Abstract
Introduction. The accuracy of impedance cardiography for cardiac index assessment is matter of debate, with available studies reporting inconsistent results. Our study aimed at evaluating the agreement between measurements of cardiac index provided by a new-generation thoracic electrical bioimpedance device (Hotman System) and an invasive approach based on thermodilution in humans. Methods. Cardiac index was assessed simultaneously with thoracic electrical bioimpedance and conventional thermodilution through comparison of five consecutive measurements in 51 cardiac patients, hospitalized in an intensive care unit (mean± SD age: 60 ± 11 years; 68% males). The agreement between cardiac index values measured by both methods was assessed by the Bland–Altman approach, adjusted for repeated measures. The repeatability coefficient and the intraclass correlation coefficient were used to assess reproducibility of replicates. Results. Average (± SD) cardiac index was 3.05 ± 0.91 l/min/m2 with Hotman System and 3.14 ± 1.12 l/min/m2 with thermodilution. The bias of precision was −0.09 ± 0.41. The coefficients of repeatability and intraclass correlation coefficients were high and similar for the two techniques (0.95 l/min/m2 and 0.91 for Hotman System vs 0.78 l/min/m2 and 0.90 for thermodilution). Conclusions. Cardiac index values yielded by Hotman system compares favorably with that obtained with thermodilution in cardiac patients.
Introduction
Critically ill patients often require continuous cardiac function assessment, and cardiac output monitoring represents an important diagnostic and prognostic tool in these conditions (Citation1). The thermodilution technique is the clinical standard for cardiac output estimation, but it requires an invasive and costly procedure, not free from complications (Citation2–5). An alternative to such an approach might be the use of impedance cardiography or thoracic electrical bioimpedance. This technique is based on recording of changes in the electrical resistance of the chest during heartbeat: these variations are then converted to changes in volume over time and used to derive stroke volume (Citation6–9). Compared with thermodilution, impedance cardiography allows hemodynamic measurements to be carried out more easily and quickly, and without the risk of infection or other complications associated with pulmonary artery catheterization (Citation10). In addition, unlike thermodilution, the bioimpedance technique enables monitoring of cardiac output in a continuous mode at the patient's bedside, with a low cost of testing (Citation10). Since its introduction several years ago, impedance cardiography has demonstrated its usefulness in various populations and conditions (Citation10). However, a number of validation studies suggest a poor correlation between data provided by most impedance devices and invasive measurements of cardiac outputs and a wide range of variability in the agreement between the two methods for the different devices available on the market (Citation6,Citation9,Citation11–14). This evidence has questioned the reliability of bioimpedance for hemodynamic monitoring of critically ill patients, and has hindered the broad adoption of this technique as a tool for managing cardiac patients, limiting its diffusion mainly to physiological studies or perioperative applications (Citation1).
Recently, attempts have been made to provide more reliable non-invasive measurements of hemodynamic parameters through a very low current thoracic electrical bioimpedance device (Hotman System, Hemo Sapiens Medical Inc., Sedona, AZ, USA), making use of a more accurate algorithm for the calculation of cardiac output (Citation15). Preliminary evidence exists on the ability of such device to yield a reproducible non-invasive estimation of cardiac output in healthy volunteers, but no data on its clinical accuracy is yet available (Citation16).
The purpose of this study was thus to investigate the agreement between measurements of cardiac output simultaneously provided at rest by a reference invasive measuring methodology (thermodilution) and by the Hotman non-invasive electrical bioimpedance device in patients hospitalized in an intensive care unit.
Materials and methods
Study design and population
This was a non-randomized, multicenter, national study, involving two centers located in Romania. The study was designed to compare the accuracy of the cardiac output determination by a thoracic electrical bioimpedance system (Hotman System, Hemo Sapiens Medical Inc., Sedona, Arizona, USA) (Citation15) versus that obtained with pulmonary artery catheter standard bolus thermodilution, used as reference gold standard. The study was performed in 51 cardiac patients of either gender, aged 18–72 years, hospitalized in the intensive care units of Institutul de Urgenta pentru Boli Cardiovasculare “Prof. Dr. C.C. Iliescu” (n = 44) and Spitalul Militar de Urgenta “Prof. Dr. Agrippa Ionescu” (n = 7), Bucharest, Romania. Patients were included in the study if there was a clinical indication for pulmonary artery catheterization (patients undergoing cardiac surgery or a cath lab test), and following initial treatment and stabilization in the critical care unit.
Patients could not be enrolled in the study if meeting at least one of the following exclusion criteria: (i) ventricular or dual chamber pacemaker wearers; (ii) presence of a severe aortic insufficiency; (iii) patients with left to right shunt; (iv) patients with terminal illness; (v) extremely obese patients (body mass index > 35 kg/m2); (vi) patients displaying a high level of anxiety; (vii) patients undergoing hemodialysis, ultrafiltration, mechanical ventilation with continuous positive airway pressure, or life-saving treatments other than mechanical ventilation, or who were using a left ventricular assist device (including intra-aortic balloon pump); (viii) previously enrolled subjects.
The study was approved by the local ethics committee of each center. All participants provided a written informed consent prior to any study procedure.
Patients were studied in the immediate post- operative period (all patients had been subjected to open heart surgery, 33 patients with coronary artery bypass graft and 18 with valvular problems, aortic and/or mitral), and cardiac output was determined at the arrival in the cardiac intensive care unit. Assessment was done in the supine position, simultaneously by thermodilution and non-invasive thoracic electrical bioimpedance: five consecutive determinations at 3-min intervals were scheduled in each subject. The investigators had to start the recording of non-invasive hemodynamics each time a bolus was injected for the thermodilution measurement.
Transpulmonary thermodilution
Thermodilution data were obtained by the fluid bolus technique (Citation2,Citation4). A 7.5 French pulmonary artery catheter (Swan–Ganz) was introduced into the right internal jugular vein and advanced to the pulmonary artery through the right heart. A 10-ml bolus of ice-cold 5% dextrose was injected in less than 4 s in the right atrium. According to the thermodilution principle, the change in temperature detected in the blood of the pulmonary artery by a thermistor positioned at the end of the catheter was used to calculate cardiac output, using the modified Stewart–Hamilton indicator dilution equation. In order to improve the accuracy of the determination, each measurement was based on the average of three repeated passes, all within 10% of each other, by excluding the first injection. In total, five consecutive measurements within a 3-min interval were performed. Measurements with more than 10% variation compared with the average of all five measurements had to be excluded, being considered errors given by the injection time.
Non-invasive thoracic electrical bioimpedance
Cardiac output was determined also non-invasively by a novel device based on the thoracic electrical bioimpedance method (Hotman System) (Citation15). The system allows a complete non-invasive assessment of patient's hemodynamic with two important improvements compared with other previous similar devices: (i) use of a very low current (7 μA, 300–400-fold lower than that used by other products, making it safer for the patient) with a current frequency of 65 kHz, and (ii) use of a new data signal processing and of an improved mathematical algorithm. The Hotman system consists of a patient interface for automatic acquisition of blood flow and left ventricular parameters through electrodes positioned on the patient's chest and of a computer equipped with analysis software. Measured hemodynamics can be integrated by entering in the software additional information such as the upper arm blood pressure and the oxygen saturation level, which are processed by the software with calculation of additional parameters. Measurement of hemodynamic parameters is pursued through generation of a very low current circulating between two pairs of solid gel electrodes, one pair located in the upper part of the laterocervical region (root of the neck) and the other at the level of the upper abdomen (midaxillary line at the level of the xiphoid process): these are the “current electrodes” (). As the alveoli are filled with air (non-conducting medium), the electrical current is conducted mainly through the thoracic aorta and the venae cavae. Other four electrodes (“measuring electrodes”) are placed in close proximity to the current electrodes as shown in : they record the electrocardiogram as well as the voltage of the electrical current that crosses the thorax. This current is proportional to the thoracic impedance, namely to the tissue resistance when crossed by the electrical current. As this current is directed by the blood flow through the venae cavae and the thoracic aorta, and since the blood is the best electrical conductor of the human body, blood flow variations may be translated in variations of the thoracic impedance.
Figure 1. Illustration of the electrode arrays for the thoracic bioimpedance system used in the validation study. Position of current injecting electrodes and voltage measuring electrodes is reported.
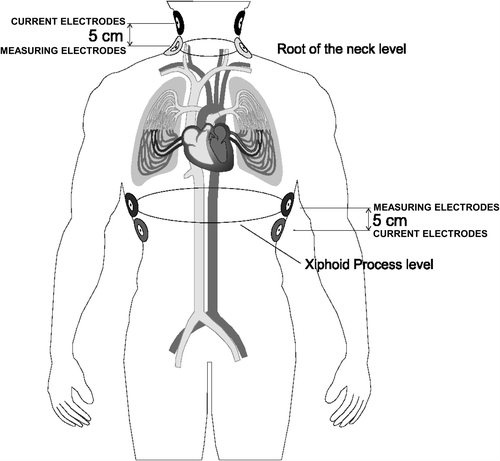
For the purpose of the validation study, because of the presence of the pulmonary artery catheter over the right internal jugular vein, the upper right-sided sensor of the neck was positioned behind the right ear lobe and the upper left-sided sensor, just before the left ear lobe. Before applying the electrodes, the skin was carefully cleaned with alcohol, to ensure good adhesion of electrodes and low skin-to-sensor impedance. The electrodes were then connected through a patient cable to the bioimpedance system. Prior to starting hemodynamic measurements, height and weight values were entered in the system and body surface area automatically computed, in order to be used for determination of cardiac indexes for both non-invasive and invasive methods. The non-invasive blood flow measurement was assessed concomitantly with the thermodilution measurement and cardiac index automatically calculated.
Data analysis
The parameter evaluated during the study and compared between methods was the cardiac index, defined as the ratio between cardiac output and body surface area and expressed in l/min/m2. Body surface area was calculated by the Du Bois & Du Bois formula (Citation17).
The study specifically aimed at assessing the accuracy of the non-invasive method and the repeatability of the measurements.
The agreement between cardiac index values measured by thermodilution and thoracic bioimpedance was assessed by calculating the mean between-method difference (bias or accuracy), and the standard deviation (SD) of the difference and the 95% confidence limits of agreement (precision) as described by Bland & Altman (Citation18,Citation19). For each patient and for each single measurement, the difference between the cardiac index detected by the thermodilution method (reference) and by the test device (thoracic electrical bioimpedance), as well as the average of the cardiac index value obtained with the two methods, were computed. Agreement between the reference and test device was checked by Bland–Altman graphs of reference-test device differences plotted vs average of the two methods, by considering the average of five measurements obtained in each individual (Citation18,Citation19). Percentage error, defined as the ratio between the limits of agreement of the bias and mean reference cardiac index for the two methods together, multiplied by 100, was calculated for interchangeability of the two methods according to the criterion described by Critchley & Critchley (Citation13). Acceptance of a new technique should rely on a percentage error of up to ± 30% (Citation13). Kendall's rank correlation tau (τ) test was used to seek a possible relation between average values and between-method differences.
Repeatability of replicated measurements for each method was also assessed by calculating the within-subject SD of the five replicates and then the repeatability coefficient, defined by 1.96×√2 multiplied by the within subject SD (Citation18,Citation20). The repeatability coefficient represents the value below which the absolute difference between two single test results may be expected to lie with a 95% probability (Citation20). In addition to the repeatability coefficient, the intraclass correlation coefficient was calculated in order to assess agreement between methods over repeated measurements (Citation21). The intraclass correlation coefficient is measured on a scale of 0 to 1, where 1 represents perfect reliability with no measurement error and 0 indicates no reliability. Wherever it was deemed necessary, a correction for repeated measured was applied, as required by the local calculation.
Results
A total of 51 cardiac patients were studied, 68% of which were males. The patients’ mean age (± SD) was 60 ± 11 years (range 28–72). Mean cardiac index (± SD) was 3.05 ± 0.91 l/min/m2 by the thermodilution method and 3.14 ± 1.12 l/min/m2 by bioimpedance. The correlation between the subject mean values showed a strong relationship (τ = 0.71; p < 0.0001) between the two methods (). The correlation coefficient within subjects was 0.97; this value showed that an increase in thermodilution indices within individual was associated with increase in bioimpedance indices.
Figure 2. Plot of the 255 pairs of cardiac index (CI) estimation derived by bioimpedance (y-axis) and thermodilution technique (x-axis). The continuous line refers to the line of complete agreement (identity).
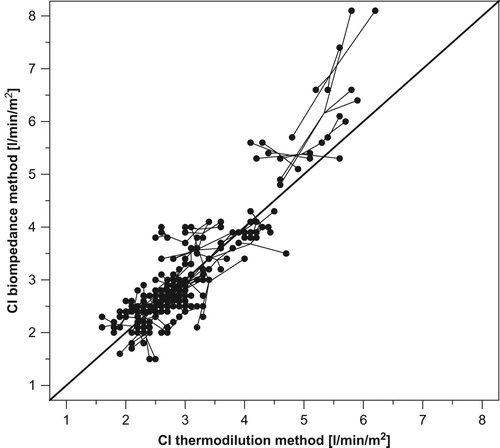
The mean thermodilution–bioimpedance difference was − 0.09 l/min/m2. The relative error of the bioimpedance method was 26%, namely less than the 30% maximum acceptable threshold (Citation13). , panel A, shows the Bland–Altman graph for comparison of cardiac index obtained by the two methods. The limits of agreement using repeated measurements (− 1.21 and 1.03) were small, suggesting that the test method might be reasonably accurate. The 95% confidence interval for the limits of agreement was also small: − 1.41 to − 1.01 for the lower limit of agreement and 0.83 to 1.23 for the upper limit of agreement. In addition, only one point (2%), laid outside the 95% limits of agreement, confirming that accuracy of the test method was acceptable for the majority of subjects. The between-method difference, using the average values for each subjects, did not systematically vary over the range of measurements as demonstrated by a small and not statistically significant Kendall's rank correlation tau (τ = 0.17; p = 0.089), in spite of the tendency for an overestimation bias by bioimpedance at higher values of cardiac index ( and ).
Figure 3. Plots of difference in cardiac index (CI) estimation between the reference (thermodilution) and test method (thoracic electrical bioimpedance system) (y-axis) vs the average cardiac index value of test and reference method (x-axis). Data are shown for the 51 cardiac patients. Dashed lines refer to bias, and upper and lower limits of agreement.
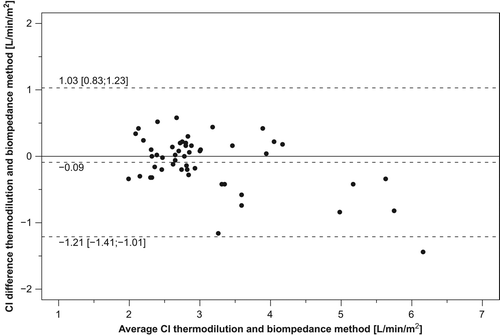
The repeatability coefficient for cardiac index determined by thermodilution was 0.78 l/min/m2, while it was 0.95 l/min/m2 for the thoracic electrical bioimpedance method, indicating only a slightly better internal agreement for the reference method. The intraclass correlation coefficients for fixed raters and the assessment for interreader agreement for random raters were similarly high for both the thermodilution [0.90 for both (95% confidence interval: 0.86, 0.94); p < 0.0001] and for the bioimpedance method [0.91 for both (0.87, 0.94); p < 0.0001], indicating high reliability.
Discussion
In the literature, a flurry of reports on validation of different bioimpedance devices vs thermodilution, the clinical standard for cardiac output determination (Citation3,Citation4,Citation22), is available. However, so far no consensus has been reached on the accuracy of impedance cardiography in the measurement of cardiac output since in some validation studies the method was evaluated as highly accurate, whereas in others more dispersion between the two methods was found (Citation12,Citation13).
In our paper, we report on a validation study of a new impedance device against thermodilution by presenting bias, limits of agreement, percentage error and repeatability coefficients, as currently universally recommended (Citation13,Citation18,Citation19,Citation23). The Bland–Altman method adjusted for repeated measures was used for analysis of the validation data because it measures the extent of deviations from the line of complete agreement (no bias = 0) between the methods (Citation18,Citation19). This is a much more reliable estimation of agreement between methods than the correlation coefficient, often used in the past, which measures how close to a straight line the pairs of measurements lie, which does not automatically imply that the line is the one of complete agreement.
We found a good agreement between the cardiac index measurements determined by non-invasive and invasive device: the bias between the two methods was −0.09 l/min/m2. The percentage error was 26%, namely within the ± 30% limit of agreement: this indicates that the test method is no less accurate than the reference method (Citation13), in spite of the tendency towards a small overestimation error by the bioimpedance device at higher values of cardiac index. The use of the percentage error strengthens the study results, since this measure incorporates not only the error of the method to be tested but also adjustments for errors in the reference method itself (Citation13).
In our study, we were also able to obtain repeated measurements in the same subject. This further increases the power of our results, but also allowed us to assess the degree of (short-term) repeatability of the methodology under test. The coefficients of repeatability were similar for the two methods (0.78 for thermodilution and 0.95 for bioimpedance) and the intraclass correlation coefficients were high (0.90 thermodilution and 0.91 biompedance), both indicating a performance of the non-invasive method comparable with that of thermodilution.
Although we acknowledge that we were able to assess only short-term repeatability of cardiac index by bioimpedance, nevertheless we must emphasize that limited evidence exists in literature for this important aspect. Previous evidence suggests that bioimpedance may have an extremely variable ability to adequately reflect cardiac output changes over time in different situations or during postural changes (Citation24–27). In addition, it has been shown that the reproducibility of cardiac index under standardized conditions is better in the short than in the long term. Verhoeve and coworkers (Citation28) showed a high degree of reproducibility of measurements obtained by means of bioimpedance cardiography during the same day (at few minutes interval) and after 24 h, in 96 cardiac patients enrolled in a rehabilitation clinic (Citation28). Intra-day correlation coefficient showed a 95% range of error of 0.92–0.96, while the percentage of variability in cardiac index from day 1 to day 2 was ± 6% with a correlation coefficient of 0.79 (Citation28). In another study, performed in 31 consecutive patients admitted in an intensive care unit, the coefficient of reproducibility for cardiac output obtained with bioimpedance was very close to that obtained through thermodilution (0.6 l/min vs 1.0 l/min), but there was no agreement between the absolute values of cardiac output (Citation29). Finally, Jewkes and coworkers (Citation30) evaluated the reproducibility of cardiac output estimation in resting supine volunteers by a thoracic electrical bioimpedance cardiograph over a short time period (30 min) and over several days. The reproducibility was better on the short term and worsened on the long term, with an average variation coefficient increasing from 5% to 11%. Unfortunately, in most of the previously referred studies, an inappropriate or not completely appropriate analytical approach was employed for assessing reproducibility.
Limitations of the study
When interpreting the data presented in our study, some methodological aspects and limitations must be considered. First, it may be argued that the observations made are based on a relatively small number of patients and thus may be of a limited value. However, the overall number of cardiac index determinations collected in our 51 subjects (n = 255) was sufficiently high to empower the study results. Second, we assessed reproducibility over a very short time interval and in standard supine position only. We cannot exclude, as suggested by the literature for most devices, that reproducibility of cardiac index estimation by impedance cardiography with the test device may worsen when assessed over a longer observation period or in different postures. Third, we did not test accuracy in situations or conditions known to negatively affect reliability of impedance measurement. We also acknowledge that further studies are needed to establish the validity of impedance cardiography by the Hotman device in unconventional situations.
Conclusions
At present, there is much controversy regarding the utility and safety of the pulmonary artery catheterization for the evaluation of cardiac output. Non-invasive techniques may represent an attractive alternative to increase the chance of assessing cardiac output also in subjects with contraindications to invasive procedures, but these techniques have not always met accuracy standards. In our validation study, we demonstrated that a totally non-invasive method of cardiac output monitoring by a new- generation thoracic bioimpedance device may perform well as a moderately invasive tool in high risk cardiac patients. Our results suggest that progress of hardware and software, including digital signal processing and new algorithms, may improve the quality of the results of non-invasive cardiac output estimation by new devices. However, since accuracy of measurements by impedance cardiography may be negatively affected by different conditions and postures, further studies are required to demonstrate the reliability of the Hotman device in such situations. Additional studies are also needed to investigate more in depth the tendency for an overestimation bias by the bioimpedance approach at higher values of cardiac index, observed in our study.
Competing interests
This study was supported by an unrestricted unconditional grant from the manufacturer of the Hotman bioimpedance device (Hemo Sapiens Inc. Sedona, Arizona, USA). The authors have no financial relationship with any product or manufacturer mentioned in the article. The authors declare that they have no competing interests.
References
- Vincent JL, Rhodes A, Perel A, Martin GS, Della Rocca G, Vallet B, et al. Clinical review: Update on hemodynamic monitoring – A consensus of 16. Crit Care. 2011;15:229.
- Mathews L, Singh RK. Swan–Ganz catheter in hemodynamic monitoring. J Anaesth Clin Pharmacol. 2006;22:335–345.
- Mathews L, Singh RK. Cardiac output monitoring. Ann Card Anaesth. 2008;11:56–68.
- Reuter DA, Huang C, Edrich T, Shernan SK, Eltzschig HK. Cardiac output monitoring using indicator–dilution techniques: Basics, limits, and perspectives. Anesth Analg. 2010; 110:799–811.
- Alhashemi JA, Cecconi M, Hofer CK. Cardiac output monitoring: An integrative perspective. Crit Care. 2011;15:214.
- Woltjer HH, Bogaard HJ, de Vries PM. The technique of impedance cardiography. Eur Heart J. 1997;18:1396–1403.
- Osypka MJ, Bernstein DP. Electrophysiologic principles and theory of stroke volume determination by thoracic electrical bioimpedance. AACN Clin Issues. 1999;10:385–399.
- Strobeck JE, Silver MA. Beyond the four quadrants: The critical and emerging role of impedance cardiography in heart failure. Congest Heart Fail. 2004;10 Suppl 2:1–6.
- Tang WH, Tong W. Measuring impedance in congestive heart failure: Current options and clinical applications. Am Heart J. 2009;157:402–411.
- Sodolski T, Kutarski A. Impedance cardiography: A valuable method of evaluating haemodynamic parameters. Cardiol J. 2007;14:115–126.
- Fuller HD. The validity of cardiac output measurement by thoracic impedance: A meta-analysis. Clin Invest Med. 1992; 15:103–112.
- Raaijmakers E, Faes TJ, Scholten RJ, Goovaerts HG, Heethaar RM. A meta-analysis of three decades of validating thoracic impedance cardiography. Crit Care Med. 1999;27: 1203–1213.
- Critchley LA, Critchley JA. A meta-analysis of studies using bias and precision statistics to compare cardiac output measurement techniques. J Clin Monit Comput. 1999;15:85–91.
- Wang DJ, Gottlieb SS. Impedance cardiography: More questions than answers. Curr Cardiol Rep. 2006;8:180–186.
- Badila E, Tîrziu C, Iorgulescu CN, Stefuriac M, Bartos D, Dorobantu M. The role of thoracic electrical bioimpedance in the control of high blood pressure. Revista româna de cardiologie. 2006;21:13–20.
- Mimoun L, Hering D, Boutouyrie P, Kucharska W, Laurent S, Narkiewicz K. Reproducibility of non-invasive cardiac index measurement with impedancemetry in hypertensives and healthy controls [abstract]. J Hypertens. 2009;27 Suppl 4:S370.
- Du Bois D, Du Bois EF. A formula to estimate the approximate surface area if height and weight be known. 1916. Nutrition. 1989;5:303–311.
- Altman DG, Bland JM. Measurement in medicine: The analysis of method comparison studies. Statistician. 1983;32: 307–317.
- Bland JM, Altman DG. Statistical methods for assessing agreement between two methods of clinical measurement. Lancet. 1986;1:307–310.
- British Standards Institution. Precision of test methods, part 1: Guide for the determination of repeatability and reproducibility for a standard test method. BS 5497, Part 1. London: BSI; 1979.
- Koch GG. Intraclass correlation coefficient. In Kotz S, Johnson NL, editors. Encyclopedia of statistical sciences. 4. New York: John Wiley & Sons; 1982. p 213–217.
- Stetz CW, Miller RG, Kelly GE, Raffin TA. Reliability of the thermodilution method in the determination of cardiac output in clinical practice. Am Rev Respir Dis. 1982;126: 1001–1004.
- Cecconi M, Rhodes A, Poloniecki J, Della Rocca G, Grounds RM. Bench-to-bedside review: The importance of the precision of the reference technique in method comparison studies – With specific reference to the measurement of cardiac output. Crit Care. 2009;13:201.
- Hartleb M, Rudzki K, Waluga M, Janusz M, Karpel E. Usefulness of thoracic electrical bioimpedance in detection of ejection fraction changes. J Physiol Pharmacol. 2000;51: 151–159.
- Heringlake M, Handke U, Hanke T, Eberhardt F, Schumacher J, Gehring H, Heinze H. Lack of agreement between thermodilution and electrical velocimetry cardiac output measurements. Intensive Care Med. 2007;33: 2168–2172.
- Tomsin K, Mesens T, Molenberghs G, Gyselaers W. Diurnal and position-induced variability of impedance cardiography measurements in healthy subjects. Clin Physiol Funct Imaging. 2011;31:145–150.
- Reisner AT, Xu D, Ryan KL, Convertino VA, Rickards CA, Mukkamala R. Monitoring non-invasive cardiac output and stroke volume during experimental human hypovolaemia and resuscitation. Br J Anaesth. 2011;106:23–30.
- Verhoeve PE, Cadwell CA, Tsadok S. Reproducibility of non invasive bioimpedance measurements of cardiac function [abstract]. J Card Fail. 1998;4(suppl.):S34
- Ng HW, Coleman N, Walley TJ, Mostafa SM, Breckenridge AM. Reproducibility and comparison of cardiac output measurement by transthoracic bioimpedance and thermodilution methods in critically ill patients. Clin Intensive Care. 1993;4: 217–221.
- Jewkes C, Sear JW, Verhoeff F, Sanders DJ, Foëx P. Non- invasive measurement of cardiac output by thoracic electrical bioimpedance: A study of reproducibility and comparison with thermodilution. Br J Anaesth. 1991;67:788–794.