Abstract
Background and aims: It has been well documented that endothelial progenitor cells (EPCs) participate in neovascularization in adults and that rarefaction of peritubular capillaries (PTCs) is closely associated with progressive kidney disease. Therefore, we investigated whether EPCs were influenced by conditioned medium (CM) of renal tubular epithelial cells (RTECs) stimulated by hypoxia, to provide evidence for EPCs transplantation in vivo in the future. Methods: Mononuclear cells of rat bone marrow were isolated by density gradient centrifugation and were cultured according to previously described techniques. RTECs were cultured primarily with routine tissue block adhering wall method. In addition, CM was harvested from RTECs cultivated for 48 h in 5% O2. EPCs proliferation and migration were evaluated by MTT [3-(4,5-dimethylthiazol-2-yl)-2,5-diphenyltetrazolium bromide] assay and transwell. The protein and mRNA expression of stromal cell-derived factor (SDF-1), vascular endothelial growth factor (VEGF), angiogenin 1 (Ang-1), and C-X-C chemokine receptor 4 (CXCR4) was separately assessed by Western blot, enzyme-linked immunosorbent assay (ELISA), and reverse transcriptase-polymerase chain reaction (RT-PCR) methods. Results: We showed that hypoxia increased the expression of SDF-1 and VEGF in RTEC. In addition, hypoxic CM improved proliferation, migration, and expression of VEGF, Ang-1, and CXCR4 of EPCs. Conclusions: These results suggest that hypoxic CM improves neovascularization of EPCs and may also be considered as therapeutic agents to supply the potent origin of reconstituion of PTCs of progressive kidney disease.
INTRODUCTION
Recently, closely pathologic analysis shows that functional impairment of kidney is better correlated with the degree of tubulointerstitial damage than that of glomerular injury, and this finding in turn has led to the broad recognition that the final common pathway of renal failure is principally the tubulointerstitial fibrosis.Citation1 Furthermore, rarefaction of peritubular capillaries (PTC) and chronic hypoxia are crucial for the progress of tubulointerstitial fibrosis. And the former is one of the major hypoxic factors. Progressive loss of PTCs appears in multiple chronic kidney diseases (CKD). Therefore, reconstitution of PTCs that targets at chronic hypoxia should prove effective against a broad range of renal diseases.
Endothelial progenitor cells (EPCs) derived from the bone marrow contribute to the formation of new blood vessels in adults,Citation2 and they show strong proliferative potential and can form tube-like structure, when cultured in medium containing specific growth factors. EPCs mobilized endogenously and administered exogenously play a crucial role in augmenting neovascularization and endothelial replacement of ischemic tissue.Citation3 Replenishment of such cells may limit vascular injury through reconstitution of the luminal barrier and paracrine factors, which provide a novel therapeutic option. Indeed, growing experimental and clinical evidence underscores the critical role that circulating EPCs play in healing the endothelium when the intrinsic system is unable to support tissue repair adequately. For example, targeted delivery of EPCs has been shown to improve the function of the infarcted myocardium,Citation4 attenuate cerebral ischemia,Citation5 decrease hind limb ischemia,Citation6 rescue the kidney from acute ischemia injury,Citation7 and participate in preserving microvascular architecture.Citation8,Citation9 As we know, there are lowered numbers and impaired function of EPCs in CKD. Along these lines, we pursued the hypothesis that bone marrow-derived EPCs exogenously administered participate in the reconstitution of PTCs in CKD. Renal tubular cells are closely related with PTCs and influenced by hypoxia in CKD, so at the first step we analyzed the effects of conditioned medium (CM) from renal tubular epithelial cells (RTECs) stimulated by hypoxia on EPCs and possible mechanisms in vitro, providing evidence for EPCs transplantation in the future.
MATERIALS AND METHODS
EPCs isolation, culture and identification
Bone mononuclear cells were isolated from the bone marrow of 6-week-old male Sprague–Dawley rats by density gradient centrifugation with Histopaque 1083 (Sigma, St. Louis, MO, USA). Mononuclear cells were cultured at a density of 1 × 106 cells in a 24-well dish coated with human fibronectin in M199 supplemented with 1 μg/mL hydrocortisone, 3 μg/mL bovine brain extract, 10 ng/mL vascular endothelial growth factor (VEGF), 10 ng/mL basic fibroblast growth factor, and 20% fetal calf serum. After 4 days, nonadherent cells were removed by washing and the cultures were maintained. On the seventh day, to identify endothelial cell-like cells, the adherent cells were incubated with 1, 1′-dioctadecyl-3,3,3′, 3′-tetramethyl-indocarbocyanine-labeled acLDL (Dil-acLDL) Molecule Probes (Invitrogen, Paisley, UK), and then fixed in 2% paraformaldehyde and counterstained with fluorescein isothiocyanate (FITC)-labeled lectin from ulex europaeus agglutinin 1 (UEA-1) (Sigma). The fluorescent images were recorded under a laser scanning confocal microscope. Cells were also characterized by immunofluorescence staining for the expression of CD34, CD133, and VEGF receptor 2 (VEGFR-2). Cells that were positive for both DiI-acLDL and FITC-UEA-1 were judged as endothelial-like cells as previously described.Citation8,Citation10,Citation11
RTECs culture
Primary tubular epithelial cells were isolated from the kidneys of 3-week-old rats as previously described with routine tissue block adhering wall method and grown in M199 supplemented with 10% fetal calf serum. Primary cells were characterized by immunofluorescence with anticytokeratin (Sigma) and anti-α-smooth muscle actin (Santa cruz, CA, USA) according to standard procedures.Citation12
Preparation of conditioned medium
The third and fourth passage RTECs were cultured under normoxic conditions until 70–80% were confluent, and the medium was changed into serum-free M199 for another 24 h, then were subjected to normoxic or hypoxic conditions (5% O2, 5% CO2, 95% humidity) for 48 h (based on preliminary experiments) in an hypoxia chamber (Heraeu, Germany). Finally, supernatants were collected, centrifuged to remove cellular debris, and passed through 0.22-μm pore filters (Millipore, Tokyo, Japan).Citation13
EPCs proliferation assay
The 3-(4,5-dimethylthiazol-2-yl)-2,5-diphenyltetrazolium bromide (MTT) assay was used to determine EPCs proliferation. 1.0 × 104 cells per well were seeded onto 96-well plates with 20 μL of cell culture medium. The cells were synchronized by 24 h serum deprivation and were treated with hypoxic and normoxic CM from RTECs. The plates were incubated at 37°C, 5% CO2 for 12, 24, 48, and 96 h, and then MTT assay was carried out. Briefly, about 20 μL of MTT dye (5 mg/mL) was added to each well. After 4-h incubation, 150 μL of dimethylsulfoxide was added to each well. The absorbency (A) was measured at 550–650 nm.
EPCs migration assay
Migration assay was performed in 24-well-fitted inserts with membranes (8-μm pore size, Costar, Cambridge, MA, USA). EPCs (1 × 105 per well) were plated in transwell chambers with serum-free M199 medium. The lower well was placed with the CM containing 5% fetal calf serum. After incubation for 6, 12, and 24 h, cells on the upper well were completely removed by cotton swab, and the migration cells were tested by an MTT colorimetric assay.
RNA extraction and RT-PCR
Total cellular RNA from 5 × 106 cells was extracted using Trizol reagent (Gibco-BRL, Grand Island, NY, USA) according to the manufacturer's instructions. The first-strand cDNA was synthesized with Superscript II reverse transcriptase (Invitrogen, Gaithersburg, MD, USA). Specific polymerase chain reaction (PCR) primer pairs and the different parameters of amplification are summarized in . PCR products were resolved on agarose gels, visualized by ethidium bromide, and analyzed using QuantityOne software (Bio-Rad, Hercules, CA, USA).
TABLE 1. The sequences for specific PCR primer pairs and the different parameters for PCR amplification
Western blot analysis
EPCs were lysed at 4°C in 50 mM tris-HCL, 150 mM NaCl, pH 8, 0.25% deoxycholic acid, 1% Triton X-100, 1 mM ethylene glycol tetraacetic acid (EGTA), 1 mM phenylmethyl sulfonyl fluoride (PMSF), and protease inhibitors (Complete; Roche, Indianapolis, IN, USA). Protein extracts were quantified using the bicinchoninic acid (BCA) protein assay kit. Equal amounts of protein (30 μg/Lane) were separated by sodium dodecyl sulfate–polyacrylamide gel electrophoresis (SDS–PAGE), transferred to polyvinylidene difluoride (PVDF) membranes (Millipore, Bedford, MA, USA), and probed with 0.5 μg/mL rabbit anti-SDF-1, anti-Ang-1, anti-VEGF, and anti-β-actin antibodies (Santa Cruz, CA, USA). Antibody binding was visualized using horseradish peroxidase-conjugated anti-IgG Fab2 and chemiluminescence (Pierce, MN, USA), and analyzed using QuantityOne software (Bio-Rad).Citation14
Enzyme-linked immunosorbent assay
Based on preliminary experiments, we separately collected CM of renal tubular cells in 20% O2 (control group), 8% O2, 5% O2, 3% O2, 1% O2 for 48 h. The absolute SDF-1 and VEGF concentrations in CM (supernatant of cultured renal tubular cells) were determined using a standard curve for SDF-1 and VEGF according to the manufacturer's recommendations.
Statistical analysis
All results were expressed as means ± SD. Comparisons of three or more groups were performed by analysis of variance (ANOVA) with Dunnett's post-test. Comparisons between two groups were performed by t-test. In all cases, all values of p < 0.05 were taken to denote statistical significance. All analyses were performed using SPSS 13.0 software (SPSS Inc, Chicago, IL, USA).
RESULTS
Characterization of EPCs
After 7 days in culture, attached cells exhibited a spindle-shaped, endothelial cell-like morphology (A). Adherent bone marrow cells had incorporated acetylated low-density lipoprotein (LDI) and stained positive for FITC lectin (B–D). EPCs from various sources have been shown to have these characteristics. EPCs also expressed VEGFR-2, a marker for endothelial-lineage cells (E), and CD34 (F) and CD133 (G), markers of stem cells.
FIGURE 1. Characterization of EPCs. (A) After 7 days in culture, attached cells exhibited a spindle-shaped endothelial cell-like morphology (original magnification × 200). (B, C, D) Adherent cells, lectin-binding (green), DiLDL uptake (red), double positive (orange) were assessed under a laser-scanning confocal microscope (original magnification × 400). The cells were further analyzed by immunofluorescence with (E) VEGFR-2, (F) CD34, and (G) CD133 antibodies (original magnification × 400).
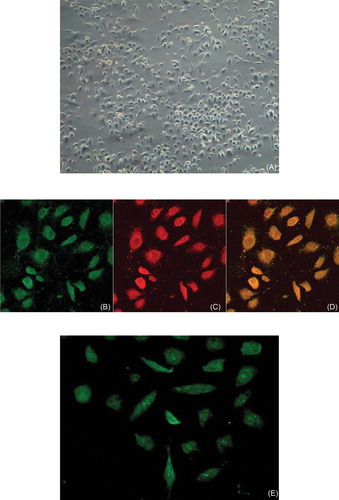
Effect of CM on EPCs proliferation and migration
Exposure of EPCs to hypoxic CM of renal epithelial cells ameliorated the proliferation of EPCs (p < 0.05), reaching the peak at 48 h (). Hypoxic CM also increased the migration of EPCs (p < 0.05), reaching the peak at 12 h ().
FIGURE 2. Effect of CM on EPCs proliferation. Hypoxic CM time-dependently increased the proliferation of EPCs. Proliferative activity of EPCs became apparent at 24 h and reached the maximum at 48 h; C (control group): conditioned medium from normoxic condition; CM: conditioned medium from hypoxic condition; A, light absorbance. Data are presented as the mean ± SD. n = 5. *p < 0.05, **p < 0.01 compared with control group 123 × 70 mm.
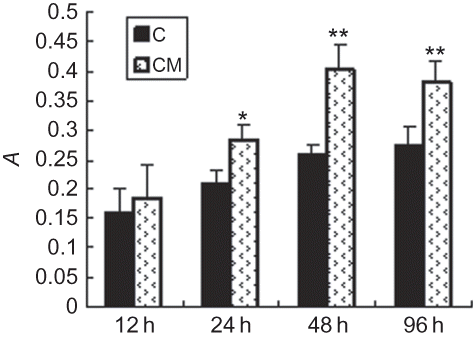
FIGURE 3. Effect of hypoxic CM on EPCs migration. CM time-dependently increased the migration of EPCs. Increase of EPCs migratory capacity became apparent after 6 h and reached the maximum at 12 h; C (control group): conditioned medium from normoxic condition; CM: conditioned medium from hypoxic condition; A, light absorbance. Data are presented as the mean ± SD. n = 5. *p < 0.05, **p < 0.01 compared with control group.
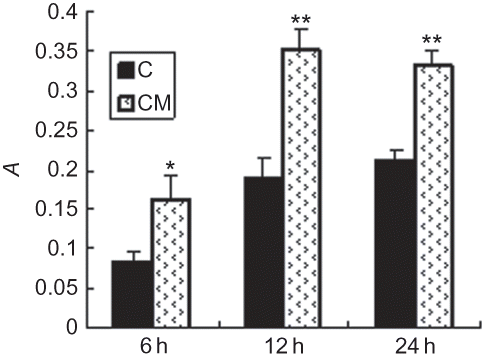
VEGF production by EPCs
To explore the possible paracrine and autocrine contributions of EPCs to vascularization, we examined whether EPCs secrete VEGF. EPCs did produce VEGF and the amount of VEGF markedly increased as compared with control group (), which suggested that angiogenic potential of EPCs had been selectively improved under our culture conditions.
FIGURE 4. Effect of CM on VEGF protein expression. Production of VEGF protein by EPCs in CM group was significantly greater than that in control group and reached the maximum at 48 h. C (control group): conditioned medium from normoxic condition; CM: conditioned medium from hypoxic condition; β-actin was used as a housekeeping protein. Data are presented as the mean ± SD. n = 5. *p < 0.05 compared with control group.
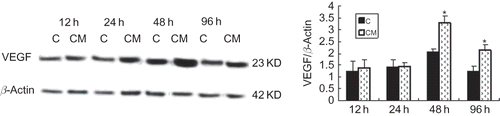
Ang-1 production by EPCs
To explore the possible paracrine and autocrine contribution of EPCs in the course of vascularization, we detected whether EPCs secrete Ang-1. EPCs also produced Ang-1, and the amount of Ang-1 markedly increased as compared to control group (), which suggested angiogenic potential of EPCs had selectively grown under our culture conditions.
FIGURE 5. Effect of CM on Ang-1 protein expression. Production of Ang-1 by EPCs in CM group was significantly greater than that in control group and became apparent and reached the maximum at 48 h. C (control group): conditioned medium from normoxic condition; CM: conditioned medium from hypoxic condition; β-actin was used as a housekeeping protein. Data are presented as the mean ± SD. n = 5. *p < 0.01 compared with control group.
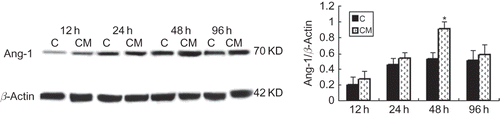
CXCR4 production by EPCs
To evaluate the effect of CM on the chemotaxis of EPCs, we measured mRNA expression of CXCR4. We found CXCR4 expression was improved at 24 h compared with the control group and reached the maximum at 48 h ().
FIGURE 6. Effect of CM on mRNA expression of CXCR4 production. RT-PCR: production of CXCR4 by EPCs in CM group was significantly greater than that in control group, and reached the maximum at 48 h. C (control group): conditioned medium from normoxic condition; CM: conditioned medium from hypoxic condition; β-actin was used as a housekeeping gene. Data are presented as the mean ± SD. n = 5. *p < 0.05, **p < 0.01 compared with control group.
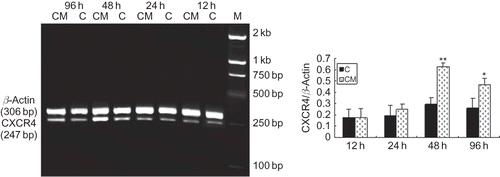
SDF-1 and VEGF production in CM
To investigate potential mechanisms of CM leading to the improvement of endothelial function, we performed ELISA and detected the expression of SDF-1 and VEGF in CM. Treatment with hypoxia significantly increased the expression of SDF-1 and VEGF in CM, which suggested that CM could influence proliferation and homing of EPCs under hypoxic condition by SDF-1 and VEGF ( and ).
FIGURE 7. SDF-1 expression in CM of cultured RTECs at different oxygen concentrations. SDF-1 expression of RTECs in hypoxic groups was significantly greater than that in control group and became apparent and reached the maximum with 5%O2 at 48 h. Data are presented as the mean ± SD. n = 5. *p < 0.05, **p < 0.01 compared with control group. C: control group (20% O2).
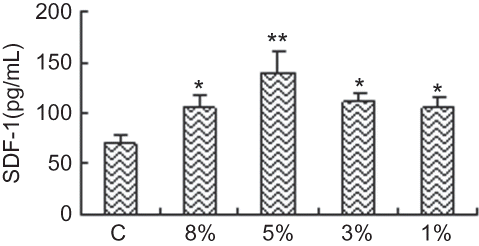
FIGURE 8. VEGF expression in CM of cultured RTECs at different oxygen concentrations. VEGF expression of RTECs in hypoxic groups was significantly greater than that in control group and became apparent and reached the maximum with 3% O2 at 48 h. Data are presented as the mean ± SD. n = 5. *p < 0.05, **p < 0.01 compared with control group. C: control group (20% O2).
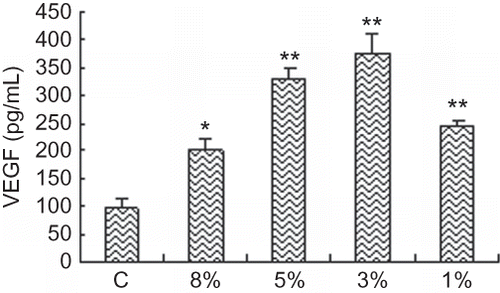
DISCUSSION
Intercellular crosstalk through soluble factors or direct cell–cell contact is a key factor in physical or pathological conditions. We hypothesized that soluble factors derived from hypoxic renal epithelial cells improved the migration, proliferation, and expression of VEGF, Ang-1, and CXCR4 of EPCs, which were associated with the angiogenic ability of EPCs.
In our study, EPCs derived from rat bone marrow could differentiate into cells which displayed classical endothelial cell morphology and characteristics, such as the capacity to take up acetylated LDL and express VEGFR-2, and also showed stem cell characteristic, such as expression of CD34 and CD133. Migratory capacity of EPCs is associated with its homing and proliferative ability with angiogenic ability. This study shows that CM of RTECs under hypoxic situation could promote the proliferative and migratory capacity of EPCs, which suggests hypoxic CM may improve the angiogenic ability of EPCs.
VEGF is probably the most important angiogenesis inducer. It has the ability to regulate key steps in angiogenesis, including proliferation and migration of endothelial cells. Overexpression of VEGF and/or its receptors promotes blood vessel formation, and VEGF inhibitors block angiogenesis.Citation15 In our study, hypoxic CM significantly improved VEGF expression of EPCs, which suggests vascularization of EPCs is dependent on autocrine or paracrine.
Ang-1 that binds to and phosphorylates the Tie-2 receptor promotes endothelial cell survival and capillary stabilization, sprouting with context-dependent ways. Simple VEGF or Ang-1 produces an incompletely compensatory response to angiogenesis. Ang-1 combining with VEGF improves vascular stability and integrity. In our study, expression of Ang-1 increased following the upregulation of VEGF. Therefore, we reasoned that EPCs transplantation is superior to simple factor for synergistic effect.
In our study, SDF-1 expression increased in RTECs under hypoxia, and CXCR4 expression of EPCs by CM upregulated. SDF1/CXCR4 axis is regarded as the most important regulator of cells homing and cell-based neovascularization. As a chemokine of the CXC subfamily originally cloned from bone marrow cell supernatants, SDF-1 plays an important role in the regulation of lots of functions of EPCs, such as cell migration, proliferation, and survival, and is also involved in fetal vasculogenesis.Citation16 From recent studies, it has gradually emerged that SDF-1 has a homing effect, recruiting EPCs from the bone marrow to damaged or ischemic tissue, or to hypoxic regions.Citation17 EPCs express CXCR4, the receptor for SDF-1, which allows homing to sites of neovascularization. With the release of SDF-1 and reversal of the marrow/periphery gradient, EPCs are mobilized in the periphery where expression of SDF-1 increases. Therefore, we reasoned that RTECs stimulated by hypoxia could improve the homing and survival of EPCs.
On the contrary, VEGF expression of RTECs was increased under hypoxic situation. In fact, the SDF-1/CXCR4 axis is regulated by angiogenic cytokines, too. The mobilization of progenitor cells with VEGF has been documented in both humans and animals,Citation18,Citation19 and VEGF signal plays an essential role in targeting marrow cells to the sites of neovascularization.Citation20 Not surprisingly, the mobilization and homing of marrow cells in response to VEGF has been shown to depend on SDF-1/CXCR4, and blocking CXCR4 with AMD3100 abrogates the angiogenic efficacy of VEGF.Citation21,Citation22 Hence, in our study, the upregulation of VEGF expression further strengthened the effect of SDF-1.
In conclusion, our findings indicate that CM from RTECs under hypoxic situation affects the vascularization of EPCs by improving the proliferation, migration, and expression of VEGF, Ang-1, and CXCR4 of the cells, and potential mechanisms are dependent on SDF-1 and VEGF secreted by RTECs. It suggests that hypoxia in CKD can induce the homing of EPCs, participating in the reconstitution of capillaries, and hence the use of EPCs transplantation represents an additional therapeutic strategy to CKD with lowered numbers and damaged function of EPCs.
Acknowledgments
The authors thank Lixia Guang and Kebing Zhang at the central laboratory of Xinqiao Hospital, the Third Military Medical University for their technical assistance.
Declaration of interest: Conflict of interest statment. None declared.
REFERENCE
- Heyman SN, Khamaisi M, Rosen S, Rosenberger C. Renal parenchymal hypoxia, hypoxia response and the progression of chronic kidney disease. Am J Nephrol. 2006; 28:998–1006.
- Delorme B, Basire A, Gentile C, Presence of endothelial progenitor cells, distinct from mature endothelial cells, within human CD 146+ blood cells. Thromb Hemost. 2005;94:1270–1279.
- Asahara T, Masuda H, Takahashi T, : Bone marrow origin of endothelial progenitor cells responsible for postnatal vasculogenesis in physiological and pathological neovascularization. Circ Res. 1999;85:221–228.
- Hamada H, Kim MK, Iwakura A. Estrogen receptors alpha and beta mediate contribution of bone marrow-derived endothelial progenitor cells to functional recovery after myocardial infarction. Circulation. 2006;114:2261–2270.
- Ohta T, Kikuta K, Imamura H, : Administration of ex vivo-expanded bone marrow-derived endothelial progenitor cells attenuates focal cerebral ischemia-reperfusion injury in rats. Neurosurgery. 2006;59:679–686.
- Urao N, Inomata H, Razvi M, Role of nox2-based NADPH oxidase in bone marrow and progenitor cell function involved in neovascularization induced by hindlimb ischemia. Circ Res. 2008;103:212–220.
- Rafii S, Lyden D. Therapeutic stem and progenitor cell transplantation for organ vascularization and regeneration. Nat Med. 2003;9:702–712.
- Chade AR, Zhu X, Lavi R, Endothelial progenitor cells restore renal function in chronic experimental renovascular disease. Circulation. 2009;119:547–557.
- Becherucci F, Mazzinghi B, Ronconi E, The role of endothelial progenitor cells in acute kidney injury. Blood Purif. 2009;27:261–270.
- Fukuda D, Sata M, Tanaka K, Nagai R. Potent inhibitory effect of sirolimus on circulating vascular progenitor cells. Circulation. 2005;111:926–931.
- Chen Q, Dong L, Wang L, Kang L, Xu B. Advanced glycation end products impair function of late endothelial progenitor cells through effects on protein kinase Akt and cyclooxygenase-2. Biochem Biophys Res Commun. 2009;381:192–197.
- Higgins DF, Biju MP, Akai Y, Wutz A, Jhonson RS, Haase VH. Hypoxic induction of CTGF is directly mediated by Hif-1. Am J Physiol Renal Physiol. 2004;287:F1223–F1232.
- Yamakawa M, Liu LX, Belanger AJ, Expression of angiopoietins in renal epithelial and clear cell carcinoma cells: Regulation by hypoxia and participation in angiogenesis. Am J Physiol Renal Physiol. 2004;287:F649–F657.
- Xu B, Chibber R, Ruggiero D, Kohner E, Ritter J, Ferro A. Impairment of vascular endothelial nitric oxide synthase activity by advanced glycation end products. FASEB J. 2003;17:1289–1291.
- Ferrara N. Vascular endothelial growth factor: Basic science. and clinical progress. Endocr Rev. 2004;25:581–611.
- Zheng H, Fu G, Dai T, Huang H. Migration of endothelial progenitor cells mediated by stromal cell-derived factor-lalpha/ CXCR4 via PI3 K/Akt/eNOS signal transduction pathway. J Cardiovasc Pharmacol. 2007;50:274–280.
- Yamaguchi J, Kusano KF, Masuo O, Stromal cell-derived factor-1 effects on ex vivo expanded endothelial progenitor cell recruitment for ischemic neovascularization. Circulation. 2003;107:1322–1328.
- Zentilin L, Tafuro S, Zacchigna S, Bone marrow mononuclear cells are recruited to the sites of VEGF-induced neovascularization but are not incorporated into the newly formed vessels. Blood. 2006;107:3546–3554.
- Kalka C, Masuda H, Takahashi T, Vascular endothelial growth factor(165) gene transfer augments circulating endothelial progenitor cells in human subjects. Circ Res. 2000;86:1198–1202.
- Li B, Sharpe EE, Maupin AB, VEGF and PIGF promote adult vasculogenesis by enhancing EPC recruitment and vessel formation at the site of tumor neovascularization. FASEB J. 2006;20:1495–1497.
- Grunewald M, Avraham I, Dor Y, VEGF-induced adult neovascularization: recruitment, retention, and role of accessory cells. Cell. 2006;124:175–189.
- Jin DK, Shido K, Kopp HG, Cytokine-mediated deployment of SDF-1 induces revascularization through recruitment of CXCR4+ hemangiocytes. Nat Med. 2006;12:557–567.