Abstract
Aims. Diabetes mellitus is characterized by structural and functional alterations of the large- and medium-size arteries. Whether blood glucose variability, i.e. the glycemic oscillations occurring during the 24-h period, represents a risk factor for vascular alterations additional to and independent on HbA1c in type 1 diabetes mellitus is still undefined. The present study was carried out with the aim at investigating the impact of different measures of blood glucose variability on arterial structure and function. We studied 17 non-complicated type 1 diabetic patients (11 males, six females) with an age of 40.8 ± 7.6 years (mean ± SD). In each patient, 24-h glucose profile was obtained by continuous glucose monitoring system and glucose variability was expressed as mean ± SD of 24-h blood glucose levels, mean amplitude of glycemic excursions and postprandial hyperglycemic spikes. Arterial structure and function was measured as carotid IMT and stiffness. Major findings. The different approaches to assessing blood glucose variability well correlated between and with HbA1c. Carotid IMT and stiffness showed significant correlations with age, blood pressure, heart rate and daily insulin intake but a non- significant correlation with blood glucose variability. Principal conclusion. Thus, in type 1 diabetes mellitus, measures of glycemic variability are useful in predicting both actual and long-lasting glycemic control. In absence of diabetes-related complications and of any intima-media thickness alterations, the major predictors of arterial distensibility are represented by traditional risk factors beside glycemic 24-h control.
Introduction
Diabetes mellitus is characterized not only by microvascular and macrovascular complications, but also by structural and functional alterations of the large- and medium-size arteries, such as an increase in arterial wall thickness and stiffness (Citation1–4). These alterations are detectable in (i) young individuals with uncomplicated diabetes (Citation3); (ii) subjects with glucose intolerance not yet displaying diabetes but predisposed to it (Citation5,Citation6); and (iii) subjects with normal glycemic values but with a family history of diabetes (Citation7). The results of both the Diabetes Control and Complications Trial in type 1 diabetes and the UK Prospective Diabetes Study in type 2 diabetes left little doubt on the fact that the risk of microvascular complications rises exponentially as mean blood glucose values increase (Citation8,Citation9). On the contrary, the relationships existing between macroangiopathy and fasting plasma glucose or glycated hemoglobin (HbA1c) are far from being clearly defined (Citation10,Citation11). This may be due to a number of causes, including the fact that fasting plasma glucose levels or HbA1c alone are unable to thoroughly reflect the impact of glycemic disorders on the cardiovascular system in diabetic patients.
Whether blood glucose variability, i.e. the glycemic oscillations occurring during the 24-h period, represents a risk factor for complications additional to and independent of HbA1c in both type 1 and type 2 diabetes is still undefined. Indeed, blood glucose variability displays an adverse prognostic impact in critical care diabetic and non-diabetic patients (Citation12) and may exert a pathophysiological role in the development of diabetic vascular complications. The data collected so far, however, on the impact of blood glucose variability on diabetic-related vascular alterations are not univocal. This is the case for the microvascular (Citation13–19) and, to a greater extent, for the macrovascular complications of the diabetic state (Citation20–22).
The aims of the present study carried out in type 1 young diabetic subjects free of micro- and macro-vascular complications were the assessment of (i) the value of different measures of blood glucose variability and (ii) the impact of anthropometric, hemodynamic as well as metabolic variables (including blood glucose variability) on carotid artery thickness and distensibility. Blood glucose values were measured throughout 24-h period via a continuous glucose monitoring system, allowing the collection of about 500 glycemic values in each single subject. Variability was assessed as the mean blood glucose standard deviation (SD) and mean amplitude of glycemic excursions, thus overcoming the methodological limitations of previous studies that based variability assessment on seven point blood glucose measurements only. Carotid artery structure was assessed as intima-media thickness, and carotid artery function as arterial distensibility, i.e. two well-established early markers of the atherogenic vascular process (Citation23–28).
Materials and methods
Subjects
We investigated 17 type 1 diabetic patients (11 males, six females) with an age amounting to 40.8 ± 7.6 years (mean ± SD). We selected them on a consecutive basis if they displayed (i) a type I diabetes mellitus receiving insulin treatment in absence of any diabetic-related complication (i.e. microalbuminuria, retinopathy and/or neuropathy excluded by dipstick test, fundus oculi examination and electromyography); (ii) no evidence of clinical or subclinical atherosclerotic disease in their history or at physical and laboratory examination (such as standard ECG, echocardiogram and echocolor-Doppler of carotid arteries and lower limbs); (iii) no major metabolic or cardiovascular disease besides diabetes; and (iv) no hypertrigliceridemia and hypercholesterolemia. With the exception of two subjects, no patient was a cigarette smoker. All subjects agreed to participate in the study after being informed of its nature and purposes. The protocol of the study was approved by the ethics committees of the institutions involved.
Continuous blood glucose monitoring
To assess 24-h glucose profile we used the continuous glucose monitoring system GlucoDay (CGM, Azienda Menarini Diagnostics, Florence, Italy). The microdialysis GlucoDay sensor was calibrated according to the manufacturers’ instructions, and its clock was adjusted to match a master clock in the room, which allowed for further synchronization of the data. A microdialysis fiber (Medica, Medolla, Modena, Italy) was inserted subcutaneously into the periumbelical region, without local anesthesia, using an 18-gauge Teflon catheter as a guide. The fiber was then connected to a portable 245-g apparatus, powered by a 9-volt battery. Glucose oxidase (sensor) and two plastic bags (one for the buffer reservoir and one for waste products) complete the apparatus, which is contained in a small wearable pouch. A perfusion buffer (Dulbecco's solution, containing sodium benzoate as preservative) circulates at a rate of 10 μl/min by means of a programmable peristaltic pump (Citation29). Glucose concentrations in the dialysate were then measured every 3 min by the glucose sensor over a 24-h period. After the 24-h monitoring period, data were downloaded into a computer. Nanoampere values reported by the GlucoDay device were converted into glucose values after two-point calibration using glucose values obtained by reflectometric postprandial automeasurements.
Carotid artery distensibility and carotid artery wall thickness
With the subject supine and the neck in partial extension, the diameter and wall motion of the right common carotid artery were measured 2 cm below the carotid bifurcation by a B-M mode echo-tracking device based on Doppler shift (Wall Track System, PIE Medical Imaging and Esaote, Maastricht, The Netherlands and Florence Italy) and on a transducer operating at a frequency of 7.5 MHz (Citation30,Citation31). The transducer was manually oriented perpendicularly to the longitudinal axis of the vessel under B-mode guidance. After switching to A-mode, the backscattered echoes from the anterior and posterior carotid artery walls were made visible on a screen and the corresponding radiofrequency signal was tracked by electronic tracers to allow the digitalized signal of the internal diameter variations to be derived at 50 Hz. The spatial resolution was 300 μm. Carotid pulse pressure was measured by a modified tonometer (pulse pen, see below) at the same time of the ultrasound evaluation, and carotid artery distensibility was derived according to the following formulae:
where Dist represents distensibility, ΔD systo- diastolic diameter change, Dd diastolic diameter of the vessel and ΔP the corresponding pulse pressure value (Citation30).
Carotid artery intima-media wall thickness was measured at a posterior wall site located 3 cm below bifurcation through an ultrasonographic device (Philips Sonos 5500, Philips, Andover, Massachusetts, USA)). Measurements were obtained by first scanning the artery in B-mode, then freezing the digitized image in M-mode and finally tracking the inner ipoechogenic and the middle anechogenic layers (Citation32). Measurements were performed by two skilled operators (CF and NS) unaware of the experimental design of the study. The within-operator and interoperator variabilities of the carotid artery diameter measurements at diastole (i.e. the coefficient of variation of the mean values of two measurements done at two different times) in our laboratory were 2.5% and 3.5%, respectively. The within-operator and interoperator variabilities for the carotid artery wall thickness were 3.0% and 4.0%, respectively.
Additional measurements
Blood pressure was measured not only by the semi-automatic AeD device (see above), but also, with the patient supine, by a mercury sphygmomanometer, taking the first and fifth Korotkoff sounds to identify systolic and diastolic values, respectively. In each subject, the average of three measurements was taken as the mean value. To calculate carotid artery distensibility, blood pressure was further measured by a modified tonometric device (Pulse Pen, DiaTecne, Milan, Italy) positioned at the level of the carotid artery. Brachial sphygmomanometric blood pressure was used to calibrate the absolute value. Heart rate values were obtained via palpatory method (radial artery) over a 30-s period. Waist and hips circumferences were measured in centimeters with the subject in standing position, and body mass index was derived from the formulae weight (kilograms)/height (squared meters). Glycated hemoglobin values were also measured from a venous blood sample within the week preceding the study.
Protocol and data analysis
Patients were invited to come to the outpatient clinic of the San Gerardo Hospital, Monza, Italy, in the morning after a 24-h abstinence from alcohol, caffeine consumption and cigarette smoking. The GlucoDay device was fitted to the patient (see above). The following day the device was then removed and additional measurements were performed as follows: (i) blood pressure was measured three times by a mercury sphygmomanometer with the patient in the sitting position; (ii) the subject was placed in the supine position, the tonometric blood pressure measuring device was placed on the carotid artery and the pulse pressure to be used for carotid artery distensibility calculation obtained; (iii) the probe for carotid artery evaluation was positioned on the neck and five 6-s acquisitions of carotid diameter throughout the cardiac cycle were obtained during 10 min; (iv) the wall track probe was removed and carotid artery intima-media wall thickness was assessed by the standard echocolor Doppler technique, and the three sphygmomanometric blood pressure values were averaged. Carotid artery diastolic diameter and distensibility were obtained by averaging data from the five 6-s acquisition periods. The 24-h glycemic values of glycemia were also averaged. Carotid artery wall thickness was measured on the screen image of the vessels over a 30-s period, based on the ultrasound image. After the 24-h monitoring period, the glucose curves were manually inspected to verify that sensors were active and all curves were qualitatively and quantitatively consistent with each other. To describe glucose variability in the 24-h period, we calculated the mean± SD. As additional data, the mean amplitude of glycemic excursions was calculated with a specific software for 10 patients (Citation33). Finally, the glycemic excursions were based on patient's information and correlated by the GlucoDay register. The hyperglycemic spike was considered as the higher glycemic value within the 2 h following the meal minus the baseline value before insulin injection preceding the meal as described in previous studies (Citation34). For each patient, spikes were calculated after the three principal meals according to their diaries.
Data analysis was performed using SAS software for data management and statistical analyses (SAS Institute Inc., Cary, North Carolina, USA). The Shapiro–Wilk test was employed to test for normality in distribution of each variable. When variables were found not normally distributed, non-parametric analysis was used and data were logarithmically transformed. The majority of our data was then found to be not normally distributed. Descriptive statistic was performed, comprehensive of calculation of mean, median, SD, interquartile range. Spearman's and or Pearson correlation coefficient (r) was calculated to evaluate the relationships between intima media thickness, carotid artery distensibility and blood glucose variables. Partial correlation for age and systolic blood pressure was calculated applying the Cholesky decomposition algorithm to the Spearman rank-order correlation matrix and using the correlation formula. Data are shown as means ± SD. A value of p < 0.05 was considered statistically significant.
Results
As shown in , in the population of our study heart rate and systo-diastolic blood pressure were in the normal range. This was the case also for plasma triglycerides and total cholesterol. Mean abdominal circumference and body mass index were in the high range, indicating that several subjects were overweight or had visceral obesity. In contrast, fasting blood glucose and HbA1c were, as expected, greater than the reference values (). This was the case also for the mean of blood glucose values (around 500 for each patient) recorded by the GlucoDay. Fasting blood glucose was slightly greater than the mean blood glucose values observed throughout the 24 h, the difference not achieving, however, statistical significance (147.2 ± 72 vs 142.3 ± 52 mg/dl, p = NS). also shows the mean values of the different glucose variability parameters assessed in the present study, i.e. mean ± SD of 24-h blood glucose levels, mean amplitude of glycemic excursions, and postprandial hyperglycemic spikes, as well as the data related to carotid artery wall thickness and distensibility in the 17 uncomplicated type 1 diabetes mellitus patients of the present study.
Table I. Demographic and hemodynamic findings in type I diabetic patients without microvascular complications enrolled in the study.
Table II. Characteristics of blood glucose metabolism and of carotid artery in the 17 type I diabetics patients enrolled in the study.
illustrates Pearson's correlation coefficients of the relationships between right carotid intima thickness, carotid artery distensibility, and anthropometric, hemodynamic and metabolic parameters. As expected, we found significant correlations between carotid distensibility and age, blood pressures and heart rate, while intima media thickness showed a non-significant trend in the same direction. Considering the different parameters of glucose metabolism, we observed a significant correlation only between carotid distensibility and daily insulin intake. In contrast, the SD of the glycemic values, taken as a marker of blood glucose variability, did not show any significant relationship with both right carotid intima media thickness and carotid artery distensibility values. This was the case also for the mean amplitude of glycemic excursion values assessed in 10 patients. When the data were adjusted for age, only the correlation between carotid distensibility and heart rate remained statistically significant (r = − 0.60, p = 0.01).
Figure 1. Pearson's correlation coefficients of the relationships between right carotid intima-media thickness, carotid artery distensibility and anthropometric, hemodynamic and metabolic parameters. SBP, systolic blood pressure; DBP, diastolic blood; TC, total cholesterol; HR, heart rate; PPHS, postprandial hyperglycemic spikes; DMD, diabetes mellitus duration; FBG, fasting blood glucose; 24h-BG, mean blood glucose of the 24 hours; BG-SD, blood glucose standard deviation; MAGE, mean amplitude of glucose excursion.
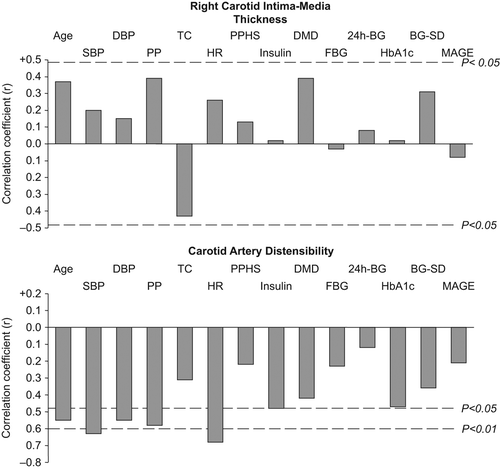
shows the correlation coefficients of the relationships between the two parameters of blood glucose variability, i.e. blood glucose SD and mean amplitude of glycemic excursions, with other parameters of blood glucose metabolism. They were both related to the mean glycemic values assessed during the 24-h period, while only mean blood glucose SD showed a significant correlation with HbA1c. The two measures of variability were significantly related to each other (). Insulin treatment and fasting blood glucose showed a tendency to correlate with mean blood glucose SD, while the mean amplitude of glycemic excursion appeared to be more related (even if not achieving statistic significance) to fasting blood glucose values.
Figure 2. Pearson's correlation coefficients of the relationships between blood glucose standard deviation and mean amplitude of glucose excursion with other parameters of blood glucose metabolism. DMD, diabetes mellitus duration; FBG, fasting blood glucose; 24h-BG, mean blood glucose of the 24 hours; PPHS, postprandial hyperglycemic spikes; MAGE, mean amplitude of glucose excursion; BG-SD, blood glucose standard deviation.
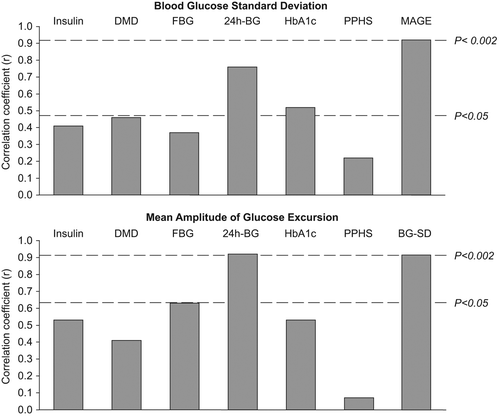
Discussion
The present study provides two sets of new information related on one side to the methodological approaches to assess glucose variability in diabetic patients and on the other to the relationship between glucose abnormalities and the functional and structural alterations of large arteries in diabetic patients. These two sets of information will be separately discussed thereafter.
As far as the first issue is concerned, our data show that two different measures of blood glucose variability such as the mean amplitude of glucose excursion and the mean blood glucose SD well correlate each other. They also show that both these two parameters were highly and significantly related to mean 24-h blood glucose levels, thus reflecting the whole day glycemic control. Finally both mean blood glucose SD and mean amplitude of glucose excursion show an elevated Pearson correlation coefficient when plotted against HbA1c (r = 0.52 and r = 0.53, respectively). The correlation value, however, achieved statistical significance only for mean blood glucose SD presumably because of the greater number of patients in which this variable was assessed compared with the mean amplitude glucose excursion (n = 17 vs n = 10). Thus both these two indices of glycemic variability represent either the day by day or the long-lasting metabolic control of blood glucose in type 1 diabetes mellitus.
Our study also provides information on the relationships between anthropometric data, hemodynamic values as well as glucose abnormalities and the functional and structural alterations of the large arteries in diabetic patients. First, in our group of normotensive, non-microalbuminuric, uncomplicated type 1 diabetes mellitus patients, the most relevant variable affecting the structure and the function of the carotid artery was, as found in the general population, subjects’ age (Citation35,Citation36). Second, the quite high correlation coefficient values observed between diabetes duration and both intima media thickness and distensibility found in the present study confirms the role of diabetes mellitus as a promoter of the adverse effects that the ageing process has on vascular structure and function (Citation37). Third, besides age, hemodynamic factors, such as systolic, diastolic and pulse pressure, highly predicted carotid artery distensibility values. However, because arterial distensibility is calculated by a formula that makes use of pulse pressure values in its determination, this significant correlation may represent simply a mathematical consequence (Citation30). Fourth, arterial distensibility significantly correlated with baseline resting heart rate and this correlation was detectable after data adjustment for age in a multivariate analysis. Heart rate is an early marker of diabetic disautonomia and sympathetic activation (Citation38,Citation39). Arterial distensibility is a very early marker of arterial function and cardiovascular damage in both type 1 and type 2 diabetic patients (Citation5,Citation7). Arterial distensibility and arterial function have been shown to be affected by heart rate both because an increased heart rate reduces the recovery time of the viscoelastic components of arterial wall (Citation40) and because wall distention is under sympathetic control both in healthy and diseased subjects (Citation41,Citation42). In the highly selected type 1 diabetes mellitus patients of the present study, arterial distensibility exhibited only spurious and not significant correlations with the different indices of glycemic status and metabolic control. Moreover, among the various indices of glycemic control taken into account in our study, HbA1c showed a correlation coefficient value (0.47) that, although not achieving statistical significance, was in any case greater than that displayed by 24-h mean glycemic values, fasting blood glucose and glycemic variability considered parameters. This supports the hypothesis that long-lasting glycemic control is of major importance also for arterial function determination (Citation19). Finally, insulin U/day value was significantly and inversely related to carotid artery distensibility, a finding suggesting that glycemic control is obtained with a prevalence of negative effects of insulin on arterial wall (Citation43).
Our study has some strengths as well as a limitation. The strengths refer to the fact that our diabetic type 1 subjects were highly selected to exclude patients with vascular complications; moreover, functional and structural carotid artery evaluation as well as 24-h glycemic control were accurately performed. Our study also has a major limitation, which consists in the fact that the relative small number of enrolled subjects precluded multiple regression analysis. It is thus possible that a larger number of subjects would allow stronger conclusions on the relationship between structure and function of arterial vessels and glycemic variability.
In conclusion, our data provide evidence that in type 1 diabetes mellitus measures of glycemic variability are useful in predicting both actual and long-lasting glycemic control. Their clinical importance is therefore further emphasized. In absence of diabetes-related complications and of any intima-media thickness alteration, the major predictors of arterial distensibility are represented by age, blood pressure, heart rate and insulin treatment daily dosage.
Conflicts of interest
None.
Sources of funding
None.
References
- Stamler J, Vaccaro O, Neaton JD, Wentworth D. Diabetes, other risk factors, and 12-yr cardiovascular mortality for men screened in the Multiple Risk Factor Intervention Trial. Diabetes Care. 1993;16:434–444.
- Nathan DM. Long term complications of diabetes mellitus. N Eng J Med. 1993;328:1676–1685.
- Mancia G, Giannattasio C. Structural arteriolar changes in diabetes mellitus. Eur Heart J. 1997;18:1049–1050.
- Kool MJ, Lambert J, Stehower CD, Hoeks AP, Struijker-Boudier HA, et al. Vessel wall properties of large arteries in uncomplicated IDDM. Diabetes Care. 1995;18:618–624.
- Giannattasio C, Failla M, Piperno A, Grappiolo A, Gamba P, Paleari F, et al. Early impairment of large artery structure and function in type I diabetes mellitus. Diabetologia. 1999;42:987–994.
- Giannattasio C, Failla M, Grappiolo A, Gamba PL, Paleari F, Mancia G., Progression of large artery structural and functional alterations in type I diabetes. Diabetologia. 2001;44:203–208.
- Giannattasio C, Failla M, Capra A, Scanziani E, Amigoni M, Boffi L, et al. Increased arterial stiffness in normoglycemic normotensive offspring of type 2 diabetic parents. Hypertension. 2008;51:182–187.
- The DCCT Research Group: The relationship of glycemic exposure (HbA1c) to the risk of development and progression of retinopathy in the Diabetes Control and Complications Trial.Diabetes. 1995;44:968 –983.
- Stratton IM, Adler AI, Neil HA, Matthews DR, Manley SE, Cull CA, et al. Association of glycaemia with macrovascular and microvascular complications of type 2 diabetes (UKPDS 35): Prospective observational study. BMJ. 2000;321: 405–412.
- Laakso M, Hyperglycemia and cardiovascular disease in type 2 diabetes. Diabetes. 1999;48:937–942.
- Orchard TJ,Olson JC, Erbey JR, Williams K, Forrest KY,Smithline Kinder L, et al. Insulin resistance related factors, but not glycemia, predict coronary artery disease in type 1 diabetes: 10-year follow-up data from the Pittsburgh Epidemiology of Diabetes Complications Study. Diabetes Care. 2003;26:1374–1379.
- Capes SE, Hunt D, Malmberg K, Gerstein HC. Stress hyperglycaemia and increased risk of death after myocardial infarction in patients with and without diabetes: A systematic overview. Lancet. 2000;355:773–778.
- Monnier L, Mas E, Ginet C, Michel F, Villon L, Cristol JP, et al. Activation of oxidative stress by acute glucose fluctuations compared with sustained chronic hyperglycemia in patients with type 2 diabetes. JAMA. 2006;295:1681–1687.
- Mohsin F, Craig ME, Cusumano J, Chan AKF, Hing S, Lee JW, et al. Discordant trends in microvascular complications in adolescents with type 1 diabetes from 1990 to 2002. Diabetes Care. 2005;28:1974–1980.
- Brownlee M, Hirsch IB. Glycemic variability: A hemoglobin A1c-independent risk factor for diabetic complications. JAMA. 2006;295:1707–1708.
- Service FJ, O’Brien PC. The relation of glycaemia to the risk of development and progression of retinopathy in the Diabetic Control and Complications Trial. Diabetologia. 2001; 44:1215–1220.
- Kilpatrick ES, Rigby AS, Atkin SL. The effect of glucose variability on the risk of microvascular complications in type 1 diabetes. Diabetes Care. 2006;29:1486–1490.
- Kilpatrick ES, Rigby AS, Atkin SL. Effect of glucose variability on the long-term risk of microvascular complications in type 1 diabetes. Diabetes Care. 2009;32:1901–1903.
- Kilpatrick ES, Rigby AS, Atkin SL. A1C variability and the risk of microvascular complications in Type 1 diabetes: Data from the Diabetes Control and Complications Trial. Diabetes Care. 2008;31:2198–2202.
- Muggeo M, Verlato G, Bonora E, Zoppini G, Corbellini M, De Marco R. Long-term instability of fasting plasma glucose: A novel predictor of cardiovascular mortality in elderly patients with non-insulin-dependent diabetes mellitus: The Verona Diabetes Study. Circulation. 1997;96:1750–1754.
- Muggeo M, Zoppini G, Bonora E, Brun E, Bonadonna RC, Moghetti P, et al. Verlato G, Fasting plasma glucose variability predicts 10-year survival of type 2 diabetic patients from the Verona Diabetes Study. Diabetes Care. 2000;23:45–50.
- Ceriello A. Postprandial hyperglycemia and diabetes complications: Is it time to treat?Diabetes. 2005;54:1–7.
- Bots ML, Hoes AW, Koudstaal PJ, Hofman A, Grobbee DE. Common carotid intima-media thickness and risk of stroke and myocardial infarction: The Rotterdam Study. Circulation. 1997;96:1432–1437.
- Wofford JL, Kahl FR, Howard GR, McKinney WM, Toole JF, Crouse JR. Relation of extent of extracranial carotid artery atherosclerosis as measured by B-mode ultrasound to the extent of coronary atherosclerosis. Arterioscler Thromb. 1991;11:1786–1794.
- O’Leary DH, Polak JF, Kronmal RA, Manolio TA, Burke GL, Wolfson SK Jr. Carotid artery intima and media thickness as a risk factor for myocardial infarction and stroke in older adults. N Engl J Med. 1999;340:14–22.
- Vlachopoulos C, Aznaouridis K, Stefanadis C. Prediction of cardiovascular events and all-cause mortality with arterial stiffness: A systematic review and meta-analysis. J Am Coll Cardiol. 2010;55:1318–1327.
- Laurent S, Katsahian S, Fassot C, Tropeano AI, Laloux B, Boutouyrie P. Aortic stiffness is an independent predictor of fatal stroke in essential hypertension. Stroke. 2003;34: 1203–1206.
- Boutouyrie P, Tropeano AI, Asmar R, Gautier I, Benetos A, Lacolley P, et al. Aortic stiffness is an independent predictor of primary coronary events in hypertensive patients: A longitudinal study. Hypertension. 2002;39:10–15.
- Maran A, Poscia A. Continuous subcutaneous glucose monitoring: The Glucoday system. Diabetes Nutr Metab. 2002;15:429–433.
- Kool MJF, Van Merode T, Reneman RS, Hoeks APG, Struyker Boudier H, Van Bortel LMAB. Evaluation of reproducibility of a vessel wall movement detector system for assessment of large artery properties. Cardiovasc Res. 1994;28:610–614.
- Laurent S, Cockcroft J, Van Bortel L, Boutouyrie P, Giannattasio C, Hayoz D, et al. European Network for Noninvasive Investigation of Large Arteries. Methodological issues and clinical applications. Eur Heart J. 2006;27:2588–2605.
- Salonen R, Haapdnen A, Salonen JT. Measurement of intima-media thickness of common carotid arteries with high- resolution B-mode ultrasonography: Inter- and intra-observer variability. Ultrasound Med Biol. 1991;17:225–230.
- Service FJ, Molnar GD, Rosevear JW, Ackerman E, Gatewood LC, Taylor WF. Mean amplitude of glycemic excursions, a measure of diabetic instability. Diabetes. 1970;19:644–655.
- Bonora E, Muggeo M. Postprandial blood glucose as a risk factor for cardiovascular disease in Type II diabetes: The epidemiological evidence. Diabetologia. 2001;44:2107–2114.
- Lee HY, Oh BH. Aging and arterial stiffness. Circ J. 2010;74:2257–2262.
- Bhuiyan AR, Srinivasan SR, Chen W, Paul TK, Berenson GS. Correlates of vascular structure and function measures in asymptomatic young adults: The Bogalusa Heart Study. Atherosclerosis. 2006;189:1–7.
- Stehouwer CDA, Henry RMA, Ferreira I. Arterial stiffness in diabetes and the metabolic syndrome: A pathway to cardiovascular disease. Diabetologia. 2008;51:527–539.
- Pfeifer MA, Weinberg CR, Cook DL, Reenan A, Halter JB, Ensinck JW, et al. Autonomic neural dysfunction in recently diagnosed diabetic subjects Diabetes Care. 1984;7:447–453.
- Vinik AI, Ziegler D. Diabetic cardiovascular autonomic neuropathy. Circulation. 2007;115:387–397.
- Mircoli L, Mangoni AA, Giannattasio C, Mancia G, Ferrari AU. Heart rate-dependent stiffening of large arteries in intact and sympathectomized rats. Hypertension. 1999; 34:598–602.
- Failla M, Grappiolo A, Emanuelli G, Vitale G, Fraschini N, Bigoni M, et al. Sympathetic tone restrains arterial distensibility of 111 healthy and atherosclerotic subjects. J Hypertens. 1999;17:1117–1123.
- Grassi G, Giannattasio C, Failla M, Pesenti A, Peretti G, Marinoni E, et al. Sympathetic modulation of radial artery compliance in congestive heart failure. Hypertension. 1995;26:348–354.
- Hsueh WA, Law RE. Insulin signalling in the arterial wall. Am J Cardiol. 1999;84:21J–24J.