Abstract
Studies from our laboratories indicate that Syzygium cordatum leaf extract contains triterpene mixtures [oleanolic acid (OA) and ursolic acid (UA)] with hypoglycemic properties. The aims of this study were to investigate the hypoglycemic effects of Syzygium aromaticum-derived OA and whether OA influenced the blood glucose lowering effects of insulin in streptozotocin (STZ)-induced diabetic rats. We envisaged that OA may provide a strategy with different mechanism of action for effective diabetic therapy because no single-marketed antidiabetic drug is capable of achieving long-lasting blood glucose control. The effects of various doses of OA and/or standard antidiabetic drugs on blood glucose were monitored in nondiabetic and STZ-induced diabetic rats given a glucose load after an 18-h fast. Rats treated with deionized water and standard antidiabetic drugs acted as untreated and treated positive controls, respectively. Blood glucose concentrations were measured at 15-min intervals for the first hour and hourly thereafter for 3 h. Blood glucose concentrations were also monitored in animals treated with OA and/or standard antidiabetic drugs for 5 weeks. OA like insulin decreased blood glucose concentrations in nondiabetic and STZ-induced diabetic rats. Combined OA and insulin treatment had even greater antihyperglycemic response, suggestive of a synergistic effect of the two. After 5 weeks, STZ-induced diabetic rats exhibited hyperglycemia and depleted hepatic and muscle glycogen concentrations. OA treatment lowered the blood glucose with concomitant restoration of glycogen concentrations to near normalcy. Our results suggest that OA may have a role in improving insulin sensitivity. These findings merit further research in this field.
INTRODUCTION
Diabetes mellitus, a syndrome affecting many people in all countries over the world, is commonly treated with blood glucose lowering agents. However, identification of new antidiabetic drugs that intervene in the early development of diabetes or to combat insulin resistance retains the attention of the scientific community. Studies in our laboratories have indeed reported that crude extracts of Opuntia megacantha (Miller) (Cactaceae), Allium sativum (Linnaeus) (Alliaceae), and Syzygium cordatum (Hochst.) (Myrtaceae) possess hypoglycemic properties.Citation1,Citation2 Herbal medicines have been used for many years by different cultures around the world, for both the prevention and management of diabetes.Citation3,Citation4 Bioactive compounds with hypoglycemic properties isolated from plant extracts include polysaccharides, flavonoids, xanthones, peptides, and triterpenes.Citation5,Citation6 Oleanolic acid (OA) (3β-hydroxy-olea-12-en-28-oic acid) and its isomer ursolic acid (UA) share many pharmacological properties such as hepatoprotective, anti-inflammatory, analgesia, cardiotonic, sedative, and tonic effects with plants containing triterpene constituents.Citation7 Considering the challenges and limitations that no single-marketed antidiabetic drug is capable of achieving long-lasting blood glucose control in the majority of patients or compensating for metabolic derangements, OA may provide a strategy with different mechanism of action and potential for effective therapy.
We have reported that the antihyperglycemic effects of the Syzygium cordatum (Hochst.) (Myrtaceae) crude leaf extract rich in triterpene mixtures (OA) and UA in streptozotocin (STZ)-induced diabetic rats are mediated in part through increased hepatic glycogen synthesis.Citation8 We, therefore, speculated that Syzygium aromaticum [(Linnaeus) Merrill & Perry] [Myrtaceae] (known as clove) extracts contain similar compounds. Accordingly, this study was designed to isolate OA from S. aromaticum flower bud and investigate its antihyperglycemic effects in STZ-induced diabetic rats. Additionally, we investigated possible synergistic antihyperglycemic effects between OA and insulin because some literature evidence suggests that OA is an insulin secretagogueCitation9–12 and exists in most food productsCitation13,Citation14 and African plant species used in traditional medicine.Citation7,Citation15 The STZ-induced diabetic rat model used in the study has been used extensively in our laboratories.Citation1,Citation2,Citation8
MATERIALS AND METHODS
Drugs and chemicals
Drugs were sourced from standard pharmaceutical suppliers. All other chemicals used were purchased from standard commercial suppliers and were of analytical grade quality.
Isolation of OA
OA was isolated form Syzygium aromaticum [(Linnaeus) Merrill & Perry] [Myrtaceae] (cloves) flower buds using a standard protocol that has been validated in our laboratory.Citation16 Briefly, air-dried powdered flower buds of S. aromaticum (1.74 kg) were sequentially extracted thrice at 24-h intervals with 3 L of hexane, dichloromethane, ethyl acetate, and methanol on each occasion. Recrystallization of ethyl acetate solubles (EAS) (25.5 g), which contained mixtures of OA/UA and methyl maslinate/methyl corosolateCitation8,Citation17 with ethanol, yielded pure OA whose structure was confirmed by spectroscopic analysis using 1D and 2D, Citation1H and Citation13C nuclear magnetic resonance (NMR) techniques. Preliminary studies indicated that the hypoglycemic effects of S. aromaticum-isolated OA and commercial OA were similar and hence the plant-extracted OA was used in the experiments as it is less costly.
Animals
Male Sprague–Dawley rats (250–300 g) bred and maintained at the Biomedical Research Unit, University of KwaZulu-Natal, South Africa were used. The animals had free access to standard rat chow (Epol-diet 4700, Epol, South Africa) and water, with a 12-h light/12-h dark cycle. Procedures involving animals and their care were conducted in conformity with institutional guidelines of the University of KwaZulu-Natal.
Induction of experimental diabetes mellitus
Diabetes mellitus was induced in the male Sprague–Dawley rats with a single intraperitoneal injection of STZ (60 mg kg−1) dissolved in freshly prepared 0.1 M citrate buffer (pH 6.3). Control animals were injected with the vehicle. Animals that exhibited glucosuria after 24 h, tested by urine strips (Rapidmed Diagnostics, Sandton, South Africa), were considered diabetic. Blood glucose concentration of 20 mmol l−1 or above measured after 1 week was considered as a stable diabetic state before experimental procedures commenced.
Experimental design
Nondiabetic and STZ-induced diabetic rats were divided into separate groups to study the acute and short-term (5 weeks) effects of OA on blood glucose (n = 6 in each group).
Effects of OA on blood glucose
The effects of OA on blood glucose were evaluated in nondiabetic and STZ-induced diabetic rats according to the method described previously.Citation18 Briefly, an 18 h fast was allowed for all animals, followed by measuring blood glucose (time 0). Subsequently, the animals were administered glucose (0.86 g kg−1, body weight, p. o.), followed by OA at various doses (40, 80, and 120 mg kg−1, p. o.). OA was freshly dissolved in dimethyl sulfoxide (DMSO, 2 mL) and normal saline (19 mL) before use in each case.Citation8 Rats treated with deionized water (3 mL kg−1, p.o.) and standard antidiabetic drugs insulin [200 μg kg−1, s.c., metformin (1,1-dimethylbiguanide hydrochloride, 500 mg kg−1, p.o.) and glibenclamide (glyburide; N-p-[2-(5-chloro-2-methoxybenzamido) ethylbenzene-sulphonyl-N′-cyclohexylurea), 500 μg kg−1, p.o.] served as control animals and positive control animals, respectively.
The influence of standard drugs on OA-induced hypoglycemic effects was studied in separate groups of animals that were treated with OA at either 40 or 80 mg kg−1 followed by insulin (100 or 200 μg kg−1, s.c.), glibenclamide (250 or 500 μg kg−1, p. o.), and metformin (250 or 500 mg kg−1, p.o.). Blood samples were collected from the tail veins of the animals at 15-min intervals for the first hour, and hourly thereafter for the subsequent 3 h for glucose measurements, using Bayer's Glucometer Elite® [Elite (Pvt.) Ltd., Health Care Division, Isando, South Africa].
Effects of OA on insulin secretion
Plasma insulin concentrations were measured in separate parallel groups of nondiabetic and STZ-induced diabetic rats as prepared to examine the blood glucose lowering effects of OA. Blood was collected by cardiac puncture into pre-cooled heparinized tubes for insulin determination after 60 min following OA (80 mg kg−1, p.o.), metformin (500 mg kg−1, p.o.), and insulin (200 μg kg−1, s.c.). Plasma insulin concentrations were also monitored in groups of animals treated with combined OA and insulin. In preliminary experiments, the plasma lowering effect of the treatments was found to reach plateau within 30 min and maintained for 60 min in fasted rats after OA or insulin administration. Separated plasma was stored at –70°C in a BioUltra freezer (Snijers Scientific, Holland) until insulin assay.
Short-term studies
Effects of OA on food and water intake and body weight changes
Groups of control and treated nondiabetic and STZ-induced diabetic male Sprague–Dawley rats were housed individually in Makrolon polycarbonate metabolic cages (Techniplats, Labotec, South Africa) for a 5-week period at the Biomedical Resource Unit, University of KwaZulu-Natal (n = 6 in each group). In those animals in which the effects of OA were investigated, the rats were treated with OA (80 mg kg−1) twice daily at 09:00 and 15:00 by means of a bulbed steel tube. Separate groups of rats similarly treated with deionized water (3 mL kg−1, p.o.) and standard antidiabetic drugs (metformin, 500 mg kg−1, p.o.; insulin, 200 μg kg−1, s.c) acted as untreated and treated positive controls, respectively. The weights of the animals were assessed every third day at 09:00.
Terminal studies
Blood samples were collected by cardiac puncture 24 h after the last treatment from all nonfasted groups of animals for glucose and insulin assay at the end of the 5-week treatment period. Samples of known liver and gastrocnemius muscle weights (1–1.5 g) were quickly removed from untreated and treated rats for glycogen measurement. The plasma, liver, and muscle samples were stored in a BioUltra freezer (Snijers Scientific) at –70°C until assayed.
Laboratory analyses
Plasma insulin concentrations were evaluated by ultrasensitive rat insulin ELISA kit (DRG Instruments GmBH, Marburg, Germany) with 100% cross-reactivity with insulin lispro (Humalog® Eli Lilly). The immunoassay is a quantitative method for the determination of plasma insulin utilizing two monoclonal antibodies which, together, are specific for insulin. The lower limit of detection was 1.74 pmol L−1. The intra- and inter-assay analytical coefficients of variation ranged from 4.4 to 5.5% and from 4.7 to 8.9%, respectively.
Glycogen was determined as described by Ong and Khoo.Citation19 The liver and gastrocnemius muscle tissue samples were homogenized in 2 mL of 30% potassium hydroxide (300 g L−1) and boiled at 100°C for 30 min, and then cooled in ice-saturated sodium sulfate. Glycogen was precipitated with ethanol, pelleted, and resolubilized in deionized water. Glycogen content was determined by the treatment with anthrone reagent and measured at 620 nm using a Novaspec II spectrophotometer (Biochrom Ltd., Cambridge, UK).
Statistical analysis
All data were expressed as means ± SEM. Statistical comparison of the differences between the means of control and experimental groups was performed with GraphPad InStat Software (version 4.00, GraphPad Software, San Diego, CA, USA), using one-way analysis of variance (ANOVA), followed by Tukey–Kramer multiple comparison test. A value of p < 0.05 was considered significant.
RESULTS
Structure of OA
Spectroscopic analyses of the white powder obtained after recrystallization of S. aromaticum EAS with ethanol carried out using 1H- and 13C-NMR (1D and 2D) spectroscopy that was compared with literature dataCitation20 confirmed the structure of OA.
The purity (about 99%) of OA was elucidated on the basis of chemical and physicochemical evidence.
Effects of OA on blood glucose
By comparison with control nondiabetic rats, the mean fasting blood glucose concentration (time 0) of control STZ-induced diabetic rats was significantly higher as compared to the control nondiabetic animals (3.26 ± 0.10 vs. 21.25 ± 0.25 mmol L−1, n = 6 in both groups). The blood glucose concentration of control nondiabetic and STZ-induced diabetic rats increased for 60 min following glucose load before declining to respective, preloading values by the end of the experimental period (). Administration of OA at various doses (40, 80, and 120 mg kg−1) significantly (p < 0.05) reduced the blood glucose of nondiabetic and STZ-induced diabetic rats by 45 min (). The blood glucose concentration in OA-treated STZ-induced rats persisted declining below preloading values by the end of the 4-h period whilst in nondiabetic animals compared to preloading values at the corresponding time. Comparison of the blood glucose-lowering effects of OA doses at each time could not be separated statistically, although effects appear to be dose-dependent in STZ-induced diabetic rats. All standard hypoglycemic drugs (insulin, glibenclamide, and metformin) demonstrated blood glucose-lowering effects in nondiabetic and STZ-induced diabetic rats, except glibenclamide, the insulin secretagogue which did not exhibit any antihyperglycemic effects in STZ-induced diabetic animals ().
FIGURE 1. OGT responses of nondiabetic rats (a and b) and STZ-induced diabetic (c and d) rats to graded doses of OA with respective untreated control or positive control animals. Values are presented as mean ± SEM (n = 6 in each group). ☆p < 0.05 by comparison with control animals.
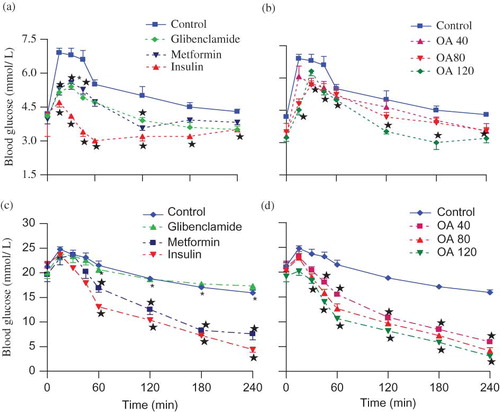
compare the influence of insulin (100 or 200 μg kg−1, s.c.), glibenclamide (250 or 500 μg kg−1, p.o.), and metformin (250 or 500 mg kg−1, p.o.) on the effects of OA (40 or 80 mg kg−1) on blood glucose in nondiabetic and STZ-induced diabetic rats. Blood glucose concentrations were significantly (p < 0.05) low at all the time points in animals treated with combined OA and insulin when compared with rats treated separately with either insulin or OA. The blood glucose-lowering effects of combined OA (40 or 80 mg kg−1) with insulin (100 or 200 μg kg−1, s.c.), glibenclamide (250 or 500 μg kg−1, p. o), and metformin (250 or 500 mg kg−1, p.o.) revealed that the effects of this narrow range of doses could not be separated statistically (). More notable, however, were the dramatic effects produced by combined OA (80 mg kg−1) and insulin (200 μg kg−1) treatment in STZ-induced diabetic rats where the blood glucose concentrations decreased to hypoglycemic levels (2.53 ± 0.22 mmol L−1, n = 6 in each group) by the end of the 4-h experimental period ().
FIGURE 2. Comparison of OGT responses to OA alone and in combination with standard antidiabetic drugs in separate groups of nondiabetic rats (a and b) and STZ-induced diabetic (c and d) rats with respective control animals. Values are presented as means ± SEM (n = 6 in each group). ☆p < 0.05 by comparison with respective control animals.
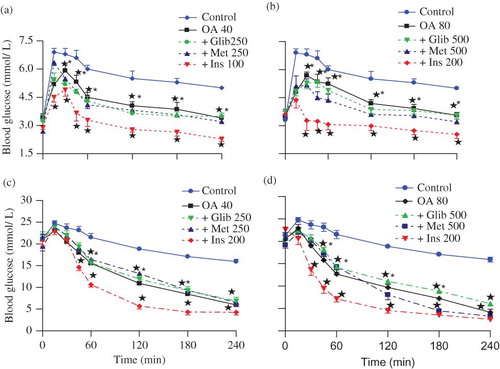
By the end of 5 weeks, treatment with OA and/or standard antidiabetic drugs (insulin and metformin) reduced blood glucose of STZ-induced rats, but the blood glucose of nondiabetic rats was not altered by any of the treatments.
OA effects on insulin secretion
Plasma insulin concentrations in untreated STZ-induced diabetic rats were significant (p < 0.05) in comparison with control nondiabetic rats (). Acute OA administration did not alter plasma insulin concentrations of STZ-induced diabetic animals, but slightly increased plasma insulin concentrations in nondiabetic rats to values that did not achieve statistical significance. Acute treatment with insulin alone significantly (p < 0.05) elevated plasma insulin concentration of both groups of nondiabetic and STZ-induced rats by comparison with respective control animals (126.90 ± 2.07 vs. 431.92 ± 23.73 pmol L−1 and 24.52 ± 0.56 vs. 33.76 ± 1.33 pmol L−1, respectively). Treatment with insulin alone or in combination with OA evoked significant (p < 0.05) increases in plasma insulin concentrations in nondiabetic and STZ-induced diabetic rats by comparison with respective control animals, but comparable with animals administered insulin alone. The administration of OA and/or insulin over a 5-week period evoked similar plasma insulin concentrations described for acute treatments.
FIGURE 3. Comparison of acute (a and b) and short-term (c and d) effects of OA and standard antidiabetic drugs on plasma insulin and glucose concentrations in nondiabetic and STZ-induced diabetic rats with respective control groups. Blood samples were collected 60 min after glucose load. Values are presented as means ± SEM (n = 6 in each group). ☆p < 0.05 by comparison with respective control animals; #p < 0.05 by comparison with respective nondiabetic animals.
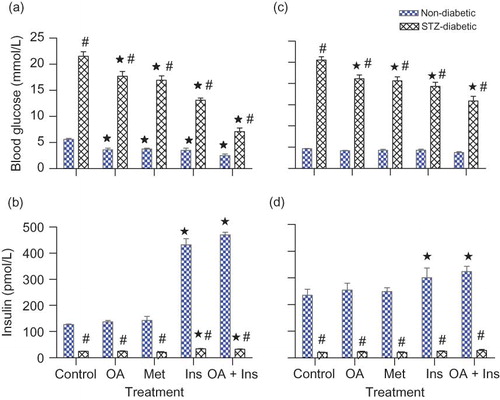
In summary, blood glucose concentrations of both nondiabetic and STZ-induced diabetic rats were decreased by OA. The hypoglycemic effects were most significant in STZ-induced rats treated with combined OA and insulin by comparison with all other treatments.
Effects of OA on body weight
Nondiabetic control rats progressively gained weight whereas STZ-induced diabetic rats exhibited severe wasting throughout the 5-week period. OA administration reduced the body weight loss of STZ-induced diabetic rats in the fifth week, whereas treatment with metformin, insulin or combined insulin, and OA-stabilized body weight of STZ-induced diabetic rats from the third to the fifth week.
Effects of OA on glycogen concentration
STZ-induced diabetic rats were characterized by the depletion of liver and muscle glycogen concentrations at the end of the 5-week experimental period (). OA treatment for 5 weeks like insulin or metformin restored the glycogen concentration of STZ-induced diabetic rats to levels that compare with nondiabetic rats. Combined OA and insulin administration, however, had more pronounced glycogen concentration restoring effect by comparison to all groups of treatments.
TABLE 1. Comparison of hepatic and muscle glycogen concentrations of various groups of nondiabetic and STZ-induced diabetic rats treated with OA and/or standard antidiabetic drugs with respective control groups
In summary, short-term OA treatment decreased blood glucose concentrations of STZ-induced diabetic rats with concomitant restoration of hepatic and muscle glycogen content to levels that comparable to levels with nondiabetic rats.
DISCUSSION
The experimental evidence presented in this study suggests that OA isolated from Syzygium aromaticum (cloves) acts in synergy with insulin to lower blood glucose of STZ-induced diabetic rats. Citation1H- and Citation13C-NMR (1D and 2D) spectroscopy studies confirmed the structure of the isolated OA by the olefinic bond between carbon 12 and carbon 13 peculiar to triterpenoids and the spectral data that compared with that previously reported for OA.Citation20
The plasma insulin concentrations in STZ-induced diabetic rats were significantly (p < 0.05) lower than in nondiabetic rats perhaps because of the destruction of pancreatic β-cells by STZ.Citation21 Acute OA treatment decreased blood glucose of both nondiabetic and STZ-induced diabetic rats without any significant effects on plasma insulin concentrations despite reports that OA increases insulin secretion isolated rat islets and pancreatic β-cells.Citation9,Citation11,Citation22,Citation23 OA administration, however, slightly increased plasma insulin concentrations in nondiabetic rats to values that did not achieve statistical significance. Interestingly, combined OA and insulin treatment elevated plasma insulin concentrations in STZ-induced diabetic and nondiabetic rats, although the magnitude in the increase of insulin concentration was far much greater in nondiabetic rats. The discrepancy can partly be attributed to the presence or absence of β-pancreatic cell activity. OA has been reported to modulate pancreatic β-cells to increase insulin levels and secretion.Citation10,Citation24 Because STZ selectively destroys β-cells of the pancreas,Citation21 we would not expect OA to increase insulin levels in STZ diabetic rats. We thus suggest that OA evoked the β-cells of the pancreas to secrete in nondiabetic rats to increase and decrease blood glucose. Sato et al.,Citation10 however, suggested that the direct effects of OA on β-cell insulin secretion may not only account for its antidiabetic properties. This point is underscored by the observation that S. aromaticum crude extract depresses the activities of hepatic gluconeogenic enzymes phosphoenolpyruvate carboxykinase (PEPCK) and glucose 6-phosphatase (G6Pase)Citation25 thereby inhibiting hepatic glucose production. Overexpression of hepatic PEPCK is associated with diabetic-like syndrome in experimental animals.Citation26
Short-term treatment of STZ-induced diabetic rats with OA significantly (p < 0.05) reduced blood glucose concentrations to levels that did not achieve normoglycemia but had no effect in nondiabetic animals. The failure to record normoglycemic values may partly be attributed to experimental design and data presentation. The blood glucose concentrations were measured 24 h after the last dosing and presumably by that time OA may have been metabolized and rendered pharmacologically ineffective. The peak hypoglycemic effect of triterpene containing herbal extracts occurs 2–4 h after administration, with blood glucose returning to pretreatment levels in 6–10 h.Citation27 Interestingly, combined OA and insulin had an even greater effect in lowering blood glucose than their individual effects, suggestive of a synergistic effect of the two. We suggest that OA in part increased insulin sensitivity to OA. Herbal extracts containing triterpenoids, UA, and corosolic acid have been reported to enhance the blood glucose-lowering effects of insulin in experimental animals.Citation28–30
STZ-induced diabetic rats were characterized by hyperglycemia and the depletion of liver and muscle glycogen concentrations perhaps because of low insulin levels caused by the destruction of pancreatic β-cells by STZ.Citation21 OA restored the glycogen concentration of STZ-induced diabetic rats to levels that compared with nondiabetic rats perhaps due to increases in noninsulin-dependent cellular uptake.Citation31–33 The effects of OA on muscle glycogen synthesis in the STZ-induced diabetic rats were perhaps due to OA's insulin mimetic properties as muscle glycogen synthesis is dependent on insulin.Citation34 On the contrary, glycogen synthesis in skeletal tissues is dependent on insulin which stimulates translocation of the GLUT-4 to the cell membrane to mediate its uptake.Citation35–37 Therefore, combined OA and insulin had an even greater effect, suggestive of a synergistic effect of the two.
By comparison with nondiabetic animals, STZ-diabetic rats progressively lost body weight and drank more water throughout the experimental period comparing with our previous observations.Citation2 Polydipsia and increased water intake as a result of enhanced proteolysis associated with hyperosmotic dehydration has been shown to be associated with weight losses in STZ-induced diabetic rats.Citation38,Citation39 Treatment with OA alone or in combination with insulin stabilized body weight of STZ-induced diabetic rats possibly due to increased incorporation of glucose into tissues, as reported in STZ-induced diabetic mice.Citation40,Citation41
In conclusion, our data suggest a synergistic effect between S. aromaticum-derived OA and insulin in lowering blood glucose of STZ-induced diabetic rats. The observed improvement of blood glucose levels and body weight in STZ-induced diabetic rats after OA treatment suggests beneficial effects in diabetes management. The limitations of the study include the absence of lipid profile and liver function assessment. In this regard, it is envisaged to utilize the obese Zucker diabetic rat model in future studies.
Acknowledgments
This study was partly funded by the University of KwaZulu-Natal, Research Division. The authors are grateful to R. Myburg of the School of Medical Sciences, University of KwaZulu-Natal for insulin assay and Mr. O.O. Oyedeji, School of Chemistry, University of KwaZulu-Natal for assistance with phytochemical studies and the Biomedical Research Unit, University of KwaZulu-Natal for the supply of animals.
Declaration of interest: The authors report no conflicts of interest. The authors alone are responsible for the content and writing of this paper.
REFERENCES
- Bwititi P, Musabayane CT. The effect of plant extracts on plasma glucose levels in rats. Acta Med Biol (Japan). 1997;45(4):167–169.
- Bwititi P, Musabayane CT, Nhachi CFB. Effects of Opuntia megacantha on blood glucose and kidney function in streptozotocin diabetic rats. J Ethnopharmacol. 2000;69(3):247–252.
- Jung M, Park M, Lee HC, Kang YH, Kang ES, Kim SK. Antidiabetic agents from medicinal plants. Curr Med Chem. 2006;13:1203–1218.
- Kim JD, Kang SM, Park MY, Jung TY, Choi HY, Ku SW. Ameliorative anti-diabetic activity of Dangnyosoko, a Chinese herbal medicine in diabetic rats. Biosci Biotechnol Biochem. 2007;71(6):1527–1534.
- Wang TG, Ng TB. Natural products with hypoglycemic, hypotensive, hypocholestrolemic, anti atherosclerotic and antithrombotic activities. Life Sci. 1999;65(25):2663–2677.
- Grover JK, Yadav S, Vats V. Medicinal plants of India with antidiabetic potential. J Ethnopharmacol. 2002;81:81–100.
- Liu J. Pharmacology of oleanolic acid and ursolic acid. J Ethnopharmacol. 1995;49(2):57–68.
- Musabayane CT, Mahlalela N, Shode FO, Ojewole JAO. Effects of Syzygium cordatum (Hochst.)[Myrtaceae] leaf extract on plasma glucose and hepatic glycogen in streptozotocin-induced diabetic rats. J Ethnopharmacol. 2005;97:485–490.
- Hsu JH, Wu YC, Liu IM, Cheng JT. Release of acetylcholine to raise insulin secretion in Wistar rats by oleanolic acid, one of the bioactive principles contained in Cornus officinalis. Neurosci Lett. 2006;404:112–116.
- Sato H, Genet C, Strehle A, Anti-hyperglycemic activity of a TGR5 agonist isolated from Olea europaea. Biochem Biophys Res Commun. 2007;4(3):793–798.
- Teodoro T, Zhang L, Alexander T, Yue J, Vranic M, Volchuk A. Oleanolic acid enhances insulin secretion in pancreatic ß-cells. FEBS Lett. 2008;582:1375–1380.
- Zhang YN, Zhang W, Hong D, Oleanolic acid and its derivatives: New inhibitor of protein tyrosine phosphates 1B with cellular activities. Bioorg Med Chem. 2008;16(18):8697–8705.
- Wang B, Jiang ZH. Studies on oleanolic acid. Chin Pharm J. 1992;27:393–397.
- Perez-Camino MC, Cert A. Quantitative determination of hydroxyl pentacyclic triterpene acids in vegetable oils. J Agric Food Chem. 1999;47(4):1558–1562.
- Liu J. Oleanolic acid and ursolic acid: Research perspectives. J Ethnopharmacol. 2005;100(1–2):92–94.
- Mapanga RF, Tufts MA, Shode FO, Musabayane CT. Renal effects of plant-derived oleanolic acid in streptozotocin-induced diabetic rats. Ren Fail. 2009;31(6):481–491.
- Somova LI, Shode FO, Nadar A, Ramnanan P. Antihypertensive, antiatheroscleritic and antioxidant activity of triterpenoids isolated from Olea europaea, subspecies africana leaves. J Ethnopharmacol. 2003;84:299–305.
- Musabayane CT, Gondwe M, Kamadyaapa DR, Chuturgoon AA, Ojewole JAO. Effects of Ficus thonningii (Blume)[Moraceae] stem-bark ethanolic extract on blood glucose, cardiovascular and kidney functions of rats, and on kidney cell lines of the proximal (LLC-PK1) and distal tubules (MDBK). Ren Fail. 2007;29(4):389–397.
- Ong KC, Khoo HE. Effects of myricetin on glycaemia and glycogen metabolism in diabetic rats. Life Sci. 2000;67:1695–1705.
- Mahato SB, Kundu AP. 13C NMR spectra of pentacyclic triterpenoids-a complication and some salient features. Phytochemistry. 1994;37:1517–1573.
- Szkudelski T. The mechanism of alloxan and streptozotocin action in B cells of the rat pancreas. Physiol Res. 2001;50(6):537–546.
- Gao D, Li Q, Li Y, Antidiabetic potential of oleanolic acid from Ligustrum lucidum Ait. Can J Physiol Pharmacol. 2007;85(11):1076–1083.
- Lin Z, Zhang, Y, Zhang Y, Oleanolic acid derivative NPLC441 potently stimulates glucose transport in 3T3-L1 adipocytes via a multi-target mechanism. Biochem Pharmacol. 2008;76(10):1251–1262.
- Jeong DW, Kim YH, Kim HH, Dose-linear pharmacokinetics of oleanolic acid after intravenous and oral administration in rats. Biopharm Drug Dispos. 2007;28:51–57.
- Prasad RC, Herzog B, Boone B, Sims L, Waltner-Law M. An extract of Syzgium aromaticum represses genes encoding gluconeogenic enzymes. J Ethnopharmacol. 2005;96:295–301.
- Valera A, Rodriguez-Gill JE, Bosch F. Vanadate treatment restores the expression of genes for the key enzymes in the glucose and ketone bodies metabolism in the liver of diabetic rats. J Clin Invest. 1993;92:4–11.
- Kakuda T, Sakane I, Takihara T, Ozaki Y, Takeuchi H, Kuroyanagi M. Hypoglycemic effect of extracts from Lagerstroemia speciosa L. leaves in genetically diabetic KK-AY mice. Biosci Biotechnol Biochem. 1996;60(2):204–208.
- Hayashi T, Maruyana H, Kasai R, Ellagatannins from Lagerstroemia speciosa as activators of glucose transport in fat cells. Planta Med. 2002;68:173–175.
- Miura T, Itoh Y, Kaneko Y, Corosolic acid induces GLUT-4 translocation in genetically type 2 diabetic mice. Biol Pharm Bull. 2004;27(7):1103–1105.
- Jung SH, Ha YJ, Shim EK, Insulin-mimetic and insulin-sensitizing activities of a pentacyclic triterpenoid insulin receptor activator. Biochem J. 2007;403:243–250.
- Buschiazzo H, Exton JH, Park CR. Effects of glucose on glycogen synthase, phosphorylase and glycogen deposition in the perfused rat liver. Proc Natl Acad Sci U S A. 1970;65(2):383–387.
- Libal-Weksler Y, Gotlibovitz O, Stark AH, Madar Z. Diet and diabetic state modify glycogen synthase activity and expression in rat hepatocytes. J Nutr Biochem. 2001;12:458–464.
- Tana BKH, Tanb CH, Pushparaj PN. Anti-diabetic activity of the semi-purified fractions of Averrhoa bilimbi in high fat diet fed-streptozotocin-induced diabetic rats. Life Sci. 2005;76:2827–2839.
- Daisy P, Balasubramaniam K, Rajalakshmi M, Eliza J, Selvaraj J. Insulin mimetic impact of catechin isolated from Cassia fistula on the glucose oxidation and molecular mechanisms of glucose uptake on streptozotocin-induced diabetic Wistar rats. Phytomedicine. 2009;17:28–36.
- Barnard RJ, Youngren JF. Regulation of glucose transport in skeletal muscle. FASEB J. 1992;6:3238–3244.
- Bouché C, Serdy S, Kahn CR, Goldfine AB. The cellular fate of glucose and its relevance in type 2 diabetes. Endocr Rev. 2004;25(5):807–830.
- Wiernsperger NF. Is non-insulin dependent glucose uptake a therapeutic alternative? Part 2: Do such mechanisms fulfil the required combination of power and tolerability? Diabetes Metab. 2005;31:521–525.
- Rebsomen L, Pitel S, Boubred F, C-peptide replacement improves weight gain and renal function in diabetic rats. Diabetes Metab. 2005;32:223–228.
- Narendhirakannan RT, Subramanian S, Kandaswamy M. Biochemical evaluation of anti-diabetogenic properties of some commonly used Indian plants on streptozotocin-induced diabetes in experimental animals. Clin Exp Pharmacol Physiol. 2006;33(12):1150.
- Naik SR, Barbosa Filho JM, Dhuley JN, Deshmukh V. Probable mechanism of hypoglycaemic activity of basic acid, a natural product isolated from Bumelia sartorum. J Ethnopharmacol. 1991;33(1–2):37–44.
- Okine LK, Nyarko AK, Osei-Kwabena N, Oppong IV, Barnes F, Ofosuhene M. The anti-diabetic activity of the herbal preparation ADD-199 in mice: A comparative study with two oral hypoglycaemic drugs. J Ethnopharmacol. 2005;97(1):31–38.