Abstract
Objective. Although sleep is a biomarker for general health and pathological conditions, its changes across age and gender are poorly understood.
Methods. Subjective evaluation of sleep was assessed by questionnaires in 5,064 subjects, and 2,966 were considered without sleep disorders. Objective evaluation was performed by polysomnography in 2,160 subjects, and 1,147 were considered without sleep disorders. Only subjects without sleep disorders were included (aged 40–80 years).
Results. Aging was strongly associated with morning preference. Older subjects, especially women, complained less about sleepiness, and pathological sleepiness was significantly lower than in younger subjects. Self-reported sleep quality and daytime functioning improved with aging. Sleep latency increased with age in women, while sleep efficiency decreased with age in both genders. Deep slow-wave sleep decreased with age, but men were more affected. Spectral power densities within slow waves (< 5 Hz) and fast spindles (14–14.75 Hz) decreased, while theta-alpha (5-1 Hz) and beta (16.75–25 Hz) power in non-rapid eye movement sleep increased with aging. In REM sleep, aging was associated with a progressive decrease in delta (1.25–4.5 Hz) and increase in higher frequencies.
Conclusions. Our findings indicate that sleep complaints should not be viewed as part of normal aging but should prompt the identification of underlying causes.
Key words: :
Older subjects complain less about sleepiness, and pathological sleepiness is significantly lower than younger subjects; self-reported sleep quality and daytime functioning also improve with aging.
Sleep latency increases with age in women only, while sleep efficiency decreases with age in both genders.
Sleep complaints in older subjects are not normal and should prompt the identification of underlying causes.
Introduction
Sleep is an essential biological function, and its structure, duration, and quality are altered in many conditions, especially with aging. The exact contribution of aging per se or of the interaction between age and multiple factors (e.g. biological, socio-economical, psychological) to sleep changes is difficult to disentangle. Sleep structure, duration, and quality have different dynamics during aging and associated disorders, but common patterns can be identified both in healthy aging and in subjects with intrinsic sleep disorders. These include increased sleep onset latency and wake after sleep onset (WASO), increased non-rapid eye movement (NREM) sleep stage 1 (shallow sleep), and reduced amount of slow-wave sleep (SWS or deep sleep) (Citation1–3). Sleep duration, objectively measured using polysomnography, was found to be shorter in the elderly compared to young and middle-aged adults (Citation4), but when comparing subjects aged 60 with subjects over 70 years this trend was not observed, suggesting that the decline is not linear (Citation5).
Beyond sleep architecture, specific electroencephalographic (EEG) features can be altered. For example, aging was associated with diminished power density within the slow frequency range (0.5–4.5 Hz) during NREM sleep, the so-called delta power, a highly reliable index of sleep intensity (Citation6,Citation7). The beta power density, that measures high-frequency EEG activity, was shown to increase with aging, along with decreases in sigma, delta, and theta band powers in almost all sleep stages, suggesting a general alteration in the sleep EEG (Citation4).
Gender is an important factor affecting the modulation of sleep. Previous studies reported poorer subjective sleep quality in elderly women (Citation8), but polysomnographic data showed a better sleep structure (Citation9) in terms of higher SWS and decreased WASO (Citation10) compared to elderly men. It was proposed that different psychosocial factors may contribute to gender dissimilarities in the perception and evaluation of symptoms (Citation10) which may contribute to the differences in self-rating sleep quality. Women usually complain about difficulties in falling asleep (Citation11), while men have more problems in sleep maintenance, lighter sleep, and more frequent sleep-related breathing disorders (Citation12). More recent studies also revealed gender differences in the sleep EEG. Differences between men and women were suggested in terms of brain maturation, perceived sleep, NREM–REM distribution, or EEG features (Citation13–20).
A major source of sleep alteration in aging populations is the increasing occurrence of sleep disorders. Insomnia is one of the most common sleep disorders with an increase in prevalence and severity with aging, especially in women (Citation21). The prevalence of obstructive sleep apnea syndrome also increases with aging (Citation22). Periodic limb movements in sleep (often associated with restless legs syndrome) are highly prevalent in the general population (Citation23–25) and increase in both elderly men and women (Citation26). Given the high prevalence of sleep disorders in older subjects with their well-documented impact on sleep structure, careful exclusion of those subjects is necessary to evaluate the history of sleep changes across age and gender.
Thus, the aim of this study was to perform a detailed subjective and objective characterization of normal sleep and the sleep EEG in relation with age and gender in one of the largest European sleep cohorts: the HypnoLaus study. Moreover, the impact of changes in normal sleep on daytime sleepiness, sleep quality, and daytime functioning were also analyzed.
Materials and methods
Study population
HypnoLaus Cohort Study participants were recruited from the original CoLaus population during the first follow-up. The CoLaus study was designed to assess the prevalence of cardiovascular and metabolic disorders, their risk factors, and to identify their genetic determinants (Citation27). All subjects (6,733 participants, aged 35–75 years), randomly selected between 2003 and 2006 from the adult general population of Lausanne (Switzerland), underwent a comprehensive somatic and genetic investigation. HypnoLaus participants completed a series of self-rating sleep pattern and disorder questionnaires. Altogether 5,064 participants, who agreed to participate in the follow-up, correctly filled in the sleep questionnaires (46.5% women). There were no demographic differences between original CoLaus and HypnoLaus. Out of these, 3,051 consecutive subjects were invited for an ambulatory full-night polysomnography, without any prior selection based on the questionnaires; 2,166 subjects (71.0% of contacted) agreed to participate. Those who declined were 8.2 years older, mildly sleepier (Epworth + 0.4 points), and with a slightly higher Pittsburgh Sleep Quality Index (PSQI) score (+ 0.3 points). Technical issues were encountered in recordings of 60 subjects, but 54 agreed to repeat the study, resulting in a final sample of 2,160 subjects (51.2% women).
Sleep questionnaires
All participants were asked to fill in questionnaires to assess chronotype, sleepiness, and sleep quality. Chronotype was assessed by the Horne and Östberg Morningness–Eveningness Questionnaire (MEQ), which is based on 19 questions. The chronotype classification of subjects was performed according to Taillard et al. (Citation28). Sleepiness was assessed by the Epworth Sleepiness Scale (ESS), which is widely used to asses sleepiness in the general population and in different sleep disorders, and rates the chance of falling asleep in eight different situations (Citation29). Scores higher than 10 (range 0–24) are associated with excessive daytime sleepiness. Sleep quality was assessed by the Pittsburgh Sleep Quality Index (PSQI), which is an effective instrument for subjective evaluation of sleep quality over seven domains (Citation30): subjective sleep quality, sleep latency, sleep duration, sleep efficiency, sleep disturbances, use of sleep medication, and daytime dysfunctions over the last month. For each domain, the score ranges between 0 and 3, where 3 is the negative extreme. A total score higher than 5 (out of 21) is associated with poor sleep quality (Citation30). There was a 6–18 month delay between the questionnaire administration and the polysomnographic evaluation. Therefore, in the morning following the polysomnographic recording participants completed another questionnaire providing information about the quality of their sleep the previous night, the amount of alcohol consumed 4 hours before going to bed, and their current medication.
Polysomnography
Full ambulatory polysomnography was performed using a digital portable sleep/wake recording system (EMBLA Titanium®, Embla systems, Inc., Broomfield, CO, USA). Sensors were placed by a trained technician at CIRS (Center for Investigation and Research in Sleep, Lausanne University Hospital, Lausanne, Switzerland). The following measurements were performed: electroencephalogram (EEG) from frontal, central, and occipital areas (F3-M2, F4-M1, C3-M2, C4-M1, O1-M2, O2-M1) according to the international 10/20 electrodes configuration system, right and left electro-oculogram (EOG), submental electromyogram (EMG), right and left leg EMG, thoracic and abdominal breathing movements (inductance plethysmography), electrocardiography, pulse oximetry, respiratory airflow via nasal cannula connected to a pressure transducer, and body position. Subjects were instructed to follow their habitual sleep habits.
Sleep stages, leg movements, and arousals were scored according to the 2007 American Academy of Sleep Medicine (AASM) criteria (Citation31). Apneas/hypopneas were scored according to the AASM 2012 rules (Citation32). The average number of apneas/hypopneas per hour of sleep (apnea/hypopnea index (AHI)) was calculated. Analyzed polysomnographic (PSG) variables were: sleep duration or total sleep time (TST) (total minutes of any stage of sleep from sleep onset to morning awakening); wake after sleep onset (in minutes); percentage of stage 1, stage 2, SWS, and REM sleep (percentage of TST spent in each sleep stage); sleep efficiency (ratio between the total sleep time and time spent in bed); arousal index (AI), periodic leg movements index during sleep (PLMSI); 4% oxygen desaturation index (ODI) (number of events (AI, PLMSI, and ODI, respectively) divided by hours of TST).
Spectral analysis
Signals were filtered by a high-pass filter (EEG and EOG: − 3 dB at 0.5 Hz; EMG: 10 Hz), a low-pass filter (EEG: − 3 dB at 35 Hz; EMG: 70 Hz), and a notch filter at 50 Hz. Data were sampled at 256 Hz. C3-M2 EEG derivation was subjected to spectral analysis. Sleep stages and the events were visually scored by two registered PSG technologists on a 30-s epoch basis (Somnologica® Software, Medcare Embla® Flaga, Reykjavik, Iceland) according to AASM criteria (Citation31). The EEG power spectra of consecutive 30-s epochs (average of 4-s epoch interval with 50% overlap, fast Fourier transform routine, Hamming window, frequency resolution 0.25 Hz) for NREM sleep (stage 2 and stage 3) and REM sleep were calculated using (PRANA®, PhiTools, Strasbourg, France) software (Citation33). Movement, arousals, and respiratory events were considered artifacts and removed from the analysis. An automatic artifact detection and removal was performed using PRANA® software (Citation33), but each recording was also visually inspected for the correction of the artifact selection, and additional artifacts were manually marked. All epochs containing artifact were removed from spectral analysis. NREM–REM cycles were defined according to Feinberg and Floyd (Citation34). Sleep-onset REM period was defined as at least one epoch of REM sleep occurring within the first 15 min of sleep. NREM and REM sleep spectral analysis was separately calculated for the first four sleep cycles. Besides EEG spectral composition, delta (0.75–4.5 Hz), theta (4.75–8 Hz), alpha (8.25–11 Hz), slow spindles (11.25–13 Hz), fast spindles (13.25–15 Hz), and beta (15–25 Hz) bands were calculated. To account for interindividual differences, EEG power spectra were normalized by dividing the power in each 0.25-Hz bin by the total power for NREM and REM sleep over a 0.75–30 Hz frequency range. A total of 114 recordings were not used in the analysis due to: lack of signal on C3-M2 EEG channel, mirror of ECG in EEG channels, and increased amount of artifacts which increased the probability of the remaining amount being non-representative for the whole-night EEG. There were no age or gender differences between excluded subjects and remaining ones.
Selection of subjects without sleep complaints
To assess the effects of age and gender on subjective measures a ‘non-complaining’ population was selected based on information obtained from PSQI. Subjects who declared sleep complaints (mainly environmental such as noise including the partner's snoring, personal or job-related stressors, n = 418), shift workers (n = 30), those taking any sleep medication (n = 985), and those reporting the presence of diagnosed sleep disorders (n = 933) were excluded from the analysis ().
Figure 1. Selection of the population with normal sleep. *Sleep complaints: mainly environmental disturbances. **More than twice per week. ***Documented sleep disorder (insomnia, sleep-related breathing disorders or frequent awakenings due to other problems). 4% ODI = 4% oxygen desaturation index; PLMI = periodic leg movement index. All percentages are relative to the total population subjectively (n = 5,064) or objectively (n = 2,160) investigated.
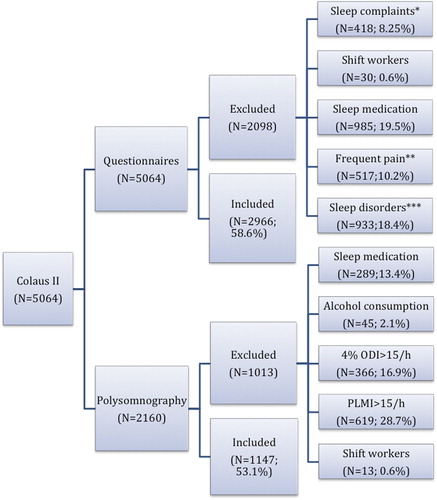
From the polysomnographic data were excluded subjects taking medication which interferes with sleep structure and/or duration (n = 289), with alcohol consumption (2 glasses of wine or equivalent) consumed 4 hours prior to sleep recording (n = 45), with 4% oxygen desaturation index and AHI > 15/h (n = 366) indicating the presence of moderate to severe sleep apnea, or with periodic limb movements index higher than 15/hour (n = 619). Note that for both subjective and objective measures an excluded subject could fulfill one or several exclusion criteria ().
Statistical analysis
Subjective parameters analyzed were: MEQ scores and chronotype, Epworth Sleepiness Scale (ESS) score, PSQI total score, and, separately, each domain and sleep estimates (bedtime, sleep onset latency, wake-up time, total sleep duration). Subjective habitual sleep duration was used either as a continuous variable or as a categorical (less than 6 h, between 6 and 7.5 h, and more than 7.5 h of sleep). The following polysomnographic data were used: total sleep time, sleep onset latency, wake after sleep onset, sleep efficiency, arousal index and sleep stages distribution, periodic leg movement, and oxygen desaturation events during sleep. Since some recordings were performed on a Friday night, polysomnographic data were adjusted also for the day of the recording (Friday or not). A total of 12% of our population were recorded on Friday, and their mean sleep duration was 24 minutes (± 6.1 min SEM) longer. No other sleep variables differed between week-days and week-ends.
To analyze the effects of age, four 10-year age groups were created (40–49 y; 50–59 y; 60–69 y; 70–80 y), and one-way ANOVA/Kruskal–Wallis test or chi-square were used to test the effect of age (as categorical variable). T tests, chi-square, or Fisher's exact tests were performed to test the effect of gender. To assess the interaction between age and gender for questionnaires and polysomnographic and spectral analysis data, a two-way ANOVA (followed by Holm–Sidak post-hoc multiple comparisons tests) or multivariate logistic regression was performed. To derive the mid-sleep point, bedtime, sleep onset, wake-up time sleep estimates from PSQI were used. Multinomial logistic regression was used to evaluate the chronotype in relationship with age (unadjusted model) or in relationship with daytime functioning (adjusted for age and gender). Partial correlation analysis was used for testing the association between demographic, subjective, and objective data. Pearson correlation analysis was used to evaluate the association between polysomnographic parameters.
All statistical tests were computed using PASW 18.0 Statistics (IBM SPSS Statistics, Armonk, NY, USA), and statistical significance for all tests was assumed when P < 0.05.
Results
Subjective sleep evaluation
After removing subjects with declared disturbed sleep, 2,966 participants (50.8% women; mean age 56.8 ± 10.4 years) were considered ‘non-complaining’ and included in the analysis. Population characteristics and self-rated sleep pattern results are summarized in .
Table I. Effects of age and gender on sleep habits.
Chronotype
Aging was associated with a gradual shift towards morningness (). The likelihood of being a morning type was 5.9 times higher in older subjects compared with younger ones (). The diurnal preference measured by the MEQ could also be approximated by mid-sleep point, time to go to sleep, time to fall asleep, and time to wake up from the PSQI (age and gender-adjusted partial correlations: r = –0.473, r = –0.464, r = –0.471, and r = –0.334, P < 0.001 respectively). Older subjects went to bed and fell asleep earlier than younger ones, and their subjective sleep duration was longer (). Gender effect was stronger for younger subjects, with women going to bed and falling asleep earlier, and sleeping longer (). Reported sleep latency increased in women with aging (70–80 y.o. versus 40–49 y.o. group, and 70–80 y.o. versus 50–59 y.o. group, P < 0.001). Sleep efficiency decreased both in men and women with aging, with 40–49 y.o. and 50–59 y.o. groups having higher sleep efficiency than the other two groups. The presence of sleep disturbances was highest in 50–59 y.o. women and lowest in the oldest women (P < 0.05). The gender effect was stronger in the youngest population, with women going earlier to sleep compared with men, but these differences dissipate with age. Overall 40–49 y.o. subjects went to bed later than the oldest (40–49 y.o. versus 70–80 y.o., and 50–59 versus 70–80 y.o., P < 0.01). The youngest participants woke up earlier compared with the older ones (40–49 y.o. versus 60–69 y.o. and versus 70–80 y.o., P < 0.001; 50–59 y.o. versus 60–69 y.o. and versus 70–80 y.o., P < 0.001).
Figure 2. (A) The prevalence of morning (black line) and evening type (red line) according to age (n = 2,966). Morning types were all ‘definite’ or ‘moderate’ morning type participants, and all participants included in ‘definite’ and ‘moderate’ evening type were considered as evening type. Black asterisks mark significant differences between age groups (multinomial logistic regression, P < 0.001). (B) Declared sleep duration (minutes): black bars represent men and red bars women; black lines connect significantly different age groups, and asterisks mark gender differences (two-way analysis of variance with factors ‘age group’, P < 0.05, ‘gender’, P < 0.05, and ‘age group × gender’, P = n.s.). (C) Daytime functioning (red line) and sleep quality (black line) improves with aging (asterisks mark significant differences between age groups, chi-square test, P < 0.001; each point represents the percentage of the participants rating their daytime functioning and sleep quality as very good). (D) The prevalence of excessive daytime sleepiness decreases with aging: black bars represent men and red bars women; asterisks mark gender differences (chi-square test, P < 0.001). Excessive daytime sleepiness was considered based on the ESS scores, using a cut-off of 10.
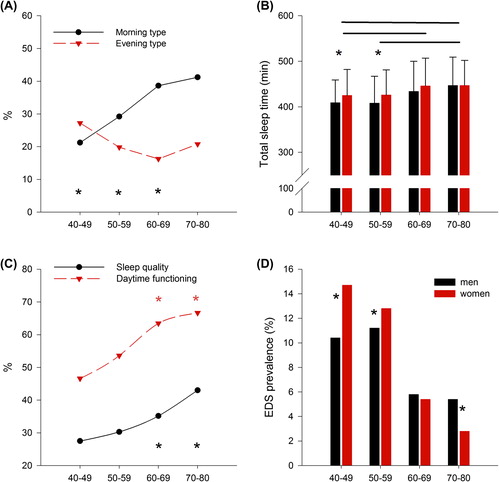
Table II. Effects of age on chronotype.
Daytime sleepiness and sleep quality
The ESS score decreased with aging, and the reduction was larger in women (two-way ANOVA for ‘age’, ‘gender’, ‘age × gender interaction’, P <0.001). Also, the prevalence of excessive daytime sleepiness (EDS), evaluated by an ESS higher than 10, decreased with aging from 11.7% among participants aged 40–49 years to 6.4% in men and from 14.1% to 2.3% in women aged over 70 (, ). The only self-assessed sleep variable correlated with increased daytime sleepiness was short sleep duration. Subjects who slept less than 6 h/night had the highest ESS scores compared with normal/long sleepers (two-way ANOVA, P < 0.001 for ‘age’ and ‘total sleep time category’, P = 0.08 for the interaction). After controlling for sleep duration, the association between age and excessive daytime sleepiness remained highly significant. Before adjusting for sleep duration, the likelihood of reporting excessive daytime sleepiness was 3.11 times higher in the 40–49 y.o. group compared with the 70–80 y.o. group (95% CI 1.7–5.7), and 2.98 times higher in the 50–59 y.o. group compared with the 70–80 y.o. group (95% CI 1.6–5.4, P < 0.001). After adjusting for sleep duration, the likelihood of reporting excessive daytime sleepiness was 2.9 times higher (1.6–5.4) in the 40–49 y.o. group versus the 70–80 y.o. group, and 2.8 times higher (1.5–5.1) in the 50–59 y.o. group versus the 70–80 y.o. group (P < 0.001). Self-reported sleep quality and daytime functioning measured by PSQI improved with aging (, ).
Polysomnographic data
A total of 1,147 of the 2,160 subjects who underwent polysomnographic (PSG) recording were considered free of significant sleep disorders. Women slept on average 26 min longer than men, and overall sleep duration diminished by only 28 minutes across age groups (). Sleep onset latency was not significantly affected by age or gender. Sleep efficiency decreased with age both in men and women (two-way ANOVA for age P < 0.0001, gender P = 0.002, interaction P = 0.2). SWS amount decreased with age, with men being more affected (two-way ANOVA for age P < 0.0001, gender P < 0.001, interaction P = 0.009). REM sleep duration was inversely correlated with REM sleep latency (r = –0.319, P < 0.0001), and the same negative correlation was found between SWS latency and SWS amount (r = –0.288, P < 0.0001). As expected, a higher proportion of stage 1 sleep was associated with decreased sleep efficiency (r = –0.312, P < 0.0001) and increased sleep fragmentation (r = 0.407, P < 0.0001). Men spent more time in stage 1, independent of age, and had less SWS and REM sleep ().
Table III. Effects of age and gender on variables assessed by polysomnography.
Subjective versus objective sleep evaluation
Subjects older than 70 years estimated their sleep duration better than younger subjects (Spearman correlation between declared sleep in PSQI and PSG recorded sleep: r = 0.10, P = 0.06 for the subjects aged 40–49 years and r = 0.302, P = 0.007 for those aged 70–80 years, slopes significantly different, P < 0.05). SWS and REM sleep amounts were not associated with any of the sleepiness or sleep quality measures. Sleep quality estimated in the morning following the polysomnography was strongly correlated with PSQI sleep quality (Spearman correlation r = 0.64, P < 0.001).
Spectral analysis
Out of 1,147 PSG recordings of normal sleepers, 776 which contained at least 4 sleep cycles and sleep efficiency > 80% were subjected to spectral analysis
NREM sleep
presents the average relative NREM EEG spectra for each age group. A significant decrease with increasing age was observed in low frequencies (1–3 Hz; 4.25–5.00 Hz) (all age groups significantly different) and high spindles (14.00–14.75 Hz, with no differences between 40–49 y.o. and 50–59 y.o. groups; 60–69 y.o. and 70–80 y.o. groups, but significant differences between 40–49 y.o. versus 60–69 y.o. and 70–80 y.o. groups, and 50–59 y.o. versus 60–69 y.o. and 70–80 y.o. groups) and an increase in theta-alpha (5.00–11.00 Hz; with the 70–80 y.o. group significantly different from all other age groups, and 60–69 y.o. group significantly different from youngest group) and beta frequencies (16.75–25 Hz; all age groups significantly different; two-way ANOVA, ‘age group’, ‘frequency bin’, and ‘age group × frequency bin’, P < 0.001). When analyzing the association of different sleep stages with spectral results, men and women were differently affected. For example, increased amount of stage 1 sleep in older women was negatively associated with spindle frequency power (r = –0.393, P = 0.02), while in men, no association was found. This finding suggests gender differences in spectral EEG composition and its relationship with sleep architecture. To test overall age and gender interaction for spectral data, separately for NREM and REM sleep, three-way analysis of variance (‘age group’, ‘gender’, and ‘frequency bin’) was performed. Interaction between age and gender was significant both for NREM (F = 6.085, P < 0.001) and REM sleep (F = 3.555, P < 001). We therefore tested first the effect of gender overall, and then assessed the effect of age separately for women and men. When considering the absolute powers of spectral analysis, women had higher power in a large frequency range (0.75–1.25 Hz; 4.75–13 Hz, P < 0.001) compared with men, but after normalizing for total power, women had lower power for a small frequency range in the delta (2–2.75 Hz) and beta band (16.25–25 Hz) and higher power in theta-alpha band (5.75–10.25 Hz) (P < 0.001, t test) (). With aging, women showed an increasing trend in power density in high frequencies (), except fast spindles. In men () the largest age-related increase was found in the beta band.
Figure 3. Effect of age (A) and gender (B) on NREM-relative EEG spectral power (n = 776, logarithmic scale). Down-oriented triangles connect frequencies for which power densities were significantly decreased with age, while up-oriented triangles connect frequencies for which power densities were significantly increased with age. Effect of age (C) and gender (D) on REM sleep-relative EEG spectral power. Down-oriented triangles connect frequencies for which power densities were significantly decreased with age, while up-oriented triangles connect frequencies for which power densities were significantly increased with age (P < 0.05). EEG power spectra were normalized by dividing the power in each 0.25 Hz bin by the total power for NREM and REM sleep, respectively, over the 0.75–30 Hz frequency range. (Two-way ANOVA for factors ‘age group’, P < 0.05, ‘bin’, P < 0.0001, and ‘age group × bin’, P < 0.05, and for factors ‘gender’, ‘bin’, and their interaction, P < 0.05). Overall, 60–69 y.o. and 70–80 y.o. groups differed significantly from 40–49 y.o. and 50–59 y.o.
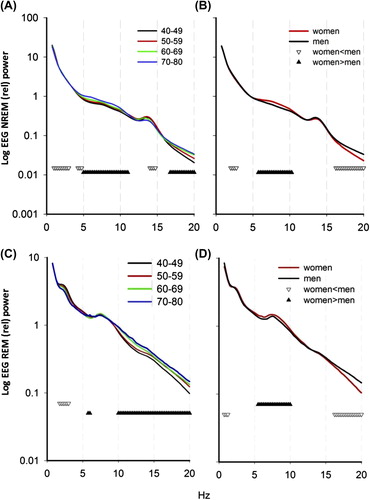
REM sleep
Spectral composition of REM sleep was not affected by sleep structure, duration, or efficiency. Nevertheless, higher index of spontaneous arousal was associated with higher alpha power (r = 0.112, P = 0.001), and total arousal index was correlated with power in slow sigma frequency band (r = 0.136, P = 0.0003, partial correlation adjusted for age and gender).
Except very low frequencies (0.75–1.25 Hz), which were not altered, aging was associated with a progressive decrease in delta power and an increase in power in high frequencies (). The interaction between age and gender was also evident in REM sleep. Women had higher theta and lower beta frequency powers, while men had higher power in the low-frequency range (). To understand how age affects each gender specifically, age groups were compared separately in men and women. For women the only decrease was observed in delta frequency range, while alpha and sigma power increased. Importantly, very high frequencies (high beta) were unchanged in women with aging. In contrast, the most important increase in men was found in the beta frequency range (), followed by increase in sigma band. Delta frequency in REM sleep was not affected in men with aging, yet theta power decreased.
Discussion
The present work aimed to reassess sleep, with special focus on its changes across age and gender in a large representative sample of normal sleepers from the general population. To that end, subjective and objective measurements of sleep and sleep habits were analyzed.
Chronotype
Our results confirm that old age is associated with a higher prevalence of morning types, when using MEQ (Citation35). Both circadian clock and environmental factors regulate the chronotype. The difference between morning and evening types in terms of daytime functioning and total PSQI score was also described and explained previously by ‘social jetlag’ experienced on a daily basis by evening-type subjects (Citation36). Dissipation of gender differences due to aging is also supported by previous work (Citation37). Shifting to morningness explains the earlier time in bed, while later wake-up time and longer sleep duration with aging might contradict the circadian phase advance hypothesis in older subjects. Recent data suggested that sleep duration seems independent of chronotype (Citation38–41). One important confounding factor might be retirement, which would attenuate the difference between work and free days (social jetlag). The exact time of retirement was not available in our population. The impact of chronic sleep deprivation due to societal and occupational obligations may contribute to the differences in TST and wake-up time; also, component 7 (daytime dysfunction) could have been altered due to this factor.
Daytime sleepiness
Surprisingly, elderly people declare themselves less sleepy. Young age was previously associated with increased sleepiness (Citation42,Citation43), but it was shown that, at very old age, people experience increased sleepiness again (Citation43), which is not supported by our results. Also, in our study, the lowest ESS scores were obtained in subjects over 70 years of age. Overall, the prevalence of sleepiness in our study is lower than previously reported (Citation44–47). The fact that the lowest ESS scores were reported by the oldest participants, as well as a decreased prevalence of EDS in our sample, is supported by previous data suggesting that EDS in the elderly is not a normal phenomenon, but rather a sign of an underlying disturbance affecting daytime alertness (Citation44–47).
Subjective sleep quality
Reported sleep quality improved with age, as well as the daytime functioning. Similar results were found in other cohorts (Citation48). One possible explanation for better rating of daytime and sleep quality is an adaptation of expectations about sleep in older populations (Citation49) or an acclimatization to sleep changes over time (Citation50). Recent data (Citation51) suggest an association between self-rated sleep quality and duration in aging populations with markers of cellular aging, such as telomere length, which reinforces the importance of promoting and maintaining healthy sleep in aging.
Effects of age and gender on sleep structure
Sleep structure is strongly influenced by age and gender. These findings are supported by previous studies, especially the fact that men have more shallow sleep than women (Citation2,Citation10,Citation52). Alterations in sleep structure with aging, especially the decrease in SWS amount, could be attributed to hormonal influences, such as a decrease in growth hormone pulse observed both in men and women (Citation53,Citation54). Also recently, a reduced number of galanin-immunoreactive neurons in the intermediate nucleus was associated with sleep fragmentation in elderly subjects both with and without Alzheimer's disease (Citation55). Age-related differences in sleep stage amounts are more pronounced after 60 years of age, and this may be one of the reasons why other studies, which analyzed sleep in young and middle-aged adults (Citation56), did not find differences in REM sleep amount. The decrease in SWS starts earlier, in middle-aged men and women, and was well-documented previously (Citation3,Citation20,Citation57,Citation58). Interestingly, despite the fact that SWS is an index of sleep quality and intensity, aged subjects are less sleepy than younger ones, even if they lose most of their SWS (Citation59). Experimental disruption of SWS in the elderly resulted in increased sleepiness (Citation59), but the daytime functioning was not impaired (Citation60), suggesting that elderly subjects might manage their sleepiness better.
Effects of age and gender on EEG spectral composition
Age-related changes in the sleep EEG, such as the progressive power density decrease in low frequencies accompanying the reduction in SWS amount, are concordant with previous results (Citation56,Citation61). Another major component of aging, accentuated by gender, was the spindle frequency spectrum. Our findings showing an increase in slow spindle frequency and attenuation in fast spindle frequency bands confirm the hypothesis of two distinct components (Citation56), differently affected by the aging process. EEG and brain imaging studies demonstrated different origins (Citation62) and distinct temporal dynamics (Citation63) of spindles, which might be differentially affected by aging.
In spite of a better preservation of sleep architecture with aging in women, they had lower delta and beta power and higher alpha and sigma power densities than men. Previous studies which used the same principle for normalizing the spectral data (Citation64) reported also that, in spite of a higher absolute delta power, women had lower delta power contribution to the total power, while the beta band was attenuated compared with men. The functional significance of these changes are unknown but might be of major importance in terms of gender differences in sleep need and associated daytime alertness and cognitive processes.
Fast EEG frequencies (> 15 Hz) were previously associated with insomnia (Citation65), a sleep disorder with increased prevalence in women with aging (Citation66), but it was also suggested that in middle-aged subjects it is difficult to distinguish between age-related changes and insomnia (Citation67). In our study beta frequency was higher in men than in women, both in NREM and REM sleep. Contrary to previous findings, which suggested a better preservation of delta power in NREM sleep in men and in REM sleep in women (Citation64), in our sample women had lower relative delta power also in REM sleep, and the age effect on this frequency band was more pronounced in women compared with men. Methodological issues (e.g. normalization of data, larger sample size) may explain some of the differences between previous findings and ours.
Limitations
We report in this study how age and gender contribute to the changes in sleep quality, structure, and EEG spectral composition in one of the largest population-based cohorts. Since the presence of sleep disorders was carefully excluded, one may consider our population as ‘supernormal’. Nevertheless, we did not exclude subjects with an unexplained subjective sleepiness (ESS > 10) or those with moderate alterations such as ODI or PLMSI < 15/hour. Direct comparison between subjective and objective measures might be compromised in our study by a time gap between the administration of the questionnaires and polysomnography. However, it was shown that PSQI measures remain stable over a year (Citation68), and sleep quality estimated in the morning following the polysomnography in our study was strongly correlated with PSQI, with better sleep quality in those with lower PSQI scores. Finally, lack of information on menopausal status, retirement, and napping during the day before polysomnography could impact our findings,
In conclusion, we found that age-related changes in sleep do not affect subjective sleepiness or daytime functioning. Presence of sleep complaints should not be viewed as part of normal aging, but should prompt the identification of underlying co-morbidities. Normative data for sleep should be adapted for aging populations to reduce overmedication in the elderly. Our findings on the history of sleep micro- and macro-structure can be used as standards to evaluate impaired sleep structure in populations with specific pathologies. Moreover, the prospective clinical follow-up of this cohort (in progress) will determine the clinical significance of changes in sleep states and associated EEG features in terms of incident neurologic and psychiatric diseases.
Acknowledgements
R.H. and M.T. are co-senior authors and contributed equally to this paper.
Funding: This work was supported by grants from Leenaards Foundation, Swiss National Science Foundation (122661, 139468, and 148491), Marie Curie Actions (Neuroendocrine Immune Networks in Ageing Project, NINA Project 238665), GlaxoSmithKline, Lausanne University, and University Hospital of Lausanne (CHUV). G.L. was supported by Marie Curie Actions.
Declaration of interest: The authors report no conflicts of interest.
References
- Dijk DJ. Sleep of aging women and men: back to basics. Sleep. 2006; 29:12–13.
- Redline S, Kirchner H, Quan SF, Gottlieb DJ, Kapur V, Newman A. The effects of age, sex, ethnicity, and sleep-disordered breathing on sleep architecture. Arch Intern Med. 2004;164:406–18.
- Vitiello MV. Sleep in normal aging. Sleep Medicine Clinics. 2012;7: 539–44.
- Carrier J, Land S, Buysse DJ, Kupfer DJ, Monk TH. The effects of age and gender on sleep EEG power spectral density in the middle years of life (ages 20–60 years old). Psychophysiology. 2001;38:232–42.
- Ohayon MM, Carskadon MA, Guilleminault C, Vitiello MV. Meta-analysis of quantitative sleep parameters from childhood to old age in healthy individuals: developing normative sleep values across the human lifespan. Sleep. 2004;27:1255–73.
- Dijk DJ, Brunner DP, Beersma DG, Borbely AA. Electroencephalogram power density and slow wave sleep as a function of prior waking and circadian phase. Sleep. 1990;13:430–40.
- Borbély AA, Baumann F, Brandeis D, Strauch I, Lehmann D. Sleep deprivation: effect on sleep stages and EEG power density in man. Electroencephalogr Clin Neurophysiol. 1981;51:483–93.
- Li RH, Wing YK, Ho SC, Fong SY. Gender differences in insomnia— a study in the Hong Kong Chinese population. J Psychosom Res. 2002;53:601–9.
- Fukuda N, Honma H, Kohsaka M, Kobayashi R, Sakakibara S, Kohsaka S, et al. Gender difference of slow wave sleep in middle aged and elderly subjects. Psychiatry Clin Neurosci. 1999;53:151–3.
- Rediehs MH, Reis JS, Creason NS. Sleep in old age: focus on gender differences. Sleep. 1990;13:410–24.
- Rodin J, McAvay G, Timko C. A longitudinal study of depressed mood and sleep disturbances in elderly adults. J Gerontol. 1988;43:P45–53.
- Wilhoit SC, Suratt PM. Obstructive sleep apnea in premenopausal women. A comparison with men and with postmenopausal women. Chest. 1987;91:654–8.
- Dijk DJ, Beersma DG, Bloem GM. Sex differences in the sleep EEG of young adults: visual scoring and spectral analysis. Sleep. 1989;12: 500–7.
- Armitage R. The distribution of EEG frequencies in REM and NREM sleep stages in healthy young adults. Sleep. 1995;18:334–41.
- Baker FC, Turlington SR, Colrain I. Developmental changes in the sleep electroencephalogram of adolescent boys and girls. J Sleep Res. 2012; 21:59–67.
- Campbell IG, Grimm KJ, de Bie E, Feinberg I. Sex, puberty, and the timing of sleep EEG measured adolescent brain maturation. Proc Natl Acad Sci U S A. 2012;109:5740–3.
- Carrier J, Viens I, Poirier G, Robillard R, Lafortune M, Vandewalle G, et al. Sleep slow wave changes during the middle years of life. Eur J Neurosci. 2011;33:758–66.
- Dijk DJ, James LM, Peters S, Walsh JK, Deacon S. Sex differences and the effect of gaboxadol and zolpidem on EEG power spectra in NREM and REM sleep. J Psychopharmacol. 2010;24:1613–18.
- Feinberg I, Campbell IG. Sleep EEG changes during adolescence: an index of a fundamental brain reorganization. Brain Cogn. 2010;72: 56–65.
- Mourtazaev MS, Kemp B, Zwinderman AH, Kamphuisen HA. Age and gender affect different characteristics of slow waves in the sleep EEG. Sleep. 1995;18:557–64.
- Leger D, Guilleminault C, Dreyfus JP, Delahaye C, Paillard M. Prevalence of insomnia in a survey of 12 778 adults in France. J Sleep Res. 2000;9:35–42.
- Tufik S, Santos-Silva R, Taddei JA, Bittencourt LR. Obstructive sleep apnea syndrome in the Sao Paulo Epidemiologic Sleep Study. Sleep Med. 2010;11:441–6.
- Allen RP, Walters AS, Montplaisir J, Hening W, Myers A, Bell TJ, et al. Restless legs syndrome prevalence and impact: REST general population study. Arch Intern Med. 2005;165:1286–92.
- Innes KE, Selfe TK, Agarwal P. Prevalence of restless legs syndrome in North American and Western European populations: a systematic review. Sleep Med. 2011;12:623–34.
- Hogl B, Kiechl S, Willeit J, Saletu M, Frauscher B, Seppi K, et al. Restless legs syndrome: a community-based study of prevalence, severity, and risk factors. Neurology. 2005;64:1920–4.
- Moraes W, Piovezan R, Poyares D, Bittencourt LR, Santos-Silva R, Tufik S. Effects of aging on sleep structure throughout adulthood: a population-based study. Sleep Med. 2014;15:401–9.
- Firmann M, Mayor V, Vidal P, Bochud M, Pecoud A, Hayoz D, et al. The CoLaus study: a population-based study to investigate the epidemiology and genetic determinants of cardiovascular risk factors and metabolic syndrome. BMC Cardiovasc Disord. 2008;8:6.
- Taillard J, Philip P, Chastang J-F, Bioulac B. Validation of Horne and Ostberg morningness-eveningness questionnaire in a middle-aged population of French workers. J Biol Rhythms. 2004;19:76–86.
- Johns MW. A new method for measuring daytime sleepiness: the Epworth sleepiness scale. Sleep. 1991;14:540–5.
- Buysse DJ, Reynolds CF 3rd, Monk TH, Berman SR, Kupfer DJ. The Pittsburgh Sleep Quality Index: a new instrument for psychiatric practice and research. Psychiatry Res. 1989;28:193–213.
- Iber C, Ancoli-Israel S, Chesson AL, Quan SF. The AASM manual for the scoring of sleep and associated events: rules, terminology, and technical specifications. 1st ed. Westchester, IL: American Academy of Sleep Medicine, 2007.
- Berry RB, Budhiraja R, Gottlieb DJ, Gozal D, Iber C, Kapur VK, et al. Rules for scoring respiratory events in sleep: update of the 2007 AASM Manual for the Scoring of Sleep and Associated Events. Deliberations of the Sleep Apnea Definitions Task Force of the American Academy of Sleep Medicine. J Clin Sleep Med. 2012;8:597–619.
- Zoubek L, Chapotot F. Automatic classification of sleep/wake stages using two-step system. In: Snasel V, Platos J, El-Qawasmeh E, editors. Digital information processing and communications. Communications in computer and information science. Berlin: Springer; 2011. p. 106–17.
- Feinberg I, Floyd TC. Systematic trends across the night in human sleep cycles. Psychophysiology. 1979;16:283–91.
- Tankova I, Adan A, Buela-Casal G. Circadian typology and individual differences. A review. Personality and Individual Differences. 1994;16: 671–84.
- Wittmann M, Dinich J, Merrow M, Roenneberg T. Social jetlag: misalignment of biological and social time. Chronobiol Int. 2006;23: 497–509.
- Roenneberg T, Kuehnle T, Juda M, Kantermann T, Allebrandt K, Gordijn M, et al. Epidemiology of the human circadian clock. Sleep Med Rev. 2007;11:429–38.
- Randler C, Ebenhöh N, Fischer A, Höchel S, Schroff C, Stoll JC, et al. Chronotype but not sleep length is related to salivary testosterone in young adult men. Psychoneuroendocrinology. 2012;37:1740–4.
- Adan A, Archer SN, Hidalgo MP, Di Milia L, Natale V, Randler C. Circadian typology: a comprehensive review. Chronobiol Int. 2012;29: 1153–75.
- Roenneberg T, Kuehnle T, Pramstaller PP, Ricken J, Havel M, Guth A, et al. A marker for the end of adolescence. Curr Biol. 2004;14:R1038–9.
- Viola AU, Archer SN, James LM, Groeger JA, Lo JCY, Skene DJ, et al. PER3 polymorphism predicts sleep structure and waking performance. Curr Biol. 2007;17:613–18.
- Pallesen S, Nordhus IH, Omvik S, Sivertsen B, Tell GS, Bjorvatn B. Prevalence and risk factors of subjective sleepiness in the general adult population. Sleep. 2007;30:619–24.
- Bixler EO, Vgontzas AN, Lin H-M, Calhoun SL, Vela-Bueno A, Kales A. Excessive daytime sleepiness in a general population sample: the role of sleep apnea, age, obesity, diabetes, and depression. J Clin Endocrinol Metab. 2005;90:4510–15.
- Vashum KP, McEvoy MA, Hancock SJ, Islam MR, Peel R, Attia JR, et al. Prevalence of and associations with excessive daytime sleepiness in an Australian older population. Asia Pac J Public Health. 2015;27: NP2275–84.
- Wu S, Wang R, Ma X, Zhao Y, Yan X, He J. Excessive daytime sleepiness assessed by the Epworth Sleepiness Scale and its association with health related quality of life: a population-based study in China. BMC Public Health. 2012;12:849.
- Souza JC, Magna LA, Reimao R. Excessive daytime sleepiness in Campo Grande general population, Brazil. Arq Neuropsiquiatr. 2002;60: 558–62.
- Baldwin CM, Kapur VK, Holberg CJ, Rosen C, Nieto FJ. Associations between gender and measures of daytime somnolence in the Sleep Heart Health Study. Sleep. 2004;27:305–11.
- Doi Y, Minowa M, Uchiyama M, Okawa M. Subjective sleep quality and sleep problems in the general Japanese adult population. Psychiatry Clin Neurosci. 2001;55:213–15.
- Vitiello MV, Larsen LH, Moe KE. Age-related sleep change: gender and estrogen effects on the subjective-objective sleep quality relationships of healthy, noncomplaining older men and women. J Psychosom Res. 2004;56:503–10.
- Unruh ML, Redline S, An MW, Buysse DJ, Nieto FJ, Yeh JL, et al. Subjective and objective sleep quality and aging in the sleep heart health study. J Am Geriatr Soc. 2008;56:1218–27.
- Cribbet MR, Carlisle M, Cawthon RM, Uchino BN, Williams PG, Smith TW, et al. Cellular aging and restorative processes: subjective sleep quality and duration moderate the association between age and telomere length in a sample of middle-aged and older adults. Sleep. 2014;37:65–70.
- Hume K, Van F, Watson A. A field study of age and gender differences in habitual adult sleep. J Sleep Res. 1998;7:85–94.
- Van Cauter E, Plat L, Copinschi G. Interrelations between sleep and the somatotropic axis. Sleep. 1998;21:553–66.
- van Coevorden A, Mockel J, Laurent E, Kerkhofs M, L’Hermite-Baleriaux M, Decoster C, et al. Neuroendocrine rhythms and sleep in aging men. Am J Physiol. 1991;260:E651–61.
- Lim AS, Ellison BA, Wang JL, Yu L, Schneider JA, Buchman AS, et al. Sleep is related to neuron numbers in the ventrolateral preoptic/ intermediate nucleus in older adults with and without Alzheimer's disease. Brain. 2014;137:2847–61.
- Landolt HP, Dijk DJ, Achermann P, Borbely AA. Effect of age on the sleep EEG: slow-wave activity and spindle frequency activity in young and middle-aged men. Brain Res. 1996;738:205–12.
- Bliwise DL. Sleep in normal aging and dementia. Sleep. 1993;16: 40–81.
- Ehlers CL, Kupfer DJ. Slow-wave sleep: do young adult men and women age differently? J Sleep Res. 1997;6:211–15.
- Dijk DJ, Groeger JA, Stanley N, Deacon S. Age-related reduction in daytime sleep propensity and nocturnal slow wave sleep. Sleep. 2010;33:211–23.
- Groeger JA, Stanley N, Deacon S, Dijk DJ. Dissociating effects of global SWS disruption and healthy aging on waking performance and daytime sleepiness. Sleep. 2014;37:1127–42.
- Landolt HP, Borbely AA. Age-dependent changes in sleep EEG topography. Clin Neurophysiol. 2001;112:369–77.
- Ayoub A, Aumann D, Horschelmann A, Kouchekmanesch A, Paul P, Born J, et al. Differential effects on fast and slow spindle activity, and the sleep slow oscillation in humans with carbamazepine and flunarizine to antagonize voltage-dependent Na+ and Ca2 + channel activity. Sleep. 2013;36:905–11.
- Tagaya H, Trachsel L, Murck H, Antonijevic I, Steiger A, Holsboer F, et al. Temporal EEG dynamics of non-REM sleep episodes in humans. Brain Res. 2000;861:233–40.
- Latta F, Leproult R, Tasali E, Hofmann E, Van Cauter E. Sex differences in delta and alpha EEG activities in healthy older adults. Sleep. 2005;28:1525–34.
- Krystal AD, Edinger JD, Wohlgemuth WK, Marsh GR. NREM sleep EEG frequency spectral correlates of sleep complaints in primary insomnia subtypes. Sleep. 2002;25:630–40.
- Calem M, Bisla J, Begum A, Dewey M, Bebbington PE, Brugha T, et al. Increased prevalence of insomnia and changes in hypnotics use in England over 15 years: analysis of the 1993, 2000, and 2007 National Psychiatric Morbidity Surveys. Sleep. 2012;35:377–84.
- Bastien CH, LeBlanc M, Carrier J, Morin CM. Sleep EEG power spectra, insomnia, and chronic use of benzodiazepines. Sleep. 2003;26: 313–17.
- Knutson KL, Rathouz PJ, Yan LL, Liu K, Lauderdale DS. Stability of the Pittsburgh Sleep Quality Index and the Epworth Sleepiness Questionnaires over 1 year in early middle-aged adults: the CARDIA study. Sleep. 2006;29:1503–6.