Abstract
Background and purpose. MicroRNAs (miRNAs) are short, non-coding RNAs whose deregulation has been shown in several human diseases, including pain states and diseases associated with increased cardiovascular (CV) risk. This study aimed at identifying differentially expressed circulating miRNAs in patients with ‘migraine without aura’ (MO), a pain condition whose link with CV risk remains debated.
Methods. Fifteen female MO patients and 13 matching healthy controls underwent a circulating microRNA expression profiling. MiR-22, miR-26a, miR-26b, miR-27b, miR-29b, let-7b, miR-181a, miR-221, miR-30b, and miR-30e were selected for validation by quantitative real-time polymerase chain reaction.
Results. In migraineurs versus controls, four miRNAs were differentially expressed: miR-27b was significantly up-regulated (q < 0.004), while miR-181a, let-7b, and miR-22 were significantly down-regulated (q ≤ 0.01). MiR-22 and let-7b down-regulation was also confirmed in circulating blood monocytes. A logistic regression model based on microRNA expression profile showed a high accuracy for identifying migraine (AUC of ROC curve: 0.956; P < 0.001).
Conclusion. A specific circulating miRNAs profile is associated with migraine without aura. Remarkably, the same miRNAs are known to be modulated in the setting of atherosclerosis and stroke in humans. This study represents a first step towards further characterization of MO diagnosis/pathophysiology, also in relation to its link with cardiovascular risk.
Migraine without aura (MO) is associated with changes in circulating microRNAs.
MiR-181a, let-7b, miR-27b, and miR-22, which are modulated in MO, are known to be involved in important pathways for cardiovascular disease development.
Evaluation of microRNA expression could represent a new tool for diagnostic/pathophysiologic characterization of different forms of migraine also in relation to the relative associated CV risk.
Introduction
Migraine is a highly frequent neurological disorder (1-year prevalence: 18% in women and 6% in men) and one of the most intense and disabling forms of pain that a human being can experience (Citation1). In spite of this epidemiological relevance, its pathophysiology still remains incompletely known, which results in a lack of objective markers of the disease, with diagnosis being based exclusively on clinical criteria (Citation2), and an often unsatisfactory pharmacologic control of the symptoms due to scarce availability of mechanism-based compounds (Citation3). The impact of the migraine status on patients’ health may furthermore be complicated by the increased cardiovascular risk that has been reported by many studies as being significantly associated with the condition. Results of three meta-analyses of observational studies showed, for instance, that patients with migraine have a 2-fold augmented risk of ischemic stroke; the risk seems to increase with increasing migraine attack frequency, and women and younger age groups are particularly affected (Citation4,Citation5). Although most data regard the association of cardiovascular (CV) risk with migraine with aura (MA), several studies also suggest a possible increased CV risk in migraine without aura (MO), which is of particular clinical relevance considering that MO has a much higher incidence in the general population (two-thirds of migraine patients) and is also characterized by a higher migraine attack frequency than MA (Citation6). To date, however, the actual relationship between this form of migraine and the increased CV risk remains debated and elusive. On this basis, the aim of our study was to search for objective markers of migraine without aura, with particular attention on factors that are also of relevance for cardiovascular diseases. Since male sex is regarded as an independent risk factor for CV risk, in this preliminary study we restricted our evaluation to female patients, who also represent the vast majority of the migraine population (Citation7).
MicroRNAs (miRNAs) are short, non-coding RNAs that represent a powerful class of negative post-transcriptional modulators of gene expression (Citation8). Although miRNAs are expressed by every cell, some of them are preferentially found in specific cell types. For example, in vessel walls miR-126 is almost exclusively expressed by endothelial cells—and not by vascular smooth muscle cells—resulting in important consequences in terms of final target genes expression in the different cell types (Citation9). By controlling the expression levels of several key biological targets, miRNAs have been shown to play crucial roles in human diseases ranging from cancer to inflammatory diseases, cardiovascular diseases, and atherosclerosis (Citation8,Citation10–12).
Despite their intracellular production, miRNAs are released in the extracellular space and show a remarkable stability in the blood-stream, raising the possibility that circulating miRNAs could serve as potential biomarkers in human diseases (Citation11,Citation13). In particular, being regulators of key processes in the nervous and inflammatory systems, miRNAs are likely to be involved in pain signalling. Studies in different animal models have indeed shown that pain might influence miRNA expression in primary afferent nociceptors, and deregulation of miRNAs could modulate local inflammation and nociceptor sensitization (Citation14). Furthermore, dysregulation of miRNAs has been found in patients with complex regional pain syndrome, a neuropathic condition also associated with a specific inflammatory profile (Citation15,Citation16), in osteoarthritis pain, and in fibromyalgia (Citation17–19). Finally, miRNAs have been associated with ischemic stroke in humans (Citation8,Citation10,Citation20–22). At this time, however, no evidence is available about the expression and the role of miRNAs selectively in the pain condition of ‘migraine without aura’ (MO). Thus, in the present study, we performed a profiling of circulating miRNAs in MO patients compared to healthy controls as a first step towards identification of new biomarkers of this form of migraine.
Materials and methods
Study population
We enrolled 15 female patients (aged 34.9 ± SD 10.7 years) with a diagnosis of migraine without aura made by a headache specialist according to the International Headache Society criteria at least 5 years before the start of the study (last criteria published at the time of the diagnosis, then confirmed by the 2013 beta revision criteria) (Citation23). Due to the analytical challenges of microRNA profiling and the lack of evidence in the literature on the expression levels of microRNAs in exosomes of migraineurs, the a priori evaluation of the sample size was not easily possible. For this reason, we empirically enrolled 15 patients and 13 controls. A post hoc analysis of statistical power based on the data relative to microRNA expression in healthy controls revealed that our population was able to provide on average a discrete power (> 80%) for detecting 2.5-fold changes in microRNA expression. All the patients had a number of monthly attacks ranging between 3 and 6 in the past 6 months; no prophylactic migraine treatment in the past 3 months; absence of any concomitant disease or treatment; a negative urine pregnancy test. A balanced control group of 13 female subjects (aged 31.7 ± 2.9 years) was considered. The age difference between the migraine and control groups was not significant.
All participants but one were in their fertile phase of life. A positive familial history of headache was present in all patients except one, and patients had been suffering from migraine for 17.3 ± 10 years. The mean monthly number of attacks in the past 6 months was 4.1 ± 1.2. All the subjects gave their informed written consent for inclusion in the study.
Blood sampling, exosome isolation, and RNA extraction
All subjects underwent venous blood sampling in the same relative phase of the menstrual cycle (follicular phase) to minimize inter-subject variability due to hormonal fluctuations (Citation24). In patients, care was taken to collect the venous sample at least 72 hours after any symptomatic drug assumption for a migraine attack. Although circulating microRNAs in the blood-stream could be found both in vesicle (including exosomes) and in vesicle-free form, in our study we specifically investigated the microRNA profile of exosomes isolated from plasma, as studies propose exosomes as vehicles for the miRNA-based paracrine (and potentially endocrine) communication system (Citation11,Citation13,Citation25,Citation26). Exosomes were isolated and RNA extracted as previously described (Citation27). Briefly, 500 μL of plasma were taken from each sample. ExoQuick exosome precipitation solution (System Biosciences, Mountain View, CA, USA) (126 μL) was added to plasma to precipitate an exosome pellet, as described by the manufacturer. This exosome pellet was then treated as a normal cell pellet and lysed in 300 μL of RNeasy Lysis Buffer RLT (Qiagen, Hilden, Germany); RNA extraction was then performed using a mixture of trizol (Invitrogen) and carrier (MS2 phage carrier (Roche Applied Science, Indianapolis, IN, USA)), according to the manufacturer's instructions. The RNA pellet was washed in ethanol 75% and then re-suspended in 10 μL of DNAse/RNase-free water.
Peripheral blood monocyte isolation and RNA extraction
Peripheral blood mononuclear cells (PBMCs) were obtained by density gradient centrifugation using Ficoll-Paque Plus (GE Healthcare Europe, Freiburg, Germany). Total RNA enriched for small RNAs was isolated using the trizol (Invitrogen, Carlsbad, CA, USA) according to the manufacturer's protocol. Total RNA concentration and purity were controlled by UV spectrophotometry (A260 / A280 < 2.0) using a NanoDrop ND 1000 (ThermoScientific, Wilmington, DE, USA). The quality of RNAs was determined using 1.5% agarose gel.
Evaluation of microRNAs levels by real-time polymerase chain reaction (qRT-PCR)
Evaluation of microRNAs was performed as previously described (Citation27). For the microarray analysis, 2 μL of RNA extracted from exosomes from the subjects’ samples were pooled in order to obtain two homogeneous samples (‘migraine’ and ‘control’). After reverse transcription carried out using the mirCURY LNATM Universal cDNA synthesis kit (Exiqon, Copenhagen, Denmark), the reactions were performed on a Serum/Plasma Focus microRNA PCR Panel 384 well (V1) (Exiqon) according to the manufacturer's protocol using the ABI 7900HT (Applied Biosystems, Foster City, CA, USA). The microRNAs evaluated in the screening procedure are listed in Supplementary Table I to be found online at http://informahealthcare.com/doi/abs/10.3109/07853890.2015.1071871. After this first screening, the levels of selected microRNAs (let-7b, miR-22, miR-26a, miR-26b, miR-27b, miR-29b, miR-30b, miR-30e, miR-181a, and miR-221) were assessed by qRT-PCR on each individual sample. For each sample, a fixed volume of 4 μL of RNA was used in reverse transcription with mirCURY LNATM Universal cDNA synthesis kit (Exiqon). Each qRT-PCR was performed at least in duplicate on every individual sample.
MicroRNAs profiling data were analysed using GenEx (Exiqon GenEx Pro, Academic) in order to set a more robust data normalization, incorporating both NormFinder and GeNorm software. MiR-103 and miR-93 (best genes from GenEx) were validated in each single sample and then used for normalization of qRT-PCR data. Relative quantification (RQ) values were calculated using the equation RQ = 2-ΔΔCt.
Peripheral blood monocytic cells: cDNA synthesis and qRT-PCR
One microgram of total RNA was used to perform first-strand cDNA synthesis with mirCURY LNATM Universal cDNA synthesis kit (Exiqon) according to the manufacturer's protocol. The quantitative RT-PCR was performed using 80 × diluted cDNA in order to quantify the expression of miR-181a, let-7b, miR-22, and miR-27b in SYBR Green technologies (Exiqon) according to the manufacturer's protocol. Data were normalized using miR-93 and miR-103, which showed remarkable stability in our samples.
Statistical analysis
Statistics were calculated using SPSS 16.0 (SPSS Inc., Chicago, IL, USA) and Prism 6.0 (Graphpad Inc., San Diego, CA, USA). Log10-transformed values were used for all computations to approximate a Gaussian distribution. After data transformation, the normality distribution was tested by the D’Agostino–Pearson omnibus normality test. Since Gaussian distribution was not confirmed for all the data, the non-parametric Mann–Whitney U test was used to test differences between the two groups (Supplementary Figure 1 to be found online at http://informahealthcare.com/doi/abs/10.3109/07853890.2015.1071871). The probability values were corrected for multiple comparisons by using the Benjamini–Hochberg approach, and the adjusted q values are reported. A q value < 0.05 was considered as statistically significant. Furthermore, a more restrictive Bonferroni approach was also applied. A logistic regression analysis, aimed at identifying the ability of deregulated miRNAs correctly to classify the study subjects, was performed as previously described (Citation20,Citation27). A ROC curve analysis was carried out in order to verify the goodness of model fitting on our study population. A P value < 0.05 was considered statistically significant.
Results
For the initial screening, a microarray covering over 170 miRNAs known to be highly enriched in plasma was performed on two pooled samples: one including RNAs extracted from plasma exosomes of migraine patients and the other from the balanced healthy control subjects. In spite of the limitation that the study of pooled samples could not provide definitive results in terms of statistical significance, this screening analysis led us to the identification of 22 potentially dysregulated circulating miRNAs in migraineurs as compared to controls (), which represented the basis for the subsequent analysis. Indeed, 10 of these microRNAs were selected according to their fold change between the two groups and their potential involvement in biological processes underlying migraine. These microRNAs (miR-22, miR-26a, miR-26b, miR-27b, miR-29b, miR-181a, miR-221, miR-30b, miR-30e, and let-7b) were therefore validated by qRT-PCR in each single sample from individual patients.
Figure 1. MiRNome analysis for circulating microRNAs in migraine. Schematic representation of circulating microRNAs as modulated by migraine. The x-axis indicates fold change in expression level on a log10 scale as compared to control subjects.
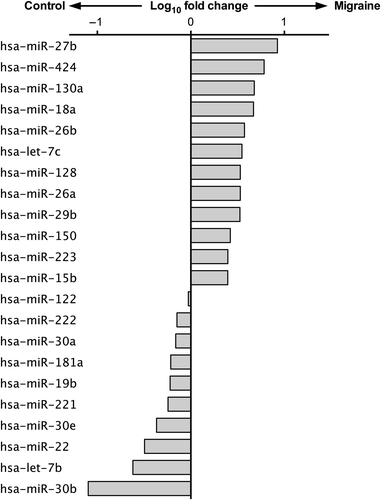
As depicted in , we found that miR-27b levels were significantly higher in migraineurs when compared with controls (crude P value < 0.001), whereas levels of miR-181a (crude P value < 0.001), let-7b (crude P value < 0.001), and miR-22 (crude P value = 0.004) were significantly lower. Interestingly, these differences between migraine patients and controls remained significant also after accounting for multiple comparisons according to the Benjamini–Hochberg false discovery rate (FDR) (q ≤ 0.01), as well as after the more conservative Bonferroni approaches (Bonferroni probability value < 0.005) (Supplementary Table II to be found online at http://informahealthcare.com/doi/abs/10.3109/07853890.2015.1071871). Despite a clear trend towards a differential expression, the differences found in the levels of miR-26a, miR-29b, miR-30b, miR-30e did not reach statistical significance.
Figure 2. Validation of circulating microRNAs expression on individual plasma samples by qRT-PCR. Data are shown as fold change from the control group. Significance levels were assessed by Mann–Whitney U test. * q < 0.05; ** q < 0.01; *** q < 0.001 (probability values corrected for multiple comparison by the Benjamini–Hochberg FDR method; n = 13 for control subjects, n = 15 for migraine patients).
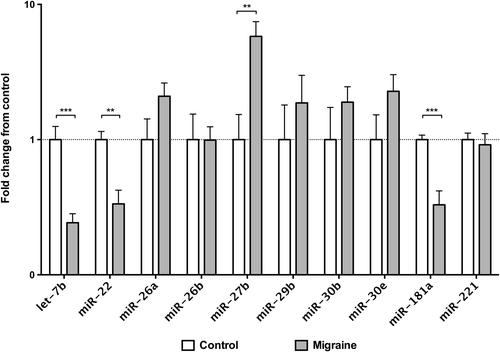
To confirm that the presence of this ‘microRNA signature’ was significantly associated with migraine in our study population, we entered the circulating level of miR-27b, miR-181a, let-7b, and miR-22 as independent variables in a binary logistic regression model. Interestingly, the model result was statistically significant (P < 0.001), and the derived receiver operating characteristic (ROC) curve demonstrated good accuracy of this model for statistically detecting migraineurs in our study population (AUC = 0.956; 0.887–1.000; P < 0.001) (). Thus, the concomitant evaluation of the circulating levels of these microRNAs might have the same accuracy for diagnosis of MO of the currently available clinical diagnostic criteria in our population (Citation23).
Figure 3. Receiver-operating characteristic (ROC) curve derived from the logistic regression model developed with circulating let-7b, miR-22, miR-27b, and miR-181a as predictors. AUC = area under curve.
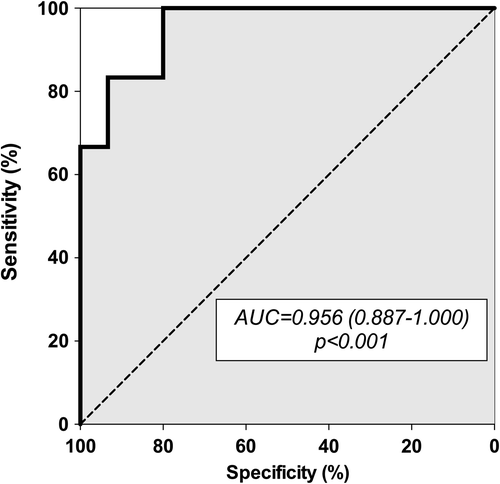
Finally, because complex mechanisms regulate microRNAs packaging and release, and the circulating microRNAs might not reflect their intracellular levels (Citation11,Citation28), we analysed the expression of let-7b, miR-22, miR-27b, and miR-181a in PBMCs from the blood of all the subjects included in our study by qRT-PCR. Notably, our results showed that intracellular levels of let-7b (P = 0.035) and miR-22 (P = 0.004) were lower in patients with migraine in accordance with exosome circulating levels (). Conversely, despite a clear trend of association between lower intracellular miR-181a levels and migraine, this difference did not reach statistical significance. Finally, in contrast with exosome levels, the intracellular levels of miR- 27b levels were apparently not modulated by migraine in our population ().
Figure 4. Expression of microRNAs in peripheral blood mononuclear cells (PBMCs) assessed by qRT-PCR. Data are shown as fold change from the control group. Significance levels were assessed by Mann–Whitney U test. *P < 0.05; ** P < 0.01; *** P < 0.001 (n = 13 for control subjects, n = 15 for migraine patients).
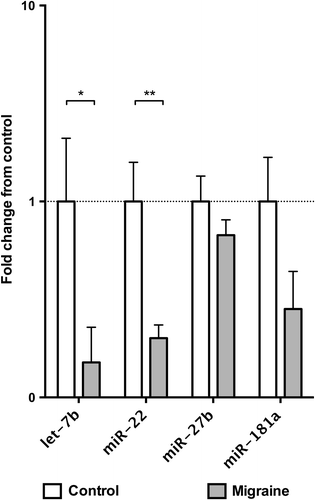
Discussion
To the best of our knowledge, in this study we provide the first evidence that female patients affected exclusively with migraine without aura exhibit a unique circulating exosome miRNA signature. Specifically, we found that the expression of four miRNAs was significantly different in MO patients versus controls: miR-27b was up-regulated while miR-181a, let-7b, and miR-22 were down-regulated. It is worth noting that in our population sensitivity and specificity of the microRNA signature were highly comparable to the ‘gold-standard’ of clinical criteria, suggesting that the concomitant evaluation of these four miRNAs might represent a new powerful tool for identifying MO status, although future prospective studies on larger and independent study populations will be needed for confirmation of the current data (Citation23).
Some other findings furthermore arise from our study, which concern the relationship between migraine and CV risk, a topic that has been debated for years. Although this relationship is complex and likely to involve multiple factors, one possible pathophysiological connection put forward in previous studies lies in the endothelial dysfunction (ED) advocated in migraine (Citation29,Citation30). ED is a clinical syndrome that is described by impaired reactivity of the vasculature: the association of migraine attacks with changes in endothelial biomarkers suggests that migraine attacks may be the cause of the ED. Recent research has, in particular, focused on the possible link between migraine without aura and vascular events by evaluating endothelial function by chemical and functional markers of endothelium in MO as compared with the normal population. The main finding of this study was a defective flow-mediated dilatation (FMD) and a decreased nitrite concentration in MO versus controls, showing a clear endothelial dysfunction in this form of migraine, and suggesting that MO could also be a risk for cardiovascular disease (Citation31).
Dysfunction of the endothelium is also the first step in the development of atherosclerotic lesions, and miRNAs are involved in every stage of the biological process of atherosclerosis, from endothelium dysfunction to cellular adhesion, plaque development, and plaque rupture (Citation32). A recent study by Bao et al. (Citation33) specifically showed protective effects of let-7a and let-7b on oxidized low-density lipoprotein-induced endothelial cell injuries, providing new insights into the protective properties of let-7a and let-7b in preventing the endothelial dysfunction associated with cardiovascular disease, such as atherosclerosis. Interestingly, our study on MO patients has shown a down-regulation of let-7b, which may represent a specific index of increased risk of endothelial dysfunction common to CV and migraine pathophysiology.
Also the other miRNAs identified as being altered in MO in our study have indeed been previously associated with vascular (dys)function and CV events, although not specifically with ED. MiR-27b has been found to be over-expressed in patients with peripheral artery disease, and its levels correlate with the severity of the disease (Citation34). Another member of the miR-27 family (miR-27a) has recently been found to be increased in plasma of patients with spontaneous intracerebral haemorrhage (Citation35). On the other hand, miR-181a and also let-7b have been found to be modulated in plasma of young patients with ischemic stroke, thus supporting their involvement in cerebrovascular diseases (Citation21). Furthermore, a reduction of miR-181a has been independently associated with cardiovascular diseases in patients with metabolic syndrome, and among its putative targets are several key genes involved in vascular biology, inflammatory response, and atherosclerosis, including NO-synthase and toll-like receptor (TLR)-4 (Citation36). Finally, miR-22 might represent an additional intriguing link between migraine and cardiovascular diseases, at least in the female sex. In fact, it has been demonstrated that miR-22 influences the estrogen pathway by directly targeting the estrogen receptor (ER)-α (Citation37). The observation that ER-α mutations are associated with both migraine and cardiovascular diseases suggests a functional link between miR-22, migraine, and cardiovascular events (Citation38,Citation39). In this context, the observation that circulating miR-22 truly reflects its intracellular levels in inflammatory cells (such as monocytes evaluated in our study population) would further support the role of this miRNA in migraine.
Interestingly, previously published reports have found miRNAs to be modulated in several other conditions that represent well-established cardiovascular risk factors. Indeed, conditions such as type-2 diabetes (Citation11,Citation27,Citation40), hypertension (Citation22,Citation41,Citation42), hypercholesterolemia (Citation43,Citation44), as well as the presence of atherosclerotic plaque vulnerability (Citation10,Citation20), have been associated with specific changes in microRNA expression. In our microarray analysis (), however, most of these microRNAs were not modulated in our study population, thus potentially suggesting a specific modulation process in patients with MO. The nature of our research being preliminary, further studies on larger independent and prospective populations will be needed to investigate further the complex links among the different forms of migraine, microRNA, and cardiovascular risk. Furthermore, despite about 2,000 microRNAs being annotated so far, our screening procedure included only the 175 of them (Supplementary Table I to be found online at http://informahealthcare.com/doi/abs/10.3109/07853890.2015.1071871) most commonly detected in plasma, thus potentially not highlighting the possible modulation of other microRNAs not frequently investigated in plasma samples. In spite of the limitations of the present set of experiments, however, we believe that the identification of a specific miRNA profile in female migraineurs without aura might represent a step towards a better characterization of the disease pathophysiology and open new avenues for the identification of molecular targets for future pharmacological strategies in the treatment of this highly common form of migraine.
Supplementary material available online
Supplementary Table I & II and Figure 1 to be found online at http://informahealthcare.com/doi/abs/10.3109/07853890.2015.1071871
iann_a_1071871_sm8577.pdf
Download PDF (584.6 KB)Acknowledgements
We are deeply indebted to Domenico De Cesare for his invaluable technical assistance, to Christian Ries for his kind suggestions on the revision of the final manuscript, and to Lesley Skeens for revising the English language.
E.T., D.S., and V.d.N. share first authorship; M.A.G. and F.C. share senior authorship.
Funding: This study was supported by a grant from the Italian Ministry of University and Scientific Research (COFIN MIUR 2009 protocol 2009L4X28T_002).
Declaration of interest: The authors report no conflicts of interest.
References
- Murray CJ, Lopez AD. Measuring the global burden of disease. N Engl J Med. 2013;369:448–57.
- Headache Classification Committee of the International Headache Society. The international classification of headache disorders, 3rd edition (beta version). Cephalalgia. 2013;33:629–808.
- Giamberardino MA, Martelletti P. Emerging drugs for migraine treatment. Expert Opin Emerg Drugs. 2015;20:137–47.
- Tana C, Santilli F, Martelletti P, di Vincenzo A, Cipollone F, Davi G, et al. Correlation between migraine severity and cholesterol levels. Pain Pract. 2014 Jul 10. [Epub ahead of print].
- Kurth T, Diener HC. Migraine and stroke: perspectives for stroke physicians. Stroke. 2012;43:3421–6.
- Tana C, Tafuri E, Tana M, Martelletti P, Negro A, Affaitati G, et al. New insights into the cardiovascular risk of migraine and the role of white matter hyperintensities: is gold all that glitters? J Headache Pain. 2013;14:9.
- Kurth T, Gaziano JM, Cook NR, Bubes V, Logroscino G, Diener HC, et al. Migraine and risk of cardiovascular disease in men. Arch Intern Med. 2007;167:795–801.
- Santovito D, Mezzetti A, Cipollone F. MicroRNAs and atherosclerosis: new actors for an old movie. Nutr Metab Cardiovasc Dis. 2012;22:937–43.
- Santulli G, Wronska A, Uryu K, Diacovo TG, Gao M, Marx SO, et al. A selective microRNA-based strategy inhibits restenosis while preserving endothelial function. J Clin Invest. 2014;124:4102–14.
- Wronska A, Kurkowska-Jastrzebska I, Santulli G. Application of microRNAs in diagnosis and treatment of cardiovascular disease. Acta Physiol (Oxf). 2015;213:60–83.
- Mastropasqua R, Toto L, Cipollone F, Santovito D, Carpineto P, Mastropasqua L. Role of microRNAs in the modulation of diabetic retinopathy. Prog Retin Eye Res. 2014;43:92–107.
- Charan Reddy K.Regulatory noncoding RNAs in cardiovascular disease: shedding light on ‘Dark Matter.’ J. Cardiovasc. Dis. 2015;3:301–7.
- Creemers EE, Tijsen AJ, Pinto YM. Circulating microRNAs: novel biomarkers and extracellular communicators in cardiovascular disease? Circ Res. 2012;110:483–95.
- Kress M, Huttenhofer A, Landry M, Kuner R, Favereaux A, Greenberg D, et al. microRNAs in nociceptive circuits as predictors of future clinical applications. Front Mol Neurosci. 2013;6:33.
- Parkitny L, McAuley JH, Di Pietro F, Stanton TR, O’Connell NE, Marinus J, et al. Inflammation in complex regional pain syndrome: a systematic review and meta-analysis. Neurology. 2013;80:106–17.
- Orlova IA, Alexander GM, Qureshi RA, Sacan A, Graziano A, Barrett JE, et al. MicroRNA modulation in complex regional pain syndrome. J Transl Med. 2011;9:195.
- Andersen HH, Duroux M, Gazerani P. MicroRNAs as modulators and biomarkers of inflammatory and neuropathic pain conditions. Neurobiol Dis. 2014;71:159–68.
- Bjersing JL, Bokarewa MI, Mannerkorpi K. Profile of circulating microRNAs in fibromyalgia and their relation to symptom severity: an exploratory study. Rheumatol Int. 2015;35:635–42.
- Li X, Gibson G, Kim JS, Kroin J, Xu S, van Wijnen AJ, et al. MicroRNA-146a is linked to pain-related pathophysiology of osteoarthritis. Gene. 2011;480:34–41.
- Cipollone F, Felicioni L, Sarzani R, Ucchino S, Spigonardo F, Mandolini C, et al. A unique microRNA signature associated with plaque instability in humans. Stroke. 2011;42:2556–63.
- Tan KS, Armugam A, Sepramaniam S, Lim KY, Setyowati KD, Wang CW, et al. Expression profile of microRNAs in young stroke patients. PLoS One. 2009;4:e7689.
- Santovito D, Mandolini C, Marcantonio P, De Nardis V, Bucci M, Paganelli C, et al. Overexpression of microRNA-145 in atherosclerotic plaques from hypertensive patients. Expert Opin Ther Targets. 2013;17:217–23.
- Headache Classification Subcommittee of the International Headache Society. The international classification of headache disorders: 2nd edition. Cephalalgia. 2004;24(Suppl 1):9–160.
- Fillingim RB, King CD, Ribeiro-Dasilva MC, Rahim-Williams B, Riley JL 3rd. Sex, gender, and pain: a review of recent clinical and experimental findings. J Pain. 2009;10:447–85.
- Arroyo JD, Chevillet JR, Kroh EM, Ruf IK, Pritchard CC, Gibson DF, et al. Argonaute2 complexes carry a population of circulating microRNAs independent of vesicles in human plasma. Proc Natl Acad Sci U S A. 2011;108:5003–8.
- Valadi H, Ekstrom K, Bossios A, Sjostrand M, Lee JJ, Lotvall JO. Exosome-mediated transfer of mRNAs and microRNAs is a novel mechanism of genetic exchange between cells. Nat Cell Biol. 2007;9:654–9.
- Santovito D, De Nardis V, Marcantonio P, Mandolini C, Paganelli C, Vitale E, et al. Plasma exosome microRNA profiling unravels a new potential modulator of adiponectin pathway in diabetes: effect of glycemic control. J Clin Endocrinol Metab. 2014;99:E1681–5.
- Kosaka N, Iguchi H, Yoshioka Y, Takeshita F, Matsuki Y, Ochiya T. Secretory mechanisms and intercellular transfer of microRNAs in living cells. J Biol Chem. 2010;285:17442–52.
- Lee ST, Chu K, Jung KH, Kim DH, Kim EH, Choe VN, et al. Decreased number and function of endothelial progenitor cells in patients with migraine. Neurology. 2008;70:1510–17.
- Mikirova NA, Jackson JA, Hunninghake R, Kenyon J, Chan KW, Swindlehurst CA, et al. Circulating endothelial progenitor cells: a new approach to anti-aging medicine? J Transl Med. 2009;7:106.
- Heshmat-Ghahdarijani K, Javanmard SH, Sonbolestan SA, Saadatnia M, Sonbolestan SA. Endothelial function in patients with migraine without aura during the interictal period. Int J Prev Med. 2015;6:2
- Nishiguchi T, Imanishi T, Akasaka T. MicroRNAs and cardiovascular diseases. Biomed Res Int. 2015;2015:682857.
- Bao MH, Zhang YW, Lou XY, Cheng Y, Zhou HH. Protective effects of let-7a and let-7b on oxidized low-density lipoprotein induced endothelial cell injuries. PLoS One. 2014;23;9:e106540.
- Li T, Cao H, Zhuang J, Wan J, Guan M, Yu B, et al. Identification of miR-130a, miR-27b and miR-210 as serum biomarkers for atherosclerosis obliterans. Clin Chim Acta. 2011;412:66–70.
- Guo D, Liu J, Wang W, Hao F, Sun X, Wu X, et al. Alteration in abundance and compartmentalization of inflammation-related miRNAs in plasma after intracerebral hemorrhage. Stroke. 2013;44:1739–42.
- Hulsmans M, Sinnaeve P, Van der Schueren B, Mathieu C, Janssens S, Holvoet P. Decreased miR-181a expression in monocytes of obese patients is associated with the occurrence of metabolic syndrome and coronary artery disease. J Clin Endocrinol Metab. 2012;97: E1213–18.
- Pandey DP, Picard D. miR-22 inhibits estrogen signaling by directly targeting the estrogen receptor alpha mRNA. Mol Cell Biol. 2009;29:3783–90.
- Shearman AM, Cooper JA, Kotwinski PJ, Humphries SE, Mendelsohn ME, Housman DE, et al. Estrogen receptor alpha gene variation and the risk of stroke. Stroke. 2005;36:2281–2.
- Shearman AM, Cooper JA, Kotwinski PJ, Miller GJ, Humphries SE, Ardlie KG, et al. Estrogen receptor alpha gene variation is associated with risk of myocardial infarction in more than seven thousand men from five cohorts. Circ Res. 2006;98:590–2.
- Zampetaki A, Kiechl S, Drozdov I, Willeit P, Mayr U, Prokopi M, et al. Plasma microRNA profiling reveals loss of endothelial miR-126 and other microRNAs in type 2 diabetes. Circ Res. 2010;107:810–17.
- Li S, Zhu J, Zhang W, Chen Y, Zhang K, Popescu LM, et al. Signature microRNA expression profile of essential hypertension and its novel link to human cytomegalovirus infection. Circulation. 2011;124:175–84.
- Marques FZ, Booth SA, Charchar FJ. The emerging role of non-coding RNA in essential hypertension and blood pressure regulation. J Hum Hypertens. 2015;29:459–67.
- Mandolini C, Santovito D, Marcantonio P, Buttitta F, Bucci M, Ucchino S, et al. Identification of microRNAs 758 and 33b as potential modulators of ABCA1 expression in human atherosclerotic plaques. Nutr Metab Cardiovasc Dis. 2015;25:202–9.
- Karolina DS, Tavintharan S, Armugam A, Sepramaniam S, Pek SL, Wong MT, et al. Circulating miRNA profiles in patients with metabolic syndrome. J Clin Endocrinol Metab. 2012;97:E2271–6.