-term follow-up periods, offered a physiological study model for investigation of the interdependence between LDL oxidized lipids and insulin resistance.
In the fasting state, the level of hyperglycaemia is reflective of both β-cell dysfunction in the pancreas and insulin resistance (Citation15). This is the basis of HOMA-IR calculation. Unfortunately, its coefficient of variation has traditionally been relatively high, but inexpensiveness and strong associations with clamp techniques are substantial benefits. The biological representativeness of a single measurement is challenged, while repetition may improve its reliability. Some previous life-style intervention studies have shown encouraging results utilizing HOMA (Citation19,Citation25). From these perspectives, we decided to use HOMA-IR as the measurement indicating insulin resistance in our study design, involving five measurements during a combination of intervention and follow-up.
We found in the present study that both the decrease and the re-elevation of oxidized LDL lipid concentrations during WRP and follow-up periods were accompanied by corresponding changes in the HOMA-IR values. The concentrations of LDL oxidized lipids correlated with fasting insulin and HOMA-IR throughout the whole study period, and changes in ox-LDL and ox-LDL/HDL-c during the follow-up correlated with fasting insulin and HOMA-IR even after controlling for BMI and waist. These findings give strong support to the anticipated role of ox-LDL in insulin metabolism. The biological mechanism(s) connecting ox-LDL with insulin metabolism could not be studied in this intervention study. It has been reported earlier that lipid peroxides induce structural changes in human insulin, thereby disturbing insulin function (Citation26). Moreover, it is known that ox-LDL is able to disrupt insulin signalling through activation of CD36-dependent signalling pathways (Citation6,Citation7).
In addition to the total amount of LDL oxidized lipids, also the ratio ox-LDL/HDL-c showed very strong association with insulin resistance at all study points. During the follow-up period of the study the correlation of ox-LDL/HDL-c with fasting insulin and HOMA-IR was even stronger than that of ox-LDL. This finding is in agreement with recent studies showing that HDL particles play an active role not only in the reverse transport of cholesterol but also in sequestration and transport of products of lipid peroxidation (Citation27). In a recent population-based survival study among aged individuals we found that ox-LDL, when proportioned to HDL-c, was a significant predictor of mortality, even after controlling for age, sex, BMI, smoking, and diabetes, while LDL cholesterol and apoB had no predictive value (Citation28).
We have previously shown that food lipid peroxides are absorbed and incorporated into serum lipoproteins, and that the concentration of LDL oxidized lipids may substantially increase after a fatty meal (Citation27). Together with the data of the present study, this further stresses the central roles of dietary factors and lipoproteins in insulin resistance and other CD36-mediated pathophysiological processes. In support of this, it has been shown that diet rich in lipid peroxides induces insulin resistance and impairs insulin secretion (Citation29).
It is noteworthy that at the end of this study, two-and-a-half years after the weight reduction period, the first and fourth quartiles of HOMA-IR discriminate men with a 15 cm difference in waist girth (99 versus 114 cm, ). Currently, abdominal obesity (waist circumference > 100 cm in men) is acknowledged as a metabolically burdening condition (Citation30). In our study, the first HOMA-IR quartile consistently describes subjects who after WRP showed a waist girth measure below 100 cm. Consequentially, by this definition, the fourth HOMA-IR quartile demonstrated abdominal obesity throughout the study protocol. The findings of this study indicate that the metabolic burden of abdominal obesity involves not only compromised insulin sensitivity, but—consistently—also high concentrations of oxidized LDL lipids.
In conclusion, there was a significant co-existence of high levels of ox-LDL, ox-LDL/HDL-c, and insulin resistance throughout a 32-month intervention plus follow-up study in middle-aged obese men. These findings may improve our understanding of the increased atherosclerosis risk involved in diabetes and promote the importance of successful life-style changes therein.
Funding: This work was supported by Turku University Foundation, Juho Vainio Foundation, Aarne Koskelo Foundation, Finnish Foundation for Cardiovascular Research and Emil and Bilda Maunula Foundation.
Declaration of interest: The authors report no conflicts of interest.
References
- Kopprasch S, Pietzsch J, Kuhlisch E, Fuecker K, Temelkova-Kurktschiev T, Hanefeld M, et al. In vivo evidence for increased oxidation of circulating LDL in impaired glucose tolerance. Diabetes. 2002;51:3102–6.
- Stocker R, Keaney JF Jr. Role of oxidative modifications in atherosclerosis. Physiol Rev. 2004;84:1381–478.
- Nicholson AC, Frieda S, Pearce A, Silverstein RL. Oxidized LDL binds to CD36 on human monocyte-derived macrophages and transfected cell lines. Evidence implicating the lipid moiety of the lipoprotein as the binding site. Arterioscler Thromb Vasc Biol. 1995;15:269–75.
- Silverstein RL, Febbraio M. CD36 and atherosclerosis. Curr Opin Lipidol. 2000;11:483–91.
- Silverstein RL, Li W, Park YM, Rahaman SO. Mechanisms of cell signaling by the scavenger receptor CD36: implications in atherosclerosis and thrombosis. Trans Am Clin Climatol Assoc. 2010;121:206–20.
- Scazzocchio B, Varì R, D’Archivio M, Santangelo C, Filesi C, Giovannini C, et al. Oxidized LDL impair adipocyte response to insulin by activating serine/threonine kinases. J Lipid Res. 2009;50:832–45.
- Kennedy DJ, Kuchibhotla S, Westfall KM, Silverstein RL, Morton RE, Febbraio M. A CD36-dependent pathway enhances macrophage and adipose tissue inflammation and impairs insulin signaling. Cardiovasc Res. 2011;89:604–13.
- Sampson MJ, Davies IR, Braschi S, Ivory K, Hughes DA. Increased expression of scavenger receptor (CD36) in monocytes from subjects with type 2 diabetes. Atherosclerosis. 2003;167:129–34.
- Vasankari T, Fogelholm M, Kukkonen-Harjula K, Nenonen A, Kujala U, Oja P, et al. Reduced oxidized low-density lipoprotein after weight reduction in obese premenopausal women. Int J Obes Relat Metab Disord. 2001;25:205–11.
- Holvoet P, De Keyzer D, Jacobs DR Jr. Oxidized LDL and the metabolic syndrome. Future Lipidol. 2008;3:637–49.
- Borg P, Kukkonen-Harjula K, Fogelholm M, Pasanen M. Effects of walking or resistance training on weight loss maintenance in obese, middle-aged men: a randomized trial. Int J Obes Relat Metab Disord. 2002;26:676–83.
- Nguyen T, Warnick GR. Improved methods of total HDL-c and subclasses. Clin Chem. 1989;35:1086.
- Ahotupa M, Marniemi J, Lehtimäki T, Talvinen K, Raitakari OT, Vasankari T, et al. Baseline diene conjugation in LDL lipids as a direct measure of in vivo LDL oxidation. Clin Biochem. 1998;31:257–61.
- Ahotupa M, Vasankari TJ. Baseline diene conjugation in LDL lipids: an indicator of circulating oxidized LDL. Free Radic Biol Med. 1999:27:1141–50.
- Matthews DR, Hosker JP, Rudenski AS, Naylor BA, Treacher DF, Turner RC. Homeostasis model assessment: insulin resistance and beta-cell function from fasting plasma glucose and insulin concentrations in man. Diabetologia. 1985;28:412–19.
- Borai A, Livingstone C, Ferns GAA. The biochemical assessment of insulin resistance. Ann Clin Biochem. 2007;44:324–42.
- Tzotzas T, Evangelou P, Kiortsis DN. Obesity, weight loss and conditional cardiovascular risk factors. Obes Rev. 2011;12:e282–9.
- Park K, Gross M, Lee D-H, Holvoet P, Himes JH, Shikany JM, et al. Oxidative stress and insulin resistance. Diabetes Care. 2009;32:1302–7.
- Rector RS, Warner SO, Liu Y, Hinton PS, Sun GY, Cox RH, et al. Exercise and diet induced weight loss improves measures of oxidative stress and insulin sensitivity in adults with characteristics of the metabolic syndrome. Am J Physiol Endocrinol Metab. 2007;293:E500–6.
- Suomela J-P, Ahotupa M, Sjövall O, Kurvinen J-P, Kallio H. New approach to the analysis of oxidized triacylglycerols in lipoproteins. Lipids. 2004;39:507–12.
- Furnkranz A, Schober A, Bochkov VN, Bashtrykov P, Kronke G, Kadl A, et al. Oxidized phospholipids trigger atherogenic inflammation in murine arteries. Arterioscler Thromb Vasc Biol. 2005;25:633–8.
- Birukov KG. Oxidized lipids: the two faces of vascular inflammation. Curr Atheroscler Rep. 2006;8:223–31.
- Raitakari M, Ilvonen T, Ahotupa M, Lehtimäki T, Harmoinen A, Suominen P, et al. Weight reduction with very-low caloric diet and endothelial function in overweight adults. Role of plasma glucose. Arterioscler Thromb Vasc Biol. 2004;24:124–8.
- Vasankari TJ, Kujala U, Vasankari TM, Ahotupa M. Reduced oxidized LDL levels after a 10-month exercise program. Med Sci Sports Exerc. 1998;30:1496–501.
- Bhat G, Baba CS, Pandey A, Kumari N, Chourhuri G. Lifestyle modification improves insulin resistance and liver histology in patients with non-alcoholic fatty liver disease. World J Hepatol. 2012;4:209–17.
- Pillon NJ, Vella RE, Soulère L, Becchi M, Lagarde M, Soulage CO. Structural and functional changes in human insulin induced by lipid peroxidation byproducts 4-hydroxy-2-nonenal and 4-hydroxy- 2-hexenal. Chem Res Toxicol. 2011;24:752–62.
- Ahotupa M, Suomela JP, Vuorimaa T, Vasankari T. Lipoprotein-specific transport of circulating lipid peroxides. Ann Med. 2010;42:521–9.
- Linna M, Ahotupa M, Löppönen MK, Irjala K, Vasankari T. Circulating oxidized LDL lipids, when proportioned to HDL-c, emerged as a risk factor of all-cause mortality in a population-based survival study. Age Ageing. 2013;42:110–13.
- Tsujinaka K, Nakamura T, Maegawa H, Fujimiya M, Nishio Y, Kudo M, et al. Diet high in lipid hydroperoxide by vitamin E deficiency induces insulin resistance and impaired insulin secretion in normal rats. Diabetes Res Clin Pract. 2005;67:99–109.
- Huxley R, Mendis S, Zheleznyakov E, Reddy S, Chan J. Body mass index, waist circumference and waisth:hip ratio as predictors of cardiovascular risk – a review of the literature. Eur J Clin Nutr. 2010; 64:16–22.