Abstract
Introduction. Scanning laser Doppler flowmetry (SLDF) plays an important role in the study of arterial hypertension, diabetes and stroke. The technology enables non-invasive measurement of the retinal capillary perfusion (RCF), retinal haemodynamics and arteriolar morphology in human. The values can be measured in mydriasis or in non-mydriatic eyes. It is not clear whether the using of vasoactive mydriatica for pupil dilation affects the measured parameters in retina. Acetylcholine, a vasoactive neurotransmitter in human retina, affects the contractility of pericytes using muscarinic receptors and stimulates endothelial synthesis of nitric oxide (NO). We examined whether blockade of the retinal cholinergic receptors by tropicamide affects the RCF. Methods. We measured RCF in both eyes of 13 healthy subjects before and 30 min after the local application of one drop of 0.5% tropicamide to the right eye. The mean age of the group was 44 ± 14 years. The left eye was used as control. RCF was measured by Heidelberg retina flowmetry. Results. Thirty minutes after local application of one drop of 0.5% tropicamide to the right eye RCF decreased significantly (p = 0.001) by 31.9 ± 13% but did not change in the control eye. The maximal decrease was observed 20 min after application of the tropicamide. Conclusion. Locally administered tropicamide profoundly affects the RCF. Thus pupil dilatation impairs any assessment of retinal microcirculation.
Introduction
Scientific interest in SLDF measurements in the study of arterial hypertension, stroke and diabetes has recently risen substantially. The SLDF unique technology uses eye transparency and enables the non-invasive measurement of the retinal capillary perfusion (RCF), retinal haemodynamics and arteriolar morphology in human. The RCF measurement, its vasodilatory capacity and retinal arteriolar morphology is of significant importance for the study of early vascular damages in microvascular eye disease as well as in atherosclerotic processes, but the elementary problem on the measurement in mydriasis or in non-mydriatic eyes has not yet been clarified.
Retinal vessels do not have autonomic innervation. In the retina, the blood flow (RCF) is controlled by local and myogenic autoregulation, and the local control of capillary perfusion is performed by endothelial cells and pericytes (Citation1–3). The retinal vasculature has a higher density of these cells than any other vascular tissue (Citation2,Citation3), and the functional interaction of pericytes with endothelial cells is determined by the location of pericytes on the microvessel. Pericytes contain microfilament bundles with a contractile capability. The bundles of microfilaments are usually concentrated in the cytoplasm of pericytes in advance to the endothelial cells. One function of retinal pericytes is to mediate signals from retinal neurones to the retinal vessels, controlling retinal blood flow and adapting to the requirement of the retinal function (Citation4).
In the human retina, the known neurotransmitters between neurones and blood vessels are dopamine, gamma-aminobutyric acid and acetylcholine (Citation2,Citation5,Citation6). The potentially responsive cells for linking the metabolic demand of neuronal cells with capillary perfusion are starburst amacrine cells (Citation2,Citation6). In starburst amacrine cells, acetylcholine is located in synaptic connections (Citation6). It is possible that the acetylcholine reaches the pericytes and the endothelial cells of the retinal capillaries through diffusion or volume transfer as well (Citation2). In vitro the muscarinic acetylcholine receptors are present in the membrane of the retinal pericytes (Citation7). Retinal pericytes control the cytokine-induced expression of NO-synthase in microvascular endothelial cells (Citation8). It is well known that the vascular endothelium produces nitric oxide NO during exposure to acetylcholine and, conversely, the inhibitor of NO-synthesis NG-mono-methyl-l-arginin (l-NMMA) decreases retinal capillary blood flow (Citation2,Citation9–11). The exact mechanism of cholinergic modulation of NO-mediated vasodilatation has not been clarified up to now (Citation12–15). Thus it should be assumed that acetylcholine plays a vasoactive role in the retinal blood flow regulation by regulating the contractility of pericytes, NO production and, as a consequence, RCF (Citation2).
Tropicamide C17H20N2O2 – a benzeneacetamide parasympatholytic agent (N-ethyl-α-(hydroxymethyl)- N-(4-pyridylmethyl)-tropamide) – blocks the M4 muscarinic acetylcholine receptor (Citation16) and is commonly used to vasodilate the pupils prior to funduscopic examinations. Muscarinic receptors are involved controlling the pupil size and ciliary muscle tone. Tropicamide blocks the responses of the sphincter muscle of the iris and the ciliary muscle to cholinergic stimulation, thereby inducing a reversible dilatation of the pupil (mydriasis). Tropicamide acts within 15–30 min. The duration of activity is approximately 3–8 h. Complete recovery from mydriasis in some individuals may require 24 h. The effect of tropicamide on pupil diameter, intraocular pressure, visual acuity, refraction, iris thickness, iris–ciliary process distance, iris–zonule distance, anterior chamber angle, iris–sclera angle, sclera–ciliary process angle and relationship of pupil border to zonule-lens structures has been described (Citation17).
Impaired function of the endothelial vasoactivity is supposed to be a pathomechanism of some forms of retinal neuropathies and microvascular diseases. It plays a crucial role in the development of hypertensive and diabetic retinopathy (Citation10,Citation18–21) and can be investigated by SLDF. Moreover, RCF and retinal arteriolar morphology quantification methods are used in research of the vascular remodelling in artery hypertension as stroke risk factors (Citation10,Citation22–24). The retinal vessels form the blood retina barrier and are related to the brain vessels (Citation25), as retinal damage reflects hypertensive retinopathy (Citation26).
Objective
To date it has not been clearly specified whether the local application of tropicamide given to enlarge the pupil diameter is vasoactive on the retinal level and thereby falsifies any measurement of retinal circulation, arteriolar structure or function. Thus we tested the effect of local applicated tropicamide on the retinal capillary blood flow using SLDF technology.
Methods
The research followed the tenets of the Declaration of Helsinki for research involving human subjects and informed consent has been obtained from all subjects after explanation of the nature and possible consequences of the study.
Subjects
We examined 13 healthy persons (seven female, six male) aged 44 ± 14 years. All subjects were free of ophthalmologic diseases, cardiovascular or ophthalmovascular disorders; no persons were taking any medication known to affect the autonomic, cardiac or vascular system.
Study design
Mean, systolic and diastolic arterial blood pressure and heart beat frequency were measured with Accutor1 (Datascope Corp. Japan). The diameters of the pupil were examined by a pupil diameter measurement ruler (HumanOptics AG. Germany).
RCF was measured by scanning laser Doppler flowmetry (SLDF; Heidelberg Retina Flowmetry, Heidelberg Engineering GmbH Germany) (Citation27,Citation28), temporal to the optic nerve head. The laser beam wavelength was 670 μm. All images were analysed by Automatic Full Field Perfusion Image Analysis (AFFPIA; software SLDF version 3.6) (Citation29). The collected intensity data of each retinal measurement point are analysed by a discrete fast Fourier transformation. Thus the blood flow (ω) of each pixel can be computed by calculating the frequency shift for each point of measurement. Besides the Doppler effect, noise contributes to the measured intensity variations. Factor “NP” (noise power) is mainly based on photon statistics and calculated individually for each image. Finally, AFFPIA generates a two dimensional colour coded map of the retinal/optic nerve head perfusion from the calculated data. The brightness of each point is encoded by the extent of blood flow, i.e. to the Doppler frequency shift. The RCF (ω) was measured in arbitrary units [AU] and calculated by the equation:
To measure the influence of the pupil dilatation on the flow-images, the offset correction parameters (a, b) and averaged intensity of reflected light (DC, direct current) have been examined. The dependency of the noise power on the reflected light intensity (DC) was estimated by the equation (Citation28): NP = a√DC + b.
Each measurement was focused in dependency on the sharpness of the retina reflection image.
All parameters were measured before and 30 min after local application of one drop of 0.5% tropicamide (Mydriaticum Stulln® 0.5% Tropicamid Reg.Nr. M57 Pharma Stulln GmbH Germany) to the right eye. The left eye was used as control.
In a subgroup of six subjects (four female, two male) aged 33 ± 7 years the retinal capillary blood flow and the pupil diameter in both eyes were measured before, 1, 5, 10, 15, 20 and 30 min after local application of one drop of 0.5% tropicamide to the right eye. Again the left eye was left as control.
Statistical analysis
The means and the standard deviations of the RCF and pupil diameter measurements were calculated. To compare the mean values, the non-parametric Wilcoxon test for paired samples was used (software SPSS V.18.0, SPSS-Inc. USA).
Results
Mean RCF in the right eyes before tropicamide application was 320 ± 103 AU. Thirty minutes after application of tropicamide the mean RCF significantly (p = 0.001) decreased by 31.9 ± 13% to 217 ± 82 AU (, ).
Figure 1. Mean of retinal capillary blood flow before (OD-before; OS-before) and 30 min after tropicamide application to the right eye (OD-tropicamide; OS-control). OD, right eyes; OS, left eyes; significance **p < 0.01 (Wilcoxon test).
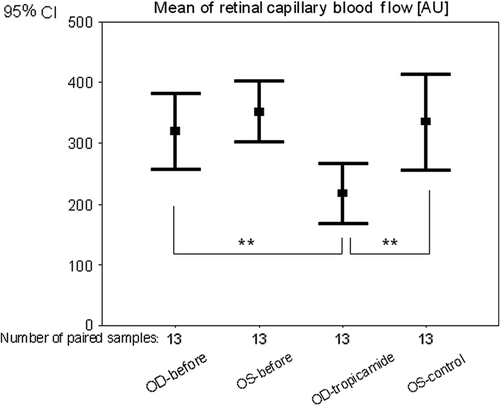
Table I. Mean ± standard deviation (SD) of retinal capillary blood flow (RCF) before and 30 min after tropicamide application to the right eye (tropicamide); left eye (control).
In the control eyes, the mean RCF changed non-significantly (p = 0.25) by − 6.8 ± 20% from 352 ± 84 AU to 335 ± 131 AU (, ).
Thirty minutes after application of tropicamide to the right eye, the RCF was significantly (p = 0.006) lower compared with the left eye ().
Figure 2. Mean differences in percent of baseline of retinal capillary blood flow 30 min after tropicamide application to the right eye and retinal capillary blood flow before application. OD, right eyes with local tropicamide application; OS, left eyes without tropicamide; significance **p < 0.01 (Wilcoxon test).
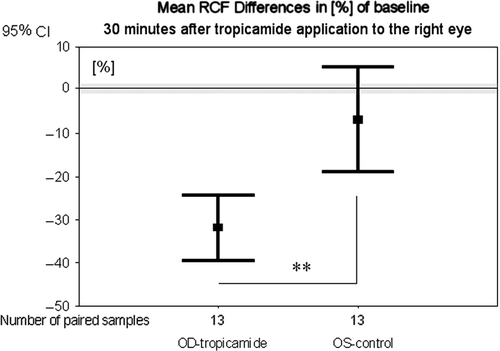
In contrast to the RCF values, the parameters of the offset correction (a, b), the reflected light intensity (DC) and the noise power (NP) showed no changes 30 min after application of 0.5% tropicamide between the test eye and the control eye.
Thirty minutes after tropicamide application, the pupil diameter increased from 3.6 ± 0.7 mm to 7.1 ± 1.3 mm significantly (p = 0.002). In the control eyes, the pupil diameter did not change.
Mean systolic and diastolic arterial blood pressure showed no significant changes before and 30 min after the local application of one drop of 0.5% tropicamide.
Time curve
In the subgroup of six subjects, after tropicamide application to the right eye, the retinal capillary blood flow showed no significant change after 1 and 5 min. After 10 min, the RCF decreased by 23 ± 20% (p = 0.046), after 20 min by 27 ± 15% (p = 0.028) and after 30 min by 12 ± 33% (, ). In the control eyes, at all times no significant changes of the retinal capillary blood flow were observed. The highest difference of RCF between treated right eyes and control left eyes was observed 10 min after the application of tropicamide. This difference reached 29.3 ± 23% (p = 0.028) (). In this group, the pupil diameter significantly increased from 3.8 ± 0.7 (before application) to 6.8 ± 1.0 mm (30 min after application) in the right eyes. In the collateral control eyes, the pupil diameter did not change. Mean, systolic and diastolic arterial blood pressure did not change significantly after local application of one drop of 0.5% tropicamide to the right eye.
Figure 3. Mean of retinal capillary blood flow (RCF) before, 1, 5, 10, 15, 20 and 30 min after tropicamide application to the right eye; N, number of paired samples; significance *0.05 ≥ p ≥ 0.01, comparison of RCF between the right eyes and the left eyes; #comparison between RCF in the time of the measurement after and before tropicamide application (Wilcoxon test).
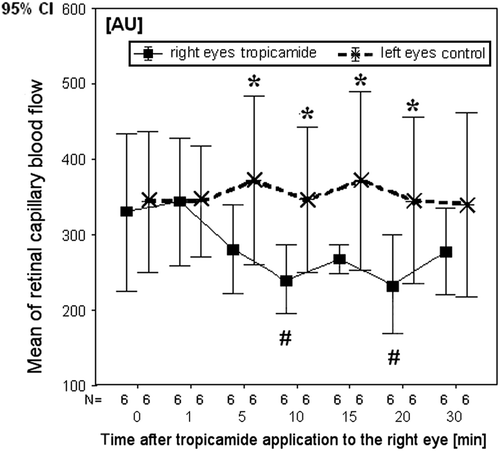
Table II. Mean ± standard deviation (SD) of retinal capillary blood flow (RCF) before, 1, 5, 10, 15, 20 and 30 min after tropicamide to the right eye; OD/OS difference – difference between RCF of the right eyes (OD) and control left eyes (OS).
Discussion
The RCF measurement by SLDF offers the opportunity to analyse non-invasively brain-related microcirculation and thereby is an important research tool of stroke and stroke risk factors study, as essential arterial hypertension, diabetes, metabolic syndrome or sleep apnoea (Citation22–24,Citation30). The method enables comparative study of the described parameters in patients with different types of hypertensive diseases, observation of the vascular changes in age, in duration of the disease, in the therapy control or in provocation tests.
The results of our study indicate that a local application of tropicamide is associated with a significant RCF reduction by approximately 30% in parapapillary retina. The maximal decrease of the RCF was observed 20 min after the application. No changes of RCF in the control eyes were observed and arterial blood pressure showed no significant change 30 min after local application of tropicamide. Furthermore, all technical parameters of the blood flow measurements by SLDF (offset correction, the intensity of the reflected light and the noise power) were nearly constant during mydriasis after local application of tropicamide. This clearly shows that the reduction of RCF is not related to the reflectivity and scatter characteristic of the retina by the dilatation of the pupil. It is also known that pharmacological mydriasis by tropicamide does not alter retinal nerve fibre layer thickness measured by scanning laser polarimetry (Citation31). Thus the changes found in Doppler shift are caused by a decreased retinal capillary blood flow.
Our observation confirms the results of Lanigan et al. (Citation32), who showed that 1% tropicamide increases retinal arteriolar and venular vasoconstriction, suggesting a vasoconstriction of the retinal vessels after the blockade of the muscarinic receptors with tropicamide. It is known that the vascular endothelium of larger vessels synthesizes the vasodilator NO during exposure to acetylcholine (Citation11,Citation12). On the other hand, Robinson et al. found in six healthy subjects that the velocity of leukocytes by blue field technique is not changed by topicamide in the macular capillaries (Citation33). The reason for the discrepancy might be the different area of the measurements (parapapillary vs macular capillary area) and/or the different setting of the investigations.
After local application of tropicamide, Baumann et al. observed a narrowing of the dye column in the corneal vessels in the vascularized cornea of the rabbit's eyes (Citation34). The observation supports our finding. This may be caused by decreasing RCF after application of tropicamide. The hypothesis that tropicamide is associated with a reduction in the RCF has the assumption that locally applied tropicamide diffuses to the retinal vessels of the posterior pole. At this time, no data are available to compare this association with tropicamide diffusion to the posterior pole after local application. Our data suggest such a pathway.
The results of the study establish the concept that local application of tropicamide is associated with a decreasing of the RCF. This might indicate that acetylcholine plays a regulatory role in the local control of perfusion in the human retina. In accordance, Wu et al. observed that the activation of muscarinic receptors induces reaction of pericytes, mainly at capillary bifurcation in isolated retinal microvessels (Citation2).
Perspectives
The clinical use of tropicamide in the ophthalmology, with exception of known risk factors, such as angle-closure glaucoma, is safe for inducing mydriasis. Our results indicate that RCF and morphology of retinal arterioles measurements must be performed in a setting without applying vasoactive agents to induce mydriasis. It simplifies the measurement and is convenient for the patients. On the other hand, the effects of RCF induced by tropicamide may be used as pharmacological provocation tool in retinal endothelial dysregulation studies.
In either case, the results demonstrate the necessity to carefully compare the results of RCF measurements performed in mydriasic vs in non-mydriatic eyes.
Acknowledgements
The authors thank Christiane Koehler for her excellent assistance. Supported by Deutsche Forschungsgemeinschaft Bonn SFB 539 BII.1 Germany. The sponsor organizations had no role in the design or conduct of this research.
Conflict of interest: The authors report no specific funding in relation to research and no conflicts of interest to disclose.
References
- Michels C. Endothelial cell function. J Cell Physiol. 2003;196:430–443.
- Wu DM, Kawamura H, Sakagami K, Kobayashi M, Puro DG. Cholinergic regulation of pericyte-containing retinal microvessels. Am J Physiol Heart Circ Physiol. 2003;284:H2083–H2090.
- Frank RN, Turczyn TJ, Das A. Pericyte coverage of retinal and cerebral capillaries. Invest Ophthalmol Vis Sci. 1990;31:999–1007.
- Alt G, Lawrenson JG. Pericytes: cell biology and pathology. Cell Tiss Org. 2001;169:1–11.
- Hutchins JB, Hollyfield JG. Acetylcholinesterase in the human retina. Brain Res. 1987;400:300–311.
- Yamada ES, Dmitrieva N, Keyeser KT, Lindstrom JM, Hersh LB, Marshak DW. Synaptic connections of starburst amacrine cells and localisation of acetylcholine receptors in primate retinas. J Comp Neurol. 2003;461:76–90.
- Ferrari-Dileo G, Davis EB, Anderson DR. Effects of cholinergic and adrenergic agonist on adenylate cyclase activity of retinal microvascular pericytes in culture. Invest Ophthalmol Vis Sci. 1992;33:42–47.
- Martin AR, Bailie JR, Robson T, McKeown SR., Al-Assar O, McFarland A, et al. Retinal pericytes control expression of nitric oxide synthase and endothelin1 in microvascular endothelial cells. Microvasc Res. 2000;59:131–139.
- Delaey C, Van de Voorde J. Regulatory mechanisms in the retinal and choroidal circulation. Ophth Res. 2000;32: 249–256.
- Delles C, Michelson G, Harazny J, Oehmer S, Hilgers KF, Schmieder RE. Impaired endothelial function of the retinal vasculature in hypertensive patients. Stroke 2004;35: 1289–1293.
- Palmer MJ, Ferrige AG, Moncada S. Nitric oxide release accounts for the biological activity of endothelium-derived relaxing factor. Nature. 1987;327:524–526.
- Loke KE, Sobey CG, Dusting GJ, Woodman OL. Cholinergic neurogenic vasodilatation is mediated by nitric oxide in the dog hindlimb. Cardiol Res. 1994;28:542–547.
- Liu J, Lee TJF. Mechanism of prejunctional muscarinic receptor-mediated inhibition of neurogenic vasodilatation in cerebral arteries. Am J Physiol Heart Circ Physiol. 1999;276: H194–H204.
- Yew DT, Chan WY, Luo CB, Zheng DR, Yu MC. Neurotransmitters and neuropeptides in the developing human central nervous system. Biol Sign Recept. 1999;8:149–159.
- Sakogami K, Kawamura H, Wu DM, Puro DC. Nitric oxide/cGMP-induced inhibition of calcium and chloride currents in retinal pericytes. Microvasc Res. 2001;62:196–203.
- Chernyavsky AI, Nguyen VT, Arredondo J, Ndoye A, Zia S, Wess J, et al. The M4 muscarinic receptor-selective effects on keratinocyte crawling locomotion. Life Sci. 2003;72:2069–2073.
- Marchini G, Babighian S, Tosi R, Perfetti S, Bonomi L. Comparative study of the effects of 2% ibopamine, 10% phenylephrine and 1% tropicamide and the anterior segment. Invest Ophthalmol Vis Sci. 2003;44:281–289.
- Haefliger IO, Meyer P, Flammer J, Luescher TF. The vascular endothelium as a regulator of the ocular circulation: A new concept in ophthalmology?. Surv Ophth. 1994;39:123–132.
- Koss MC. Functional role of nitric oxide in the regulation of ocular blood flow. Eur J Pharm. 1999;374:161–174.
- Tutaj M, Brown CM, Brys M, Marthol H, Hecht MJ, Dutsch M, et al. Dynamic cerebral autoregulation is impaired in glaucoma. J Neurol Sc. 2004;220:49–54.
- Duncan G, Collison DJ. Role of the non-neuronal cholinergic system in the eye: A review. Life Sci. 2003;72: 2013–2019.
- Harazny JM, Ritt M, Baleanu D, Ott C, Heckmann J, Schlaich MP, et al. Increased wall:lumen ratio of retinal arterioles in male patients with a history of a cerebrovascular event. Hypertension. 2007;50:623–629.
- Ritt M, Harazny JM, Ott C, Schlaich MP, Schneider MP, Michelson G, et al. Analysis of retinal arteriolar structure in never-treated patients with essential hypertension. J Hypertens. 2008;26:1427–1434.
- Baleanu D, Ritt M, Harazny J, Heckmann J, Schmieder RE, Michelson G. Wall-to-lumen ratio of retinal arterioles and arteriole-to-venule ratio of retinal vessels in patients with cerebrovascular damage. Invest Ophthalmol Vis Sci. 2009;50: 4351–4359.
- Wong TY, Mitchell P. The eye in hypertension. Lancet. 2007;369:425–435.
- Choi YK, Kim KW. Blood–neural barrier: its diversity and coordinated cell-to-cell communication. BMB Rep. 2008;41: 345–352.
- Zinser G. Scanning-laser-Doppler-flowmetry. Principle and technique. Current concept on ocular blood flow in glaucoma. The Hague: Kugler Publications 1999. p. 197–204.
- Michelson G, Schmaus B, Langhans MJ, Harazny J, Groh MJM. Principle, validity and reliability of scanning laser Doppler flowmetry. J Glaucoma. 1996;5:99–105.
- Michelson G, Welzenbach J, Pal I, Harazny J. Automatic full field analysis of perfusion images gained by scanning laser Doppler flowmetry. Br J Ophth. 1998;82:1294–1300.
- Parati G, Lombardi C, Hedner J, Bonsignore MR, Grote L, Tkacova R, et al.; European Respiratory Society; EU COST ACTION B26 members. Position paper on the management of patients with obstructive sleep apnea and hypertension: joint recommendations by the European Society of Hypertension, by the European Respiratory Society and by the members of European COST (COoperation in Scientific and Technological research) ACTION B26 on obstructive sleep apnea. J Hypertens. 2012;30:633–646.
- Hoh ST, Greenfield DS, Liebmann JM, Hillenkamp J, Ishikawa H, Mistlberger A, et al. Effect of pupillary dilatation on retinal fibre layer thickness as measured by scanning laser polarimetry in eyes with and without cataract. J Glaucoma. 1999;8:159–163.
- Lanigan LP, Clark CV, Hill DV. Retinal circulation responses to systemic autonomic nerve stimulation. Eye. 1988;2:412–417.
- Robinson F, Petrig BL, Sinclair S, Riva C, Grunwald J. Does topical phenylephrine, tropicamide, or proparacaine affect macular blood flow. Ophhalmology. 1985;92:1130–1132.
- Baumann H, Chioralia G, Abu-Oun S. Behaviour of fluorescein dye in the vascularised cornea of the animal eye after local application of certain drugs. Albrecht Von Graefes Arch Klin Exp Ophthalmol. 1979;210:9–18.