Abstract
Neutral endopeptidase (NEP: EC 3.4.24.11) is involved in the degradation of peptides such as atrial natriuretic peptide, angiotensin II (AngII), and endothelin-1 (ET-1). In this study we propose that NEP inhibition provides protection in glycerol-induced acute renal failure (ARF). Renal vascular responses were evaluated in ARF rats where ARF was induced by injecting 50% glycerol in candoxatril, a NEP inhibitor (30 mg/kg, orally; for 3 weeks) pretreated rats. AngII and U46619 (a TxA2 mimetic) vasoconstriction was increased (2- to 4-fold) in ARF while ET-1 vasoconstriction was surprisingly reduced (23 ± 3%; p < 0.05). In ARF, candoxatril paradoxically enhanced ET-1 response (60 ± 20%; p < 0.05) but reduced AngII vasoconstriction (51 ± 11%; p < 0.05) without affecting U46619 response. However, candoxatril treatment was without effect on plasma ET-1 and TxB2 levels in ARF. Candoxatril reduced plasma AngII by 34 ± 4% (p < 0.05) in ARF which was ∼3.5-fold higher compared to control. Candoxatril doubled the nitrite excretion in control but was without effect on proteinuria or nitrite excretion in ARF. Candoxatril enhanced Na+ and creatinine excretion in ARF by 73 ± 9% and 33 ± 2%, respectively. These results suggest that NEP inhibition may confer protection in glycerol-induced ARF by stimulating renal function but without a consistent effect on renal production and renal vascular responses to endogenous vasoconstrictors.
INTRODUCTION
Neutral endopeptidase (NEP) is a membrane-bound zinc-dependent metallopeptidase with a widespread distribution in the vascular endothelium, smooth muscle cells, and the brush border of the renal tubular cells. The highest concentration of NEP has been localized in the vesicles of the renal proximal tubule confirming their critical role in renal tubular function.Citation1 NEP hydrolyzes and inactivates a number of endogenous vasoactive peptides, some of which could alter various functions of cells present in the arterial wall. Thus, NEP catalyzes the degradation of the vasodilator atrial and brain natriuretic peptide (ANP and BNP),Citation2,Citation3 adrenomedullin,Citation4 substance PCitation5, and the vasoconstrictors angiotensin II (AngII) and endothelin-1 (ET-1).Citation6 The exact mechanism(s) involved in the effects produced following inhibition of NEP has been the subject of much debate. NEP inhibitors are a novel and effective strategy initially targeted for the treatment of hypertension and heart failure.Citation7–9 Potentiation of natriuretic peptide activity by NEP inhibitors may reduce the influence of vasoconstrictors and proliferative mediators such as AngII and ET-1 and increase local levels of vasodilator ANP and bradykinin.Citation10
The consensus is that enhanced production of natriuretic peptides and the ensuing increase in nitric oxide (NO) and/or cGMP productionCitation11 account for the effects of NEP inhibitors in hypertension, chronic heart failure, and atherosclerosis.Citation12–14 However, data at variance with these observations were obtained from studies that demonstrated paradoxical increases in blood pressure that were accompanied by increased levels of ET-1Citation15 and AngIICitation6 following NEP inhibition. However, numerous studies are in agreement with the renoprotective role of NEP inhibitors especially in progressive nephropathyCitation10 and subtotal nephrectomy.Citation16 NEP inhibitors are therefore being evaluated for other indications, including renal diseases that are not completely responsive to angiotensin-converting enzyme (ACE) inhibitors.Citation17
Glycerol-induced acute renal failure (ARF) is characterized by acute tubular necrosis and massive vasoconstriction in renal vessels leading to renal ischemia.Citation18,Citation19 We reported earlier that there was increased renal vascular response to endogenous vasoconstrictors such as AngII and impaired ET-1 response in this model of ARF.Citation20 Similarly we have shown an increased plasma AngII and AT1 receptor expression that is associated with an increased oxidative stress and reduced NO production.Citation21 The precise pathology behind glycerol-induced ARF has been the focus for numerous studies. It has been proven that AngII plays an important role in the ensuing renal ischemia and increased vasoconstriction that was observed in this model of renal failure as AngII inhibition via receptor blockade reduced the enhanced renal vascular reactivity.Citation20 There was an attenuation of progression of renal injury by vasopeptidase inhibition involving both ACE and NEP inhibitionCitation16 and a restoration of the balance of vasoactive hormones in progressive nephropathyCitation10 and profound natriuretic responses by NEP inhibition in chronic renal failure patients.Citation22 Surprisingly, in most of these cases, there was no influence on proteinuriaCitation23 though renal function was profoundly improved. Based on these observations, we postulate that NEP inhibition may confer renoprotection in glycerol model of ARF. Thus, we tested the effect of NEP inhibition with candoxatril (UK79300) in glycerol-induced ARF and examined the consequent modulation of renal production and vascular responses to endogenous vasoconstrictors.
MATERIALS AND METHODS
This study was approved by the Institutional Animal Care and Use Committee (IACUC) of Texas Southern University following the National Institutes of Health (NIH) guidelines for laboratory animal care and use. All chemicals used in this study were of the highest grade commercially available. Glycerol and AngII were obtained from Sigma-Aldrich Chemical Company (St. Louis, MO, USA). U46619 and ET-1 were obtained from Cayman Chemicals (Ann Arbor, MI). Candoxatril (UK79300) was a kind gift of Pfizer UK Inc. (England).
Male Sprague–Dawley rats (250–300 g, Harlan Sprague Dawley, Houston, TX, USA) were used for this study. The animals were housed under standard conditions of light and dark cycle with free access to food and water. Twenty-four-hour urine was collected for evaluation of renal function before starting any treatment. Rats were randomly divided in two groups: control and ARF. Each group was further divided into vehicle and treatment. Treatment groups received candoxatril (Candox: 30 mg/kg; orally for 3 weeks) while the control group received equal volumes of vehicle. Renal failure was induced in the ARF groups 24-hr prior to the day of the experiment.
Induction of acute renal failure
ARF was induced using the standard method involving administration of 50% glycerol (v/v, 8 mL/kg, i.m.). Required amounts of glycerol were administered as a deep intramuscular injection equally distributed to both hind legs. Rats were deprived of food and water for 24 hr after glycerol administration. After injecting glycerol, rats were placed in metabolic cages and 24-hr urine was collected to evaluate renal function as an indicator of renal failure.
Rat isolated perfused kidney
The isolated perfused kidney was prepared as previously described.Citation20 Briefly, after anesthesia with pentobarbital sodium (50 mg/kg i.p.), the left kidney was exposed by midline ventral laparotomy. The left renal artery was cannulated through the abdominal aorta and the kidney was perfused by means of a peristaltic pump (MasterFlex cartridge pump, Model 7515-10, Cole Parmer Instrument Company, Vernon Hills, IL, USA) with warmed (37°C), oxygenated (95% O2 and 5% CO2) Krebs–Henseleit buffer composed of (in mM) 118 NaCl, 25 NaHCO3, 1.9 CaCl2, 1.19 MgSO4, 4.75 KCl, 1.19 KH2PO4, and 11.1 dextrose (pH 7.2). The flow of perfusate was adjusted in each kidney to obtain a perfusion pressure of 80–120 mmHg. The flow rate achieved was 8.5–13 mL/min. The inferior vena cava was cut to allow the flow of perfusate and the kidney was removed from the surrounding fat and placed in an organ chamber pre-warmed at 37°C. Perfusion pressure was monitored constantly by means of a pressure transducer attached to a signal manifold (Transbridge, model TBM-4, World Precision Instrument, Sarasota, FL, USA) that is connected to a computer equipped with data acquisition system (model DI 720 and DI-205, DataQ Instruments, Akron, OH, USA). Because flow was maintained at a constant rate, change in perfusion pressure was used as an index of renal vascular resistance, an increase in perfusion pressure indicating vasoconstriction. All responses were measured as the differences between the minimum pressure and the peak responses. Drugs were kept on ice during the experiments. Agonists were injected close to the renal artery at a maximum volume ≤30 μL. Renal vascular response to agonists AII (2.5, 5, 10, and 25 ng), U46619, TxA2 mimetic (10, 50, and 100 ng), or ET-1 (5, 10, and 25 ng) was determined randomly as boluses in the perfusate.
Measurement of biochemical parameters
Urinary excretion of protein and creatinine were measured using commercially available kits (Sigma-Aldrich, St. Louis, MO, U SA). Urinary excretion of Na+ was measured by flame photometer (Genway FP7, Jenway Ltd., Essex, UK). Plasma AngII, Urine TxB2, and plasma ET-1 were determined by EIA kit (Cayman Chemicals, Ann Arbor, MI, USA) following manufacturer protocol. Urinary NO was determined by measuring its stable metabolite nitrite. In this fluorometric kit (Cayman Chemicals, Ann Arbor, MI, USA) nitrate was converted to nitrite using nitrate reductase and the total nitrite was quantified.
Statistical analysis
Changes in perfusion pressure were expressed as percentage changes from basal values. Data were presented as means ± SEM and group comparisons were made using ANOVA followed by Bonferroni post hoc test. In all cases p < 0.05 was considered as significant.
RESULTS
Average weight of the animals used in this study was 276 ± 19 g which was changed to 304 ± 15 g at the end of the study period. Basal perfusion pressure was not different in the isolated perfused kidneys from control (116 ± 6 mmHg) or ARF rats (118 ± 12 mmHg). Basal perfusion pressure was also not different between kidneys from control or ARF rats treated with candoxatril: 126 ± 7 and 122 ± 9 mmHg, respectively.
Effects of candoxatril on renal function and proteinuria
ARF was evident in glycerol-treated rats by a ∼2.5-fold increase in proteinuria compared to the control rats (). Candoxatril treatment did not influence protein excretion in any of the treated groups. On the other hand, urinary nitrite excretion was doubled (0.5 ± 0 to 1.09 ± 0.22 μmol/mg protein/24 hr) in control rats treated with candoxatril (). Surprisingly, there was a 200% increase in nitrite excretion in glycerol-induced ARF, which remained elevated in ARF rats treated with candoxatril. illustrates functional impairment of the kidney confirmed by the measurement of Na+ and creatinine excretion in the urine for 24 hr after induction of renal failure. In ARF rats, Na+ and creatinine excretion was blunted by 61 ± 5% (p < 0.05) and 33 ± 4% (p < 0.05), respectively. Candoxatril enhanced both Na+ and creatinine excretion by 73 ± 9% (p < 0.05) and 33 ± 2% (p < 0.05). This was accompanied by a significant reduction of plasma creatinine in candoxatril-treated ARF rats (30 ± 3%; p < 0.05) which was increased in untreated ARF by 81 + 8% (p < 0.05).
Effects of candoxatril treatment of vascular responses to AngII, U46619, and ET-1
Compared to that of control rats, there was a 4-fold increase in AngII-induced renal vasoconstriction in rats that underwent glycerol-induced ARF (). In control rats treated with candoxatril (Con + Candox), there was also an ∼2-fold increase in AngII-induced vasoconstriction. However, in ARF rats pretreated with candoxatril (ARF + Candox), AngII-induced increase in renal perfusion pressure was reduced by 51 ± 11% (p < 0.05). Similarly, U46619, a TxA2 mimetic, dose dependently increased renal perfusion pressure, which was significantly higher (p < 0.05) in ARF rats compared to the control rats (). On the contrary, candoxatril treatment did not affect U46619 vasoconstriction either in control or in ARF rats. Unlike with AngII, ET-1-induced increase in renal perfusion pressure was 23 ± 3% (p < 0.05) less in ARF rats compared to the control rats (). Although candoxatril reduced ET-1 vasoconstriction in control rats, it elicited an opposite effect in ARF rats as ET-1 response was increased up to a level similar to that of control rats.
FIGURE 3. Vascular responses to (a) AngII (2.5, 5, 10, and 25 ng), (b) ET-1 (5, 10, and 25 ng), and (c) U46619 (10, 50, and 100 ng), a TxA2 mimetic, in isolated kidneys prepared from control (Control) or ARF rats with (ARF + Candox; Con + Candox) or without (ARF) candoxatril (30 mg/kg/day; orally, 3 weeks) treatment. Numbers in the parentheses are the number of animals in each group. Values are mean ± SEM. *p < 0.05 versus control; **p < 0.05 versus ARF.
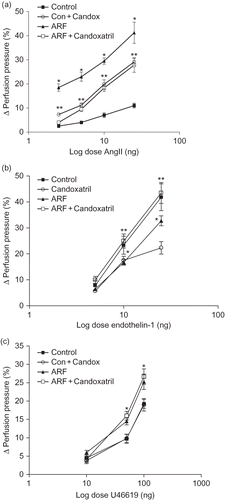
Effects of candoxatril treatment on the production of AngII, TxA2, and ET-1
Endogenous production of AngII, ET-1, and TxA2 () was significantly increased in ARF rats compared to the control rats. In the case of AngII and TxB2 (a stable metabolite of TxA2) this increase was ∼3.5-fold while in the case of ET-1 it was 23 ± 2% (p < 0.05). Candoxatril increased plasma levels of AngII in control rats while reducing it in ARF rats by 34 ± 4% (p < 0.05) (). However, candoxatril treatment did not influence plasma ET-1 or urinary excretion of TxB2 either in ARF or in control rats ( and ).
FIGURE 4. Plasma level of AngII (a), ET-1 (b), and urinary excretion of TxB2 (c) in control (Control) and ARF rats treated with (ARF + Candox; Con + Candox) or without (ARF) candoxatril (30 mg/kg/day; orally, 3 weeks) (n = 5 rats per group). Values are mean ± SEM. *p < 0.05 versus control; **p < 0.05 versus ARF.
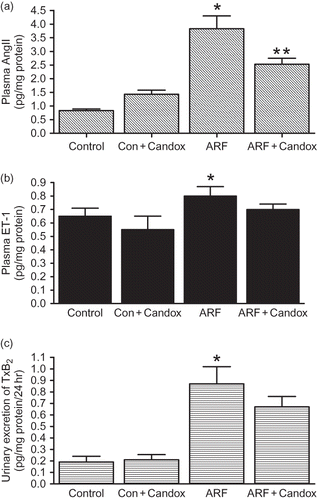
DISCUSSION
This study demonstrates the beneficial effect of chronic administration of candoxatril, a NEP inhibitor, on renal function and renal vascular reactivity in glycerol-induced ARF. In this study, the beneficial effect of candoxatril was also associated with a concomitant reduction in plasma AngII level. These findings extend the result of previous studies showing beneficial effect of NEP inhibition in ameliorating renal injury in progressive renal diseases.Citation16,Citation24 Similarly, the restoration of balance of vasoactive hormones by vasopeptidase inhibitors in progressive nephropathy implicates NEP inhibition in the correction of altered balance of vasoconstrictor/vasodilator mediators in kidney failure.Citation10
The most prominent beneficial effect of candoxatril observed in this study was the improvement of renal function as evident by increased natriuresis and enhanced creatinine clearance. This observation is supported by numerous experimental studies in which the renoprotective effects following NEP inhibition was attributed to its ability to produce profound natriuresis via increased ANP productions.Citation9,Citation22,Citation23,Citation25 This observation was further corroborated by the observation that NEP inhibition also inhibited the activation of aldosterone-mediated signaling.Citation9,Citation26 It is well established that aldosterone increases tubular sodium reabsorption in the inner medullary collecting duct cell which is also the site of action of NEP. Therefore, inhibition of aldosterone will complement the influence of NEP inhibition on natriuresis. Similar to these studies we also observed a profound natriuresis with candoxatril treatment suggesting that candoxatril may confer benefit in situations where renal function is compromised.
Candoxatril has also been reported to increase NO production,Citation25 which may also promote increasing renal perfusion and therefore renal function. This notion is supported by the observation of a 2-fold increase in nitrite excretion in control rats after treatment with candoxatril. On the contrary, in ARF rats, where nitrite excretion was already increased by ∼3-fold, candoxatril did not alter this increase any further. We propose that this increase in NO production in ARF is a protective response to the ensuing renal failureCitation20 where a maximally stimulated NO/NOS system had little or no room for candoxatril to exert any additional effect on NO production. The beneficial effect of elevated NO in ARF may also account for the reduced ET-1 vasoconstriction observed in ARF in this study. In a similar study, we have shown that increasing NO availability in ARF by antioxidants increased Na+ excretion and enhanced renal functionCitation21 confirming the critical role of NO in this model of ARF. Besides natriuresis, candoxatril also produces an increased creatinine clearance in ARF rats thus complementing enhancement of renal function. On the other hand, judged critically by proteinuria, candoxatril failed to protect the failing kidney in as much as proteinuria was unchanged. This is not surprising, as other studies have observed renoprotective effect of candoxatril despite the lack of improvement in proteinuria.Citation23 This may be explained by the fact that NEPs are predominantly expressed in the proximal nephron where they regulate the tubular function and may likely affect proximal tubular function. On the other hand, physical glomerular damage is the mechanical process that leads to leakage of myoglobin in the urine in ARFCitation18,Citation19 where inhibition of endopeptidase is of no effect. Absence of effects of candoxatril on NO excretion in glycerol-induced ARF may be due to involvement of other mechanism unknown at the moment.
The reduction in ET-1 vasoconstriction in this study corroborates our earlier observationCitation20 and may be caused by at least two reasons. First, increased renal production of NO in ARF which is a known inhibitor of ET-1 activity.Citation27 Second, the kinetics of ET-1 production in ARF is such that peak production was reached at 10 hr after ARF induction returning to normal or below normal by 24 hr,Citation28 a time coinciding with the time of experiments in this study. The long exposure of the kidney to endogenous ET-1 may therefore have caused receptor desensitization. In the presence of candoxatril, increased ET-1 vasoconstriction was a surprising observation in ARF. We interpret these data to reflect lack of ET-1 receptor desensitization due to reduced endogenous ET-1 production by candoxatril based on its documented ability to increased production of ANPCitation14,Citation29 or NO.Citation11 The changing dynamics between the effects of ARF on one hand and that of candoxatril on the other probably accounts for the unchanged plasma levels of ET-1 in candoxatril-treated ARF rats. Thus, there is a crucial balance between candoxatril-induced NO-mediated inhibition of ET-1 production and ET-1 degradation by candoxatril, which is different in normal or diseased conditions. This complex effect of candoxatril is in agreement with observations that candoxatril can reduce ET-1 production by inhibiting endothelin-converting enzyme (ECE) activityCitation14 on one hand but increased ET-1 level by inhibiting ET-1 degradationCitation30 on the other hand.
Endogenous vasoconstrictors such as AngII are known to contribute to the pathogenesis of glycerol-induced ARF or rhabdomyolysis. Besides tubular necrosis and vasoconstriction,Citation18,Citation31 increased responses to these vasoconstrictors have also been reported.Citation20 Effect of candoxatril on AngII metabolism is always the focus of study where AngII plays a major role as a vasoconstrictor. More importantly, there is no direct evidence that NEP inhibition can modulate AngII production by regulating ACE, and this is the reason for administration of ACE inhibitors together with NEP inhibitors in hypertensive diseases. The elevated plasma AngII level in candoxatril-treated control rats and an exaggerated vasoconstriction are consistent with candoxatril prevention of degradation of AngII by inhibiting NEP.Citation32 However, in the candoxatril-treated ARF rats, there was reduced AngII response and production. This may be caused by the fact that candoxatril enhanced the production and availability of natriuretic peptides in the kidney.Citation23 Since ANP and AngII work in the same sites of the kidneyCitation33 and ANP can block the stimulatory effects of AngII,Citation34 we speculate that the reduced plasma AngII level observed in this study may be attributed to the natriuretic peptides which modulate AngII production via inhibiting renin–angiotensin–aldosterone system.Citation9,Citation35 An additional axis for candoxatril-mediated reduction in AngII is NO since increased renal NO production by candoxatril may also inhibit the renin–angiotensin system.Citation36 There was no reported study showing the role of candoxatril on TxA2 production or metabolism. Therefore, inclusion of U46619, a TxA2 mimetic in the vascular response study and measurement of TxB2 in the urine, was a negative control to confirm that the effects of candoxatril on AngII and ET-1 are specific rather than a global influence of improvement in renal function or vascular reactivity.
In conclusion, we propose that candoxatril modulates the vascular responses to endogenous vasoconstrictors and enhances renal function. Absence of an unmasked effect of candoxatril on NO excretion in glycerol-induced ARF suggests involvement of other mechanisms, which are unknown at the moment. Therefore, we propose that enhancement of renal function may be related to NEP-mediated enhancement of NO production without protecting the kidney from the physical glomerular damage induced by myoglobin.
Acknowledgments
This study was supported by National Institutes of Health Grant HL03674.
REFERENCES
- Shima M, Seino Y, Torikai S, Imai M. Intrarenal localization of degradation of atrial natriuretic peptide in isolated glomeruli and cortical nephron segments. Life Sci. 1988;43:357–363.
- Stephenson SL, Kenny AJ. The hydrolysis of α-human atrial natriuretic peptide by pig kidney microvillar membranes is initiated by endopeptidase-24.11. Biochem J. 1987;243:183–187.
- Lang CC, Motwani JG, Coutie WJR, Struthers AD. Clearance of brain natriuretic peptide in patients with chronic heart failure: Indirect evidence for a neutral endopeptidase mechanism but against an atrial natriuretic peptide clearance receptor. Clin Sci. 1992;82:619–623.
- Lisy O, Jougasaki M, Schirger JA, Chen HH, Barclay PT, Burnett JCJr.. Neutral endopeptidase inhibition potentiates natriuretic actions of adrenomedullin. Am J Physiol. 1998;275:F410–F414.
- Skidgel RA, Engelbrecht S, Johnson AR, Erdos EG. Hydrolysis of substance P and neurotensin by converting enzyme and neutral endopeptidase. Peptides. 1984;5:769–776.
- Richards AM, Wittert G, Crozier IG, Chronic inhibition of endopeptidase 24.11 in essential hypertension: Evidence for enhanced atrial natriuretic peptide and angiotensin II. J Hypertens. 1993;11:407–416.
- Weber MA. Vasopeptidase inhibitors. Lancet. 2001;358:1525–1532.
- Campbell DJ. Vasopeptidase inhibition. A double-edged sword? Hypertension. 2003;41:383–389.
- Fl Martin, Stevens TL, Cataliotti A, Natriuretic and anti aldosterone actions of chronic oral NEP inhibition during progressive congestive heart failure. Kidney Int. 2005;67:1723–1730.
- Benigni A, Zoja C, Zatelli C, Vasopeptidase inhibitor restores the balance of vasoactive hormones in progressive nephropathy. Kidney Int. 2004;66:1959–1965.
- Marumo T, Nakaki T, Hishikawa K, Natriuretic peptide-augmented induction of nitric oxide synthase through cyclic guanosine 3’,5’-monophosphate elevation in vascular smooth muscle cells. Endocrinology. 1995;136:2135–2142.
- Burnett JCJr.. Vasopeptidase inhibition: A new concept in blood pressure management. J Hypertens. 1999;17:S37–S43.
- Burrell LM, Farina NK, Balding LC, Johnston CJ. Beneficial renal and cardiac effects of vasopeptidase inhibition with S21402 in heart failure. Hypertension. 2000;36:1105–1111.
- Grantham JA, Schirger JA, Wennberg PW, Modulation of functionally active endothelin-converting enzyme by chronic neutral endopeptidase inhibition in experimental atherosclerosis. Circulation. 2000;101:1976–1981.
- Ando S, Rahman MA, Butler JC, Senn BL, Floras JC. Comparison of candoxatril and atrilal natriuretic factor in healthy men: Effects on hemodynamics, sympathetic activity, heart rate variability, and endothelin. Hypertension. 1995;26:1160–1166.
- Cao Z, Burrell LM, Tikkanen I, Bonnet F, Cooper ME, Gilbert RE. Vasopeptidase inhibition attenuates the progression of renal injury in subtotal nephrectomized rats. Kidney Int. 2001;60:715–721.
- Ruggenenti P, Schieppati A, Remuzzi G. Progression, remission, regression of chronic renal diseases. Lancet. 2001;357:1601–1608.
- Karam H, Bruneval P, Clozel JP, Loffler BM, Bariety J, Clozel M. Role of endothelin in acute renal failure due to rhabdomyolysis in rats. J Pharmacol Exp Ther. 1995;274:481–486.
- Mandal AK, Davis JBJr, Bell RD, Miller JM. Myoglobinuria exacerbates ischemic renal damage in the dog. Nephron. 1989;53:261–267.
- Newaz MA, Oyekan AO. Vascular responses to endothelin-1, angiotensin II and U46619 in glycerol-induced acute renal failure. J Cardiovasc Pharmacol. 2001;38:569–577.
- Yousefipour Z, Ao Oyekan, Newaz MA. Antioxidant U74389G improves glycerol-induced acute renal failure without affecting PPARgamma gene. Ren Fail. 2007;29:903–910.
- Lipkin GW, Dawnay AB, Harwood SM, Cattell WR, Raine AE. Enhanced natriuretic response to neutral endopeptidase inhibition in patients with moderate chronic renal failure. Kidney Int. 1997;52:792–801.
- Jandeleit-Dahm K, Burrell LM, Kanazawa M, Casley D, Jackson B, Johnson CI. Effects of neutral endopeptidase inhibition I the rat remnant kidney model. Kidney Blood Press Res. 1998;21:419–424.
- Cohen DS, Mathis JE, Dotson RA, Graybill SR, Wosu NJ. Protective effects of CGS 30440, a combined angiotensin-converting enzyme inhibitor and neutral endopeptidase inhibitor, in a model of chronic renal failure. J Cardiovasc Pharmacol. 1998;32:87–95.
- Newaz MA, Yousefipour Z, Hercule H, Truong L, Oyekan AO. Chronic endopeptidase inhibition in DOCA-salt hypertension: Mechanism of cardiovascular protection. Clin Exp Hypertens. 2003;25:335–347.
- Rademaker MT, Fitzpatrick MA, Charles CJ, Comparison of chronic neutral endopeptidase inhibition and furosemide in an ovine model of heart failure. J Cardiovasc Pharmacol. 1996;27:439–446.
- Mitsutomi N, Akashi C, Odagiri J, Matsumura Y. Effects of endogenous and exogenous nitric oxide on endothelin-1 production in cultured vascular endothelial cells. Eur J Pharmacol. 1999;364:65–73.
- Shimizu T, Kuroda T, Ikeda M, Hata S, Fujimoto M. Potential contribution of endothelin to renal abnormalities in glycerol-induced acute renal failure in rats. J Pharmacol Exp Ther. 1998;286:977–983.
- Hu RM, Levin ER, Pedram A, Frank HJ. Atrial natriuretic peptide inhibits the production ad secretion of endothelin from cultured endothelial cells: Mediation through the C receptor. J Biol Chem. 1992;267:17384–17389.
- Nambi P, Pullen M, Wu HL, Prabhakar U, Hersh L, Gellai M. Down regulation of kidney neutral endopeptidase mRNA, protein and activity during acute renal failure: Possible mechanism for ischemia-induced acute renal failure in rats? Mol Cell Biochem. 1999;197:53–59.
- Yanagisawa H, Nodera M, Umemori Y, Role of angiotensin II, endothelin-1, and nitric oxide in HgCl2-induced acute renal failure. Toxicol Appl Pharmacol. 1998;152:315–326.
- Richards AM, Wittert G, Espiner EA, Yandle TG, Ikram H, Frampton C. Effects of inhibition of endopeptidase 24.11 on response to angiotensin II inhuman volunteers. Circ Res. 1992;71:1501–1507.
- Charloux A, Piquard F, Doutreleau S, Brandenberger G, Geny B. Mechanism of renal hyporesponsiveness to ANP in heart failure. Eur J Clin Invest. 2003;33:769–778.
- Oliveira-Souza M, Malnic G, Mello-Aires M. Atrial natriuretic peptide impairs the stimulatory effect of angiotensinII on H+-ATPase. Kidney Int. 2002;62:1693–1699.
- Ito T, Yoshimura M, Nakamura S, Inhibitory effect of natriuretic peptide on aldosterone synthase gene expresión in cultured neonatal rat cardiocytes. Circulation. 2003;107:807–810.
- Iwanami J, Mogi M, Iwai M, Horiuchi M. Inhibition of the rennin–angiotensin system and target organ protection. Hypertens Res. 2009;32:229–237.