Abstract
Introduction. Oxygen free radicals are involved in pathophysiology of ischemia/reperfusion (I/R) injury. This study was designed to assess the possible protective effect of pycnogenol (PYC) against I/R-induced oxidative renal damage. Materials and methods. Wistar albino rats were unilaterally nephrectomized and subjected to 45 min of renal pedicle occlusion followed by 3 h of reperfusion. PYC (10 mg kg−1, i.p.) or saline was administered at 15 min prior to ischemia and immediately before the reperfusion period. At the end of the 3 h, rats were decapitated and trunk blood was collected. Creatinine, blood urea nitrogen (BUN), and lactate dehydrogenase (LDH) activity were measured in the serum samples, while proinflammatory cytokines, TNF-α, IL-1β, and IL-6 levels were assayed in plasma samples. Kidney samples were taken for the determination of tissue malondialdehyde (MDA), glutathione (GSH) levels, Na+,K+-ATPase, and myeloperoxidase (MPO) activities, and the extent of tissue injury was analyzed microscopically. Results. Ischemia/reperfusion caused a significant decrease in tissue GSH level and Na+,K+-ATPase activity, which was accompanied with significant increases in the renal MDA level and MPO activity. Similarly, serum creatinine and BUN levels, as well as LDH and IL-1β, IL-6, and TNF-α levels, were elevated in the saline-treated I/R group as compared to saline-treated control group. On the other hand, PYC treatment reversed all these biochemical indices, as well as histopathological alterations that were induced by I/R. Conclusions. Findings of the present study suggest that pycnogenol exerts renoprotective effects, via its free radical scavenging and antioxidant activities, that appear to involve the inhibition of tissue neutrophil infiltration.
Introduction
Renal ischemia/reperfusion (I/R) injury is associated with increased mortality and morbidity rates due to acute renal failure (ARF). Various clinical–surgical procedures such as kidney transplantation, bypass processes, renal angioplasty, and clamping of the renal pedicle or the aorta above the renal arteries are frequent urological or vascular events and require temporary interruption or reduction of the renal perfusion flux.Citation[1,2] Although the return of blood flow to ischemic tissue can result in recovery of normal functions, the tissue may also be injured paradoxically during the process of reperfusion.Citation[3]
The mechanisms proposed to explain the I/R injury include reactive oxygen species (ROS) such as superoxide radical (O2−), hydrogen peroxide (H2O2), and hydroxyl radical (OH−) neutrophil accumulation, and the subsequent release of additional ROS.Citation[4,5] The infiltration of polymorphonuclear leukocytes in a tissue is characteristic of acute inflammation and indicates the collective action of chemotactic mediators.Citation[6] Once neutrophils migrate into the ischemic area, they release reactive oxygen species, proteases, elastase, myeloperoxidase (MPO), cytokines, and various other mediators,Citation[7] all of which are involved in tissue injury. The protection provided by free radical scavengers against ROS produced during injury further supports the hypothesis that free radical species are involved in the cellular pathogenesis of I/R injury.Citation[8,9] Because previous interventions against ARF have proved to be largely ineffective and dialysis remains the only effective therapy, the development of novel therapeutic interventions against I/R-induced renal injury is a topic of intense research interest. Biological compounds with anti-oxidant properties may contribute to the protection of cells and tissues against the deleterious effects of ROS and other free radicals induced by I/R.Citation[10,11] Thus, agents proposed to be useful in the clinical settings of I/R damage include free radical scavengers.
Naturally occurring flavonoids possess free radical scavenging properties and neuroprotection from oxidative injury by their ability to modulate intracellular signals.Citation[12] These flavonoids are found in fruits, vegetables, and plant-derived beverages, and may have important roles as dietary components via cytoprotective actions in many organs.Citation[13,14] Pycnogenol (PYC), a patented combination of bioflavonoids extracted from the bark of French maritime pine Pinus maritima,Citation[15] is prepared by a standardized procedure that includes the extraction of fresh pine bark with ethanol and water.Citation[16] This mixture is mainly composed of polyphenolic monomers, procyanidins, and phenolic or cinnamic acids and their glycosides procyanidins, flavonoids, and phenolic compounds.Citation[16–18] PYC has been also shown to exert ameliorative effects on cardiovascular, skin, cognitive, and menstrual disorders, as well as in the context of other diseases and disease processes such as diabetes, asthma, and inflammation.Citation[19] The mechanisms of these effects of PYC extract have been elucidated in a variety of in vitro and cell culture studies.Citation[20,21] In addition to its radical scavenging activity, an inhibition of NF-κB-dependent gene expression and decrease of the activity of various pro-inflammatory mediators and adhesion molecules were observed after incubation of cells with the pycnogenol extract.Citation[22]
In view of the findings described above, the aim of the present study was to evaluate to what extent PYC would provide protection against I/R-induced tissue damage in the kidney, by determining biochemical parameters and histological examination.
MATERIALS AND METHODS
Animals
Male Wistar albino rats (200–250 g) were housed in an air-conditioned room with 12-h light and dark cycles, where the temperature (22±2°C) and relative humidity (65–70%) were kept constant. All experimental protocols were approved by the Marmara University School of Medicine Animal Care and Use Committee.
Surgery and Experimental Protocol
Under anaesthesia (100 mg/kg ketamine and 0.75 mg/kg chlorpromazine, i.p.), a right nephrectomy was performed and the left renal pedicle was occluded for 45 min to induce ischemia prior to reperfusion for 3 h (I/R group). None of the animals died during the I/R period. Another group of rats underwent laparotomy only, where the kidneys were manipulated without nephrectomy or occlusion (sham-operated control group). Rats were treated with either PYC (10 mg/kg, i.p.; Mikrogen Pharmaceuticals, İstanbul, Turkey.) or saline on two occasions, 30 min prior to ischaemia and immediately before the reperfusion period. The dose of pycnogenol used in this study is based on previous studies where the antioxidant effects of pycnogenol are observed.Citation[23–25] Each group consisted of six rats.
Biochemical Analysis
The animals were decapitated at the end of the reperfusion period and trunk blood samples were collected to determine blood urea nitrogen (BUN)Citation[26] and creatinine,Citation[27] as indicators of kidney functions. Lactate dehydrogenase (LDH) activityCitation[28] and proinflammatory cytokines (TNF-α, IL-β, and IL-6) were also assayed in serum samples. In the renal tissue samples stored at –70°C, malondialdehyde (MDA) and glutathione levels and myeloperoxidase (MPO) and Na+,K+-ATPase activities were measured. Additional kidney samples were placed in 10% formaldehyde for histological evaluation of renal injury.
Plasma levels of TNF- α, IL-1β, and IL-6 were quantified according to the manufacturer's instructions and guidelines using enzyme-linked immunosorbent assay (ELISA) kits specific for the previously mentioned rat cytokines (Biosource International, Nivelles, Belgium). These particular assay kits were selected because of their high degree of sensitivity, specificity, inter- and intraassay precision, and small amount of plasma sample required to conduct the assay.
Tissue samples were homogenized with ice-cold 150 mM KCl for the determination of malondialdehyde (MDA) and glutathione (GSH) levels. The MDA levels were assayed for products of lipid peroxidation by monitoring thiobarbituric acid reactive substance formation as described previously.Citation[29] Lipid peroxidation was expressed in terms of MDA equivalents using an extinction coefficient of 1.56 × 105 M−1 cm −1, and results are expressed as nmol MDA/g tissue. GSH measurements were performed using a modification of the Ellman procedure.Citation[30] Briefly, after centrifugation at 3000 rev./min for 10 min, 0.5 ml of supernatant was added to 2 ml of 0.3 mol/L Na2HPO4.2H2O solution. A 0.2 ml solution of dithiobisnitrobenzoate (0.4 mg/mL 1% sodium citrate) was added, and the absorbance at 412 nm was measured immediately after mixing. GSH levels were calculated using an extinction coefficient of 1.36 × 104 M−1 cm −1. Results are expressed in μmol GSH/g tissue.
Myeloperoxidase (MPO) is an enzyme that is found predominantly in the azurophilic granules of polymorphonuclear leukocytes (PMN). Tissue MPO activity correlates significantly with the number of PMN determined histochemically in inflamed tissuesCitation[31] and therefore is frequently utilized to estimate tissue PMN accumulation. MPO activity was measured in tissues in a procedure similar to that documented by Hillegas et al.Citation[32] Tissue samples were homogenized in 50 mM potassium phosphate buffer (PB, pH 6.0) and centrifuged at 41,400 g (10 min); pellets were suspended in 50 mM PB containing 0.5 % hexadecyltrimethylammonium bromide (HETAB). After three freeze and thaw cycles, with sonication between cycles, the samples were centrifuged at 41,400 g for 10 min. Aliquots (0.3 ml) were added to 2.3 ml of reaction mixture containing 50 mM PB, o-dianisidine, and 20 mM H2O2 solution. One unit of enzyme activity was defined as the amount of MPO present that caused a change in absorbance measured at 460 nm for 3 min. MPO activity was expressed as U/g tissue.
Measurement of Na+,K+-ATPase activity is based on the measurement of inorganic phosphate that is formed from 3 mM disodium adenosine triphosphate added to the medium during the incubation period.Citation[33] The medium was incubated in a 37° C water bath for 5 min with a mixture of 100 mM NaCl, 5 mM KCl, 6 mM MgCl2, 0.1 mM EDTA, 30 mM Tris HCl (pH 7.4). Following the preincubation period, Na2ATP, at a final concentration of 3 mM, was added to each tube and incubated at 37°C for 30 min. After the incubation, the tubes were placed in an ice bath, and the reaction was stopped. Subsequently, the level of inorganic phosphate was determined in a spectrophotometer (Shimadzu, Japan) at excitation wavelength of 690 nm.Citation[34] The specific activity of the enzyme was expressed as μμol Pi mg−1 protein h−1. The protein concentration of the supernatant was measured by the Lowry method.Citation[35]
Histopathological Analysis
For the light microscopic investigations, kidney samples were fixed with 10% formaldehyde, dehydrated in graded alcohol series, cleared in toluene, and embedded in paraffin. Tissue sections (5 μm) were stained with hematoxylin and eosin (H&E) and examined under an Olympus BX51 photomicroscope (Tokyo, Japan). All tissue sections were examined microscopically for the characterization of histopathological changes by an experienced histologist who was unaware of the treatment conditions.
Statistical Analysis
Statistical analysis was carried out using GraphPad Prism 4.0 (GraphPad Software, San Diego; California, USA). Each group consisted of eight animals. All data were expressed as means ± SEM. Groups of data were compared with an analysis of variance (ANOVA) followed by Turkey's multiple comparison tests. Values of p < 0.05 were regarded as significant.
RESULTS
As shown in , blood urea nitrogen (BUN) and creatinine evels in the saline-treated I/R group were significantly higher than those in the control rats (p < 0.001). When PYC was administered before ischemia and the subsequent reperfusion period, these elevations in BUN and serum creatinine levels were significantly depressed (p < 0.01). Similarly, lactate dehydrogenase activity, as a marker of generalized tissue damage, showed a significant increase in the saline-treated I/R animals (p < 0.001), while PYC administration reversed this effect (p < 0.01). Compared with the sham groups, levels of the pro-inflammatory cytokines TNF-α, IL-1β, and IL-6 in the saline-treated I/R groups were significantly increased. On the other hand, these I/R-induced changes were also reversed following PYC treatment (p < 0.01–0.001; see ).
Table 1 Effects of pycnogenol (10 mg/kg) treatment on some biochemical parametersin the serum of experimental groups
In accordance with this, I/R caused a significant decrease in renal GSH level (1.04 ± 0.19 μmol/g; p < 0.001) when compared to saline-treated sham group (2.29 ± 0.18 μmol/g), while in the PYC-treated I/R group, renal GSH content was found to be preserved (1.95 ± 0.16 μmol/g; p < 0.05), not being significantly different from that of the saline-treated sham group (see ). The renal tissue MDA content in the saline-treated sham group (34.3 ± 5.6 nmol/g) was elevated by I/R injury (67.8 ± 7.5 nmol/g, p < 0.05); however, PYC treatment significantly decreased the I/R-induced elevation in renal MDA level (37.4 ± 7.2 nmol/g, p < 0.05; see ).
Figure 1. (a) Glutathione (GSH) and (b) malondialdehyde (MDA) levels in the kidney tissue of saline- or pycnogenol (PYC)-treated I/R groups and sham groups. Each group consists of six animals. * p < 0.05, *** p < 0.001, compared to saline-treated sham group. +p < 0.05, compared to saline-treated I/R group.
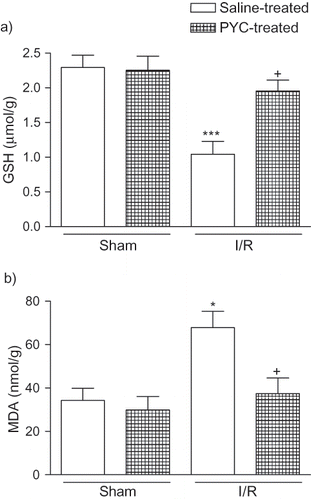
Myeloperoxidase activity, which is accepted as an indicator of neutrophil infiltration, was significantly higher in the kidney tissue of the saline-treated I/R group (28.3 ± 4.2 U/g, p < 0.001) than that of the saline-treated sham group (9.9 ± 1.5 U/g; see ). On the other hand, PYC treatment in I/R group significantly decreased renal tissue MPO level (14.9 ± 2.6, p < 0.01), which was found to be no different than that of the saline-treated sham group. The activity of Na+,K+-ATPase was shown to be significantly decreased in thre saline-treated I/R group (0.65 ± 0.17 μmol/mg protein/h, p < 0.01) compared with saline-treated sham group (2.55 ± 0.39 μmol/mg protein/h); however, PYC treatment significantly increased the I/R-induced reduction in renal Na+,K+-ATPase activity (1.93 ± 0.19 μmol/mg protein/h, p < 0.05; see ).
Figure 2. (a) Myeloperoxidase and (b) Na+-K+ ATPase activity in the kidney tissue of saline- or pycnogenol (PYC)-treated I/R groups and sham groups. Each group consists of six animals. ** p < 0.01, *** p < 0.001, compared to saline-treated sham group. +p < 0.05, ++p < 0.01, compared to saline-treated I/R group.
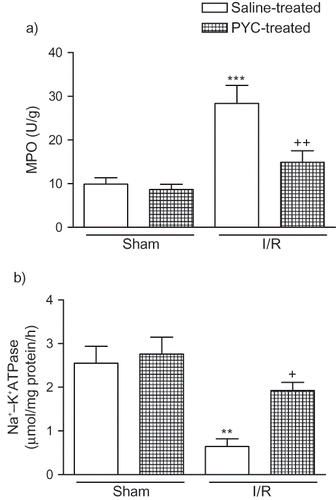
Light microscopic evaluation of the sham group, regular kidney morphology with Bowman's spaces, glomeruli, and tubular epithelium (see ) was observed. In the IR group (see ), severe vascular congestion, degeneration of the tubular epithelium, glomerular and Bowman's space structures, and severe inflammatory cell infiltration were observed. On the other hand, morphologically demonstrated degenerations in the kidney tissues of the rats were clearly improved when the animals were treated by PYC (see ).
Figure 3. (a) Regular morphology of kidney was observed in sham group. (b) Degenerated Bowman's space and glomerular structure (*), severe vascular congestion (arrow), and degenerated tubular epithelium (arrow head) and inflammatory cell infiltration (i) were observed in the IR groups. (c) Quite regular Bowman's space and glomerular structure, mild vascular congestion (v), and degenerated tubular epithelium (arrow) were observed in PYC-treated IR group. H&E staining, original magnifications: 200×, inset: 400×.
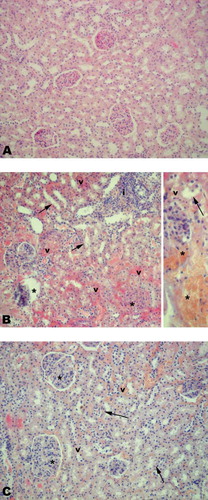
DISCUSSION
In the present study, our results demonstrated that temporary blockade of the renal blood supply yielded to structural and functional alterations in the kidney, with a concomitant increase in pro-inflammatory cytokines in the blood. PYC reduced the severity of injury and depressed the concentration of these cytokines. In addition, histopathological evaluation of the kidneys also confirms the protective effect of PYC.
In macrophages, nuclear factor κB (NF-κB) in cooperation with other transcription factors coordinates the expression of genes encoding both iNOS and TNF-α. NF-κB plays a critical role in the activation of immune cells by upregulating the expression of many cytokines essential to the immune response.Citation[36] In particular, NF-κB stimulates the production of IL-1, IL-6, TNF-α, lymphotoxin, and IFN-γ.Citation[37] It has been recently shown that tissue expression of TNF-α, IL-1β, and IL-6 was increased following renal I/R.Citation[38] In accordance with these findings, in the present study, the plasma levels of the pro-inflammatory cytokines TNF-α, IL-1β, and IL-6 were significantly elevated in I/R-induced renal injury, which was verified using both biochemical and histological assessment. Furthermore, PYC reversed all these parameters of injury and the levels of the inflammatory mediators while protecting the renal tissue against reperfusion-induced oxidative injury.
In the current study, the results obtained regarding the increased concentrations of MDA (an indicator of lipid peroxidation) and decreased GSH concentration in the kidney clearly indicate that I/R is able to induce the oxidative stress. Enhanced peroxidation of lipids in intra- and extracellular membranes results in the damage to the cells, tissues, and organs. Several studies have demonstrated that ischemia in the kidney is associated with lipid peroxidation, which is an autocatalytic mechanism leading to oxidative destruction of cellular membranes, and their destruction can lead to the production of toxic, reactive metabolites and cell death.Citation[39–41] On the other hand, cells are able to defend themselves from damaging effects of oxygen radicals by way of their own antioxidant mechanisms, including enzyme systems, vitamins, elements, and some antioxidant molecules.Citation[42] Reduced thiol agents, such as GSH, which are capable of interacting with free radicals to yield more stable elements, are known for their ability to repair membrane lipid peroxides.Citation[43] In this sense, GSH and other antioxidants play a critical role in limiting the propagation of free-radical reactions, which would otherwise result in extensive lipid peroxidation. In the present study, I/R of the kidney significantly depleted tissue GSH stores, indicating that GSH was used as an antioxidant for the detoxification of toxic oxygen metabolites, while the susceptibility of the involved tissues to I/R-induced oxidative injury was enhanced. On the other hand, tissue GSH content was not depleted in the PYC-treated I/R groups, suggesting that PYC possessed antioxidant effects and preserved the cellular antioxidant stores.
During ischemia, particularly the reperfusion phase, tissue injury involves the participation of several potential sources of toxic oxygen species, including mitochondrial electron transport systems, purine catabolism by xanthine oxidase, and infiltration of phagocytes (i.e., neutrophils and monocytes). As already mentioned above, one plausible mechanism for inducing tissue damage is free radical-induced lipid peroxidation. Furthermore, the importance of circulating polymorphonuclear leukocytes as mediators in I/R has also been widely investigated. ROS can generate hypochlorous acid (HOCl) in the presence of neutrophil-derived myeloperoxidase (MPO) and initiate the deactivation of antiproteases and activation of latent proteases, which can lead to tissue damage.Citation[44,45] Several methods have been used to define the role of neutrophils in reperfusion tissue injury. One of them is a neutrophil-specific enzyme, myeloperoxidase activity.Citation[46] On the other hand, oxidative stress could also be involved in inflammatory glomerular lesions caused by series of mediators, including cytokines and chemokines, that can lead to leukocyte activation, production of ROS, and increased glomerular damage.Citation[47] Peripheral monocytes infiltrating the kidney have traditionally been considered the primary source of renal TNF. On the other hand, oxidants released during the reperfusion of ischemic tissue stimulate transcription factors involved in TNF expression.Citation[48] It has been shown that exogenous TNF induces renal cell apoptosis, glomerular endothelial damage, fibrin deposition, cellular infiltration, and renal failure.Citation[49,50] In the present study, the elevated tissue MPO activity and the increased serum TNF-α level indicate the contribution of neutrophil infiltration and the involvement of pro-inflammatory cytokine TNF-α in I/R-induced renal injury. In this study, as expected, I/R caused a marked increase in MPO activity; furthermore, this increase was inhibited by PYC treatment. This might also result in reduced lipid peroxidation and thus less accumulation of MDA, as the activation of neutrophils might lead to the generation of more oxygen reactive metabolites.
Na+,K+-ATPase is a membrane-dependent enzyme that enables the transport of sodium and potassium across the membrane against a concentration gradient by hydrolysis of ATP and maintenance of intracellular electrolyte homeostasis.Citation[51] Because membrane-bound enzymes require phospholipids for the maintenance of their activity and are susceptible to structural changes due to lipid peroxidation,Citation[50,52] assessment of the Na+,K+-ATPase activity is also used as an index for oxidant-induced tissue injury and lipid peroxidation.Citation[50] In ischaemia, the depletion of energy stores and the initiation of lipid peroxidation due to increased free radicals following reperfusion causes inhibition of membrane Na+,K+-ATPase activity.Citation[53,54] Thus, I/R-induced ARF, characterized by impaired renal function, reduced glomerular filtration, and tubular sodium reabsorption, has been related to functional disorders of Na+/K+-ATPase.Citation[55] In the current study, tissue injury was accompanied by a simultaneous increase in MDA and a decrease in Na+,K+-ATPase activity, while the systemic inflammatory response was verified by increased serum LDH activity. On the other hand, treatment with PYC, by inhibiting neutrophil infiltration and subsequent lipid peroxidation and increasing the pump activity, significantly protected tissues against I/R injury.
Previous studies showed that PYC is a very potent antioxidant to scavenge reactive oxygen and nitrogen species, such as superoxide anion radical, hydroxyl radical, lipid peroxyl radical (LOO•), peroxynitrite radical, and singlet oxygen (1O2). It has also been shown to bind to proteins, thereby affecting both structural and functional characteristics of key enzymes and other proteins involved in metabolism; to participate in the cellular antioxidant network, especially in prolonging the lifetime of ascorbyl radical, possibly through a one-electron reduction of dehydroascorbic acid; and to protect endogenous vitamin E and glutathione.Citation[20,56] It has been claimed that PYC has diverse beneficial effects on a wide range of medical conditions, including inflammation, diabetes, asthma, hypertension, attention deficiency hyperactivity disorder, cancer, immune disease, and others.Citation[17]
The antioxidant activity of PYC has been demonstrated to protect against various degenerative conditions caused by free radicals, including diabetes-induced oxidative stress,Citation[56] ethanol-induced neuronal cell death,Citation[57] 2,4,6-trinitrobenzene sulfonic acid-induced inflammatory bowel disease,Citation[58] and ionizing radiation-induced intestinal damage.Citation[59] The results of the above studies and our investigation strongly suggest that the protective effect of PYC against I/R induced tissue damage observed in this study largely resulted from antioxidant activity, including stabilization in the intracellular antioxidant defense systems and reductions in the lipid peroxidation products and the production of reactive oxygen species.
In conclusion, this study demonstrates for the first time that PYC, a phenolic compound, reduces I/R-induced renal injury and preserves renal function. The protective effect of PYC can be attributed, at least in part, to its ability to balance oxidant-antioxidant status, to inhibit neutrophil infiltration, and to regulate the inflammatory mediators, suggesting a future role in the treatment of oxidative renal injury and subsequent organ failure due to ischemia/reperfusion.
ACKNOWLEDGMENTS
The authors are grateful to Mikrogen Pharmaceuticals for supplying pycnogenol. The authors report no conflicts of interest. The authors alone are responsible for the content and writing of the paper.
REFERENCES
- Gueler F, Gwinner W, Schwarz A, Haller H. Long-term effects of acute ischemia and reperfusion injury. Kidney Int. 2004;66:523–527.
- Cologna AJ, Lima LV, Tucci SJr, Cyclosporine action on kidneys of rats submitted to normothermic ischaemia and reperfusion. Acta Cir Bras. 2008;23:36–41.
- Koo DD, Welsh KI, West NE, Endothelial cell protection against ischemia/reperfusion injury by lecithinized superoxide dismutase. Kidney Int. 2001;60:786–796.
- Paller MS, Hoidal JR, Ferris TF. Oxygen free radicals in ischemic acute renal failure in the rat. J Clin Invest. 1984;74:1156–1164.
- Bonventre JV. Mechanisms of ischemic acute renal failure. Kidney Int. 1993;43:1160–1178.
- Ishikawa F, Miyazaki S. New biodefense strategies by neutrophils. Arch Immunol Ther Exp (Warsz). 2005;53:226–233.
- Kelly KJ, Williams WW, Colvin RB, Intercellular adhesion molecule-1-deficient mice are protected against ischemic renal injury. J Clin Invest. 1996;97:1056–1063.
- Nath KA, Norby SM. Reactive oxygen species and acute renal failure. Am J Med. 2000;109:665–678.
- Yanarates O, Guven A, Sizlan A, Ameliorative effects of proanthocyanidin on renal ischemia/reperfusion injury. Ren Fail. 2008;30:931–938.
- Amersi F, Nelson SK, Shen XD, Bucillamine, a thiol antioxidant, prevents transplantation-associated reperfusion injury. Proc Natl Acad Sci USA. 2002;99:8915–8920.
- Seo MY, Lee SM. Protective effect of low dose of ascorbic acid on hepatobiliary function in hepatic ischemia/reperfusion in rats. J Hepatol. 2002;36:72–77.
- Mercer LD, Kelly BL, Horne MK, Beart PM. Dietary polyphenols protect dopamine neurons from oxidative insults and apoptosis: Investigations in primary rat mesencephalic cultures. Biochem Pharmacol. 2005;69:339–345.
- Paganga G, Miller N, Rice-Evans CA. The polyphenolic content of fruit and vegetables and their antioxidant activities. What does a serving constitute?. Free Radic Res. 1999;30:153–162.
- Youdim KA, Joseph JA. A possible emerging role of phytochemicals in improving age-related neurological dysfunctions: A multiplicity of effects. Free Radic Biol Med. 2001;30:583–594.
- Ansari MA, Keller JN, Scheff SW. Protective effect of pycnogenol in human neuroblastoma SH-SY5Y cells following acrolein-induced cytotoxicity. Free Radic Biol Med. 2008;45:1510–1519.
- Huang WW, Yang JS, Lin CF, Ho WJ, Lee MR. Pycnogenol induces differentiation and apoptosis in human promyeloid leukemia HL-60 cells. Leuk Res. 2005;29:685–692.
- Rohdewald P. A review of the French maritime pine bark extract (pycnogenol), a herbal medication with a diverse clinical pharmacology. Int J Clin Pharmacol Ther. 2002;40:158–168.
- Matsumori A, Higuchi H, Shimada M. French maritime pine bark extract inhibits viral replication and prevents development of viral myocarditis. J Cardiac Fail. 2007;13:785–791.
- Feng WY, Tanaka R, Inagaki Y, Pycnogenol, a procyanidin-rich extract from French maritime pine, inhibits intracellular replication of HIV-1 as well as its binding to host cells. Jpn J Infect Dis. 2008;61:279–285.
- Packer L, Rimbach G, Virgili F. Antioxidant activity and biologic properties of a procyanidin-rich extract from pine (Pinus maritima) bark, pycnogenol. Free Radic Biol Med. 1999;27:704–724.
- Rohdewald PJ. Pycnogenol, French maritime pine bark extract. In Coates PM, Blackman MR, Cragg GM, Levine M, Moss J, White JD ( eds.). Encyclopedia of dietary supplements. New York: Marcel Dekker; 2005:545–553.
- Grimm T, Chovanová Z, Muchová J, Inhibition of NF-kappaB activation and MMP-9 secretion by plasma of human volunteers after ingestion of maritime pine bark extract (Pycnogenol). J Inflamm (Lond). 2006;3:1.
- Kamuren ZT, McPeek CG, Sanders RA, Watkins JB III.. Effects of low-carbohydrate diet and pycnogenol treatment on retinal antioxidant enzymes in normal and diabetic rats. J Ocul Pharmacol Ther. 2006;22:10–18.
- Ahn TH, Yang YS, Lee JC, Ameliorative effects of pycnogenol on carbon tetrachloride-induced hepatic oxidative damage in rats. Phytother Res. 2007;21:1015–1019.
- Yang YS, Ahn TH, Lee JC, Protective effects of pycnogenol on carbon tetrachloride-induced hepatotoxicity in Sprague-Dawley rats. Food Chem Toxicol. 2008;46:380–387.
- Talke H, Schubert GE. Enzymatic urea determination in the blood and serum in the Warburg optical test. Klin Wochenschr. 1965;43:174–175.
- Slot C. Plasma creatinine determination. A new and specific Jaffe reaction method. Scand J Clin Lab Invest. 1965;17:381–387.
- Martinek RG. A rapid ultraviolent spectrophotomeetric lactic dehydrogenase assay. Clin Chim Acta. 1972;40:91–99.
- Beuge JA, Aust SD. Microsomal lipid peroxidation. Methods Enzymol. 1978;52:302–311.
- Beutler E. Glutathione in red cell metabolism. In Beutler E ( ed.). A manual of biochemical methods. 2nd ed. New York: Grune & Stratton; 1975:112–114.
- Bradley PP, Priebat DA, Christersen RD, Rothstein G. Measurement of cutaneous inflammation. Estimation of neutrophil content with an enzyme marker. J Invest Dermatol. 1982;78:206–209.
- Hillegass LM, Griswold DE, Brickson B, Albrightson-Winslow C. Assessment of myeloperoxidase activity in whole rat kidney. J Pharmacol Methods. 1990;24:285–295.
- Reading HW, Isbir T. The role of cation activated ATPase in transmitter release from the art iris. Q J Exp Physiol. 1980;65:105–116.
- Fiske CH, SubbaRow Y. The colorimetric determination of phosphorus. J Biol Chem. 1925;66:375–400.
- Lowry OH, Rosenbrough NJ, Farr AL, Randall RJ. Protein measurements with the folin phenol reagent. J Biol Chem. 1951;193:265–275.
- Kopp EB, Ghosh S. Adv Immunol. 1995;58:1–27 NF-kappa B and rel proteins in innate immunity..
- Park YC, Rimbach G, Saliou C, Valacchi G, Packer L. Activity of monomeric, dimeric, and trimeric flavonoids on NO production, TNF-alpha secretion, and NF-kappaB-dependent gene expression in RAW 264.7 macrophages. FEBS Lett. 2000;465:93–97.
- Kher A, Meldrum KK, Hile KL, Aprotinin improves kidney function and decreases tubular cell apoptosis and proapoptotic signaling after renal ischemia-reperfusion. J Thorac Cardiovasc Surg. 2005;130:662–669.
- Eschwege P, Paradis C, Conti M, In situ detection of lipid peroxidation bproducts as markers of renal ischemia injuries in rat kidneys. J Urol. 1999;162:553–557.
- Sener G, Sehirli O, Velioğlu-Oğünç A, Montelukast protects against renal ischemia/reperfusion injury in rats. Pharmacol Res. 2006;54:65–71.
- Sehirli O, Sener E, Cetinel S, Yüksel M, Gedik N, Sener G. Alpha-lipoic acid protects against renal ischaemia-reperfusion injury in rats. Clin Exp Pharmacol Physiol. 2008;35:249–255.
- Schwacha MG. Macrophages and post-burn immune dysfunction. Burns. 2003;29:1–14.
- Sibilia V, Rindi G, Pagani F, Ghrelin protects against ethanol-induced gastric ulcers in rats: Studies on the mechanisms of action. Endocrinology. 2003;144:353–359.
- Kettle AJ, Winterbourn CC. Myeloperoxidase: a key regulator of neutrophil oxidant production. Redox Rep. 1997;3:3–15.
- Reiter RJ, Tan DX, Osuna C, Gitto E. Actions of melatonin in the reduction of oxidative stress. J Biomed Sci. 2000;7:444–458.
- Laight DW, Lad N, Woodward B, Waterfall JF. Assessment of myeloperoxidase activity in renal tissue after ischemia/reperfusion. Eur J Pharmacol. 1994;292:81–88.
- Rodrigo R, Rivera G. Renal damage mediated by oxidative stress: A hypothesis of protective effects of red wine. Free Radic Biol Med. 2002;33:409–422.
- Li C, Browder W, Kao RL. Early activation of transcription factor NF-kappaB during ischemia in perfused rat heart. Am J Physiol. 1999;276:H543–H552.
- Beratni T, Zoja C, Corna D, Perico N, Ghezzi P, Remuzzi G. Tumor necrosis factor induces glomerular damage in the rabbit. Am J Pathol. 1989;134:419–430.
- Liebertal W, Koh JS, Levine JS. Necrosis and apoptosis in acute renal failure. Semin Nephrol. 1998;18:505–518.
- Skou JC, Esmann M. The Na,K-ATPase. J Bioenerg Biomembr. 1992;24:249–261.
- Kolgazi M, Şener G, Çetinel Ş, Gedik N, Alican İ. Resveratrol reduces renal and lung injury due to sepsis in rats. J Surg Res. 2006;134:315–321.
- Cuzzocrea S, Reiter RJ. Pharmacological action of melatonin in shock, inflammation and ischemia/reperfusion injury. Eur J Pharmacol. 2001;426:1–10.
- Ilhan N, Halifeoglu I, Ozercan HI, Ilhan N. Tissue malondialdehyde and adenosine triphosphatase level after experimental liver ischaemia–reperfusion damage. Cell Biochem Funct. 2001;19:207–212.
- Kwon TH, Frokiaer J, Han JS, Knepper MA, Nielsen S. Decreased abundance of major Na+ transporters in kidneys of rats with ischemiainduced acute renal failure. Am J Physiol Renal Physiol. 2000;278:F925–F939.
- Maritim A, Dene BA, Sanders RA, Watkins JBIII.. Effects of pycnogenol treatment on oxidative stress in streptozotocin-induced diabetic rats. J Biochem Mol Toxicol. 2003;17:193–199.
- Siler-Marsiglio KI, Paiva M, Madorsky I, Serrano Y, Neeley A, Heaton MB. Protective mechanisms of pycnogenol in ethanol‐insulted cerebellar granule cells. J Neurobiol. 2004;61:267–276.
- Mochizuki M, Hasegawa N. Therapeutic efficacy of pycnogenol in experimental inflammatory bowel diseases. Phytother Res. 2004;18:1027–1028.
- de Moraes Ramos FM, Schönlau F, Novaes PD, Manzi FR, Bóscolo FN, de Almeida SM. Pycnogenol protects against ionizing radiation as shown in the intestinal mucosa of rats exposed to x-rays. Phytother Res. 2006;20:676–679.