Abstract
Introduction: Peritoneal fibrosis is a common complication of peritoneal dialysis (PD) although the pathway involved is unclear. Of this article, angiotensin II (Ang II)-mediated upregulation of mitogen-activated protein kinase (MAPK) pathway as well as their downstream profibrotic genes including transforming growth factor (TGF)-β1 and fibronectin (FN) was investigated. Methods: Rat peritoneal mesothelial cells (RPMCs) were obtained by enzymatic digestion from the colic omentum. After incubated with Ang II, real-time PCR, ELISA, and Western blot analysis were used to determine RPMCs cellular and secretory (supernatants) levels of TGF-β1, FN, tissue inhibitor of metalloproteinase-1 (TIMP-1), and plasminogen activator inhibitor-1 (PAI-1) as well as the phosphorylation of extracellular signal-regulated kinase (ERK1/2), p38 MAPK, and stress-activated protein kinase/c-Jun NH2-terminal kinase (JNK). We also determined the downstream pathways using the specific inhibitors including PD98059 (ERK1/2), SB230580 (p38 MAPK), SP600125 (JNK), and losartan [Ang II type-1 (AT1] receptor blocker). Results: Ang II increased mRNA and protein levels of TGF-β1, FN, TIMP-1, and PAI-1 in a time- and dose-dependent manner in RPMCs. Ang II induced a 1.5–2-fold increase in both mRNA and protein levels of the above molecules at 10 nmol/L. Ang II also upregulated the phosphorylation of ERK1/2 and p38 but not of JNK. Finally, inhibition of either AT1 or ERK1/2 was able to suppress Ang II-induced expression of FN. Conclusion: In cultured RPMCs, Ang II upregulated profibrotic signaling pathways through AT1-mediated ERK1/2 phosphorylation.
One of the most serious complications after long-term continuous dialysis is peritoneal fibrosis that often leads to failure in peritoneal dialysis (PD).Citation1 Peritoneal fibrosis is characterized as exfoliation of peritoneal mesothelial cell layer and peritoneum thicken due to deposition of extracellular matrix (ECM). Peritoneal mesothelial cells are the major components of peritoneum, which will be exposed to unphysiologic dialysates during prolonged PD maneuvers. In addition to the infectious factors, many biologically incompatible factors in dialysates such as low pH, high-carbohydrate, hyperosmotic, lactate buffers, and glucose degradation products would not only affect celiac protective mechanisms but also cause damage to the peritoneal mesothelial cells, which will lead to exfoliation.Citation2 Microvilli would then be decreased and the cells would become prismatical. Early injuries of chorions are shown as formation of prismatical mesothelial cells.Citation3 Chronic irritation of unphysiologic dialysates and continuous PD-related infection are initiating factors of peritoneal fibrosis. Fibronectin (FN) and laminin (LN), together with some factors that cause fibrosis such as transforming growth factor β1 (TGF-β1), connective tissue growth factor (CTGF), and vascular endothelial growth factor (VEGF) are secreted by injured mesothelial cells.Citation4,Citation5 Overexpression of these factors interferes with the metabolism of ECM, causes ECM to be accumulated, thus leading to peritoneal fibrosis in the end.Citation5 It is shown that TGF-β1 plays an essential role in peritoneal fibrosis.Citation6
Renin–angiotensin system (RAS) in local tissue plays an important role in the adjustment of blood pressure, electrolytes balance, and blood volume.Citation7 It is convinced that the fibrogenesis in some major organs such as the kidney, heart, and lungs are mainly caused by overactivation of RAS in local tissue.Citation8,Citation9 Many investigations show that pathological changes in peritoneal fibrosis are greatly improved by angiotensin-converting enzyme inhibitors (ACEIs) or angiotensin receptor blocking (ARB) agents, which support the view that angiotensin II (Ang II) is involved in the process of peritoneal fibrosis.Citation10–12 However, the mechanism of regulatory effect of Ang II on production of ECM still remained unclear. Here, we investigated the effects of Ang II and its downstream signaling pathways on profibrotic phenotype of rat peritoneal mesothelial cells (RPMCs).
MATERIALS AND METHODS
Cells culture experiments
Male Sprague–Dawley (SD) rats (about 200 g in weight) were provided by the Experimental Animal Center of Surgical Department, Medical School of Shanghai Jiaotong University. For isolating RPMCs, every time we sacrificed one SD rat. RPMCs were isolated according to Stylianou introductionCitation13 and were identified by phase contrast microscope (OLYMPUS, CK2, PA, USA), indirect immunofluorescence includes Cytokeratin (Sigma, MO, USA), factor VIII (Sigma), and vimentin (Zhongshan Biotechnology, Beijing, PR China) monoclonal antibody, and transmission electron microscopy (PHILIP, CM—120, Amsterdam, Holland).
Measurement of TGF-β1
For the measurement of TGF-β1, 1 × 105 RPMCs were inoculated into a 6-pore plate. When 70–80% confluence was achieved, the medium was changed to serum-free Dulbecco's modified Eagle's medium (DMEM)/F12 culture fluid for 24 h to achieve growth synchronization. Finally, Ang II of varied concentrations (0, 1, 10, 100, 1000 nmol/L) were added in and cells were harvested 24 h later. Meanwhile, we examined whether treatment of cells with different agents affect cell proliferation and viability. We found that both cell proliferation rate and viability did not change among different culture conditions that we used in the study. Real-time PCR and Western blot analysis were used to evaluate the expression of TGF-β1 mRNA and protein in RPMCs undergoing stimulation with Ang II of different concentrations and ELISA was used to detect the secretion of TGF-β1 in RPMCs culture fluid. However, we repeat experiments at least thrice from three different rats.
Real-time PCR
Extraction of total RNA was manipulated according to the instruction of TRIzol Reagent. Two micrograms RNA was reverse transcribed into cDNA by RT-PCR kit (Takara, Japan). The TGF-β1 mRNA expression in RPMC was detected by using real-time quantitative PCR (MJ Research, MA, USA). The quantitative PCR reagents included SYBR GREEN Mix (TOKOB, Japan) 7.5 μL, 0.3 μL (20 pmol) primer 1 and 2, respectively, cDNA 1.0 μL, and water was added to 15 μL. The reaction conditions included pre-denaturation at 94°C for 5 min, 1 cycle; denaturation at 92°C for 15 s; anneal at 60°C and extension for 1 min, a total of 40 cycles; final extension 10 min at 72°C. The primers sequences (synthesized by Shanghai Biotechnology Co., Shanghai, China) are shown in . Glyceraldehyde-3-phosphate dehydrogenase (GAPDH) is one of the most commonly used housekeeping genes for normalizing real-time PCR data because its expression is usually not affected by different conditions.Citation14 It has been shown that GAPDH is a better selection for the comparisons of gene of peritoneal mesothelium cells.Citation15 Our data suggest that GAPDH mRNA levels are constant among different conditions that we used in the study. Therefore, we selected GAPDH as a housekeeping genes in our studies.
TABLE 1. Primer sequences for real-time PCR analysis of Ang II/MAPK-pathways
Western blot analysis
Routine RIPA + PMSF were used to extract total protein and the BCA protein assay method was used to determine the concentration of the total protein. Equal amounts of proteins were mixed with equal volume of reducing sodium dodecyl sulfate (SDS) sample buffer and boiled for 5 min at 95°C. Protein samples were resolved on a 12% SDS-polyacrylamide gel electrophoresis (PAGE) and then electroblotted on nitrocellulose membranes (Bio-Rad, Hercules, CA, USA). After electroblotting, nonspecific binding was blocked with 5% nonfat milk solution. The membrane then was incubated with TGF-β1 antibodies (work concentration: 1 : 200) (Santa Cruz, CA, USA) overnight at 4°C followed by incubation with a horseradish peroxidase-conjugated secondary antibodies for 1 h at room temperature. Exposure, developing, and fixing on X-ray films were obtained after incubation with ECL chemiluminescent reagent. Finally, the results were detected by computer-aided gel image analysis system.
ELISA
Total TGF-β1 in the cell culture supernatant was measured by specific ELISA (R&D Systems, Minneapolis, Minnesota, USA) of conditioned cell culture supernatant samples. TGF-β1 concentration was normalized by the cell number, which was determined by counting cells with a hemocytometer. Data were expressed as nanomole TGF-β1/L per 105 cells.
Measurements of FN, TIMP-1, and PAI-1
Real-time PCR was used to evaluate the mRNA expression of FN, tissue inhibitor of metalloproteinase-1 (TIMP-1), and plasminogen activator inhibitor-1 (PAI-1) undergoing stimulation with Ang II of different concentrations as described previously. The procedure of real-time PCR is the same as above. The sequence of primers is shown in . Western blot analysis was used to measure the expressions of FN in RPMCs using rabbit anti-rat FN antibody (Gibco, CA, USA) (work concentration: 1 : 200).
Assay of ERK1/2, p38 MAPK, and JUN signal pathway
RPMCs (1 × 105) were placed into a 6-well plate. When 70–80% of cells confluence was reached, the medium was changed to serum-free DMEM/F12 for 24 h to achieve growth synchronization. These cells were divided into six groups: N (untreated), A (Ang II 1000 nmol/L), AL (Ang II 1000 nmol/L + Losartan 10-5 mol/L), APD (Ang II 1000 nmol/L + PD98059 20 μmol/L), ASB (Ang II 1000 nmol/L + SB203580 20 μmol/L), and ASP (Ang II 1000 nmol/L + SP600125 20 μmol/L). Cells were pretreated with different inhibitors for 1 h and then stimulated with Ang II for 24 h. Then, cells lysates were obtained for Western blot analysis of extracellular signal-regulated protein kinase (ERK1/2), p38 mitogen-activated protein kinase (MAPK), and SAPK/ c-Jun NH2-terminal kinase (JNK) phosphorylation using rabbit anti-rat Total-ERK1/2 (Santa Cruz) and total-p38 MAPK antibodies (Santa Cruz) (work concentration: 1:200), mouse anti-rat phospho-ERK1/2 (Santa Cruz) and phospho-p38 MAPK (Santa Cruz, USA) (work concentration: 1:200), rabbit anti-rat total-JNK antibody (Cell Signaling, CA, USA), and mouse anti-rat phospho-JNK antibody (Cell Signaling) (work concentration: 1 : 800).
Statistical analysis
Statistical analysis was performed with SPSS 11.0 software package. Enumeration data were expressed in percentage (%) and comparison of ratios was tested by χ2 test. Measurement data were expressed in mean ± standard deviation (x ± s) and comparison of multiple data was made by one-way analysis of variance (ANOVA). Least significant difference and Student–Newman–Keuls test were selected to pairwise comparisons for intergroup means. As for irregular variance, Tamhane's T2 test was filled. A value of p < 0.05 was considered as statistical significance.
RESULTS
Cell culture
A primary culture model for RPMCs was established using enzymatic digestion of rat colic omentum. Under a phase contrast microscope, RPMCs exhibited a typical cobblestone pattern (A) after 6–8 days. We confirmed the presence of rich microvilli on the surface of cells and the existence of rich endoplasmic reticulum and mitochondria using fluoroscopic electron microscopy (B).We also established the phenotype using immunofluorescent analysis for keratin (C) and vimentin (D), and factor VIII was used as a negative control (data not shown).
FIGURE 1. Representative microscopy images of rat peritoneal mesothelial cells (RPMCs). (A) Typical cobblestone pattern in RPMCs at ×200 magnification; (B) typical microvilli on RPMC at ×4200 magnification; (C) immunofluorescent stain for keratin at ×400; and (D) immunofluorescent stain for vimentin at ×400. The representative pictures are shown here.
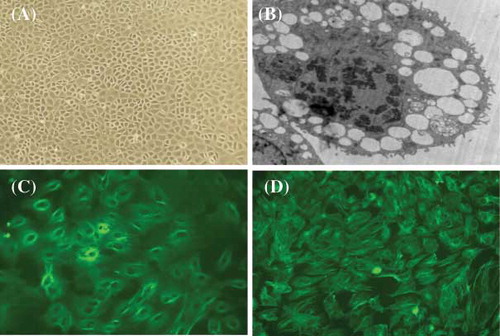
Effect of Ang II on TGF-β mRNA and protein expression
We found that both mRNA and protein levels increased in a dose-dependent manner after the stimulation of cells with different concentration of Ang II for 24 h (A). Both mRNA and protein expression of TGF-β1 reached its peak when cells were stimulated with 100 nmol/L of Ang II, which was 2–3-fold increase compared to the control group (p < 0.05). Similarly, Ang II also stimulated TGF-β1 secretion but peaked at a lower concentration (1 nmol/L) with a 2.14-fold increase as compared to the control (p < 0.01) (B). Ang II also stimulated TGF-β1 secretion in a time-dependent manner and peaked at 24 h (C).
FIGURE 2. Time- and dose-dependent upregulation of TGF-β1 in RPMCs after exposure to Ang II. (A) Concentration-dependent increase of TGF-β mRNA and protein levels following RPMC incubation for 24 h with various concentrations of Ang II. *p < 0.05 compared with Ang II absent group. (B) Concentration-dependent increase of TGF-β secretion following RPMC incubation for 24 h with various concentrations of Ang II and measured using ELISA. *p < 0.05 compared with Ang II absent group; #p < 0.01 compared with Ang II absent group. (C) Time-dependent increase of TGF-β secretion following RPMC incubation with 1 nmol/L of Ang II for various time intervals and measured by ELISA. *p < 0.05 compared with 0 h group; #p < 0.01 compared with 0 h group. Reported p-values indicate significant differences versus control group (only vehicle and no incubation time). Immunoblots are the representative pictures of three independent experiments.
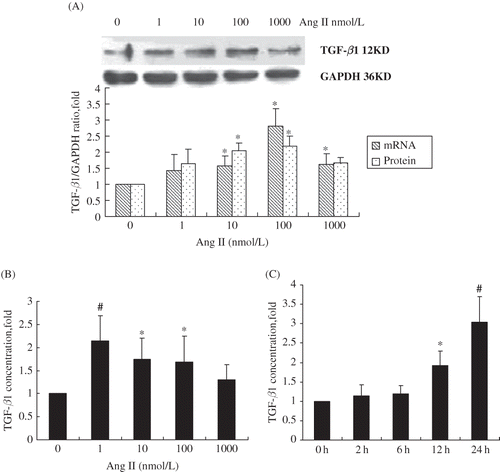
Concentration-dependent effect of Ang II on TIMP-1, PAI-1, FN mRNA, and protein expression
Ang II-stimulated FN mRNA and protein levels in a dose-dependent manner after 24 h stimulation (A). mRNA levels for both TIMP and PAI-1 were also significantly increased in a dose-dependent manner after cells were stimulated by Ang II at different concentrations for 24 h (B and C).
FIGURE 3. Dose-dependent increase in (A) fibronectin, (B) TIMP, and (C) PAI-1 mRNA and protein levels in RPMCs after incubation with various concentrations of Ang II for 24 h. (A) Dose-dependent increase of fibronectin mRNA and protein levels in RPMC after incubation with various concentrations of Ang II for 24 h. (B) Dose-dependent increase of TIMP mRNA levels in RPMC after incubation with various concentrations of Ang II for 24 h. (C) Dose-dependent increase of PAI-1mRNA levels in RPMC after incubation with various concentrations of Ang II for 24 h. Immunoblots are representative pictures of three independent experiments. *p < 0.05 compared with Ang II absent group.
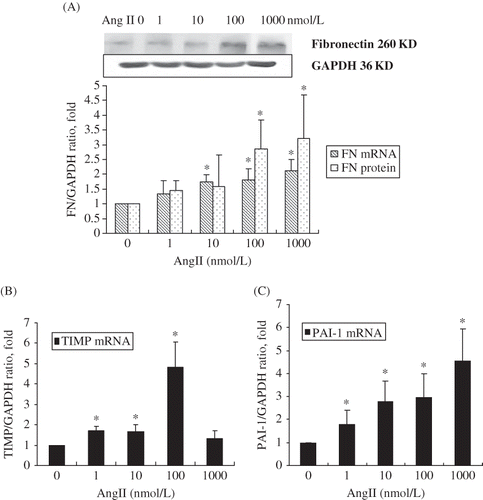
Activation of MAPK pathways in RPMCs
A baseline phospho-ERK1/2 and phospho-p38 MAPK were found in control cells. However, Ang II (1 μmol/L) significantly stimulated phosphorylation of ERK1/2 and p38 MAPK after 1 h incubation while the expressions of total-ERK1/2, total-p38 MAPK, phospho-JNK, and total-JNK remained unchanged (). The densitometric analysis of these immunoblotting data is summarized in the lower panel of . All these experiments were repeated at least thrice. As shown in , phospho-ERK1/2 was greatly attenuated (p < 0.05) by Losartan or PD98059 as expected (A). SB203580 attenuated the phosphorylation of p38 MAPK while Losartan had no effect on it (B). SP600125 attenuated the phosphorylation of JNK significantly while Losartan had no effect on it (D). Furthermore, Ang II at 1000 nmol/L stimulated the phosphorylation of ERK1/2 in a time-dependent manner and peaked at 10 min, while the total ERK1/2 remained unchanged (C).
FIGURE 4. Dose-dependent upregulation of ERK/p38/JNK phosphorylation in RPMCs stimulated with Ang II in the absence or presence of Ang II antagonists. (A) ERK1/2 phosphorylation rose after 60 min stimulation with 1 μmol/L of Ang II, but was inhibited by the addition of either the antagonists losartan (10-5 mol/L; AL) or PD98059 (20 μmol/L; APD). (B) P38 phosphorylation rose after 60 min stimulation with 1 μmol/L of Ang II (A), but was inhibited by the addition of SB203580 (20 μmol/L; ASB). (C) ERK1/2 phosphorylation rose time-dependently following incubation with 1 μmol/L of Ang II and peaked at 10 min, while total ERK1/2 remained unchanged. (D) JNK phosphorylation did not change after 60 min stimulation with 1 μmol/L of Ang II (A), but baseline phosphorylation was inhibited by the addition of SP600125 (20 μmol/L; ASP). All above immunoblots are representative pictures of at least three independent experiments. *p < 0.05 compared with N group(A,B,D) or 0 min group(C); #p < 0.05 compared with A group.
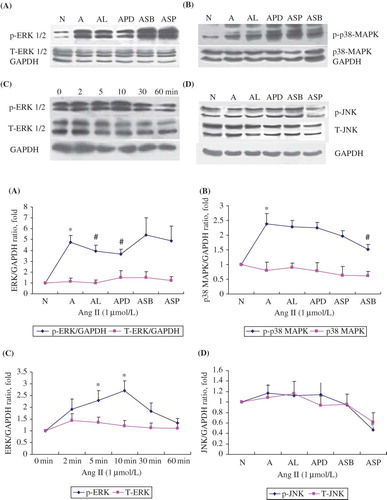
Effect of Ang II and MAPK inhibitors on fibrotic phenotype
Ang II (1000 nmol/L)-induced FN mRNA and protein expression in RPMCs were significantly suppressed by Ang II type-1 (AT1) blocker (Losartan: 105 mol/L) and ERK1/2 pathway inhibitor (PD98059: 20 μmol/L). However, p38 MAPK inhibitor (SB203580) and JNK inhibitor (SP600125) had no effects (). These data suggest that Ang II stimulates FN expression through ERK1/2 pathway.
FIGURE 5. Fibronectin mRNA and protein rose after 60 min stimulation with 1 μmol/L of Ang II (A), but was inhibited by the addition of the AT1 antagonists losartan (10−5 mol/L; AL), the ERK antagonist PD98059 (20 μmol/L; APD), but not with the p38 MAPK inhibitor SB203580 (20 μmol/L; ASB) or the JNK inhibitor SP600125 (20 μmol/L; ASP). Immunoblots are representative pictures of three independent experiments. *p < 0.05 compared with N group; #p < 0.05 compared with A group.
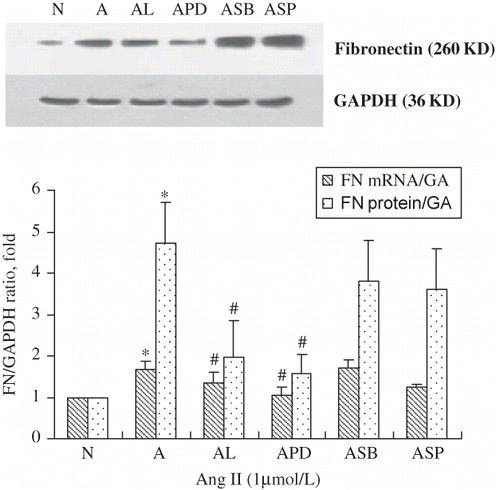
DISCUSSION
Peritoneal fibrosis is a major complication of long-term peritoneal dialyses and ultrafiltration failure is an important reason for patients undergoing continued ambulatory peritoneal dialyses to quit treatment. For this reason, relationship between local RAS and peritoneal fibrosis has become a hot topic in recent studies. Studies showed that chronic activation of local RAS plays an important role in fibrogenesis of organs. Ang II was detected in peritoneal effluent of patients undergoing PD, which was 30 times higher when peritonitis was present than normal.Citation16 AT1 are expressed in human peritoneal mesothelial cells (HPMCs) as well as AT2. Ang II augments the production and secretion of ECM in HPMCs through AT1 receptor. NohCitation17 found that HPMCs express AT1 and synthesize Ang II. HPMC increasing ECM secretion was related to high glucose. All these findings suggest that local RAS exists in the abdominal cavity. Ang II is a type of vasoactive peptide and one of the main effectors of RAS. As a cell factor, Ang II takes part in a series of histopathological processes such as inflammation, proliferation, fibrosis, and so on.Citation18 In this study, we try to elucidate the role of Ang II in peritoneal fibrosis by causing RPMCs injuries. Studies indicate that Ang II is a potential contributor leading to peritoneal fibrosis.
TGF-β1 is an important growth factor involved in ECM modulation and can increase ECM protein syntheses and decrease their degradation. MargettsCitation6 established a peritoneal fibrosis model by adenovirus-mediated gene transfer of active TGF-β1 to rat peritoneum. In this study, we found that Ang II augmented the expression of TGF-β1 mRNA and protein levels in RPMCs in a dose-dependent manner. Interestingly, our results showed that at 1 nmol/L Ang II stimulated TGF-β1 secretion, however, Ang II at the same concentration did not induce TGF-β1 mRNA levels. Our explanation of this fact is that Ang II may stimulate secretion of TGF-β1 at lower dose. In addition, the sensitivity of ELISA may be different from the real-time PCR. Similarly, Kyuden et al.Citation19 reported that Ang II stimulates mesothelial cells to synthesize and secrete TGF-β1. Such results indicate that a large amount of Ang II generated by the activation of RAS during PD has the potential to increase the synthesis and secretion of TGF-β1 in mesothelial cells, leading to fibrogenesis.
As a major component of ECM, FN is capable of binding with fibrin, fibrinogen, and collagen. After binding with integrin, FN plays an important role in mediating cell proliferation and organ sclerosis. An increase in FN synthesis is in parallel with an increase in ECM synthesis.Citation20,Citation21 PAI-1 is a major inhibitor of plasminogen activators. Plasma plasmin could specifically degrade majority of components of ECM and its activity is regulated by plasminogen activators. Increase in PAI-1 synthesis could inhibit plasmin activation, thus reducing ECM degradation.Citation22 As a kind of glycosidoproteins, TIMP binds with all activated matrix metalloproteinases (MMPs) to form a stable complex and inhibits the activity of it.Citation23 ECM degradation is inhibited by MMP by this way. Our study shows that stimulation of cells by Ang II at different concentrations for 24 h increased the expression of FN, TIMP, and PAI-1 significantly in a dose-dependent manner as well. The expression of mRNA for FN and PAI-1 is 1.78 (p < 0.05) and 4.57 (p < 0.05) times, respectively, higher than that in the control group, which is consistent with what MartinCitation24 has reported. It is shown that Ang II increases the production of EMC in mesothelial cells and modulates collagen metabolism-related regulatory factors in downstream such as TIMPS and PAI-1 thus to attenuate the degradation of ECM when local RAS is activated. Ang II also breaks the dynamic equilibrium between the production and degradation of ECM and causes accumulation of ECM. There are four types of TIMP and only one is involved in this study. The other three types will be studied in further research.
Ang II activates many intracellular signal protein through AT1 and AT2 that include PKC, PKA, MAPK, PI3K, and tyrosine kinase such as Src, Pyk2, and p130.Citation25,Citation26 MAPK, a Ser/Thr kinase, is an important mediator in signal transduction pathways, which plays an essential role in cell growth and proliferation, the downstream factors of which have extensive cross-talk effect. MAPK in mammalian cell includes ERK, p38 MAPK, and JNK, which are involved in biological effect in mesothelial cells. d-Glucose increases the synthesis of prostaglandin E2 in HPMC and this effect requires activation of PKC and ERK1/2.Citation27 XuCitation28 indicated that p38 MAPK activity was increased in HPMCs exposed to high glucose and the expression of mRNA for FN was also increased. TGF-β1 specifically enhanced PAI-1 upregulation at the mRNA and protein levels in mesothelial cells in a time- and concentration-dependent manner, mainly through ERK1/2 and p38 MAPK-dependent activation mechanism.Citation29
PerlmanCitation30 reported that Ang II increased the expression of phospho-ERK1/2, which was blocked by AT1 receptor blocker Losartan. ERK1/2, p38 MAPK, and JNK signal pathways were activated by Ang II and were inhibited by the blockers of ERK1/2 and JNK pathways.Citation31 Our work demonstrates that after stimulation by 1000 nmol/L Ang II for 1 h, expressions of phospho-ERK1/2 and phospho-p38 MAPK are increased but that of total-ERK1/2, total-p38 MAPK, phospho-JNK, and total-JNK remain unchanged, which suggests that Ang II can induce the activation of ERK1/2 and p38 MAPK signal pathway but has no effect on JNK. Ang II-induced activation of ERK1/2 was inhibited by Losartan and PD98059. SB203580, SP600125 but not Losartan inhibited Ang II-induced activation of MAPK and JNK, which was consistent with what KiribayashiCitation16 and CanoeCitation32 have reported.
It was shown in Fischer's reportCitation33 that Ang II induced the expression of FN mRNA and this effect can be blocked by Losartan. Uchiyama-TanakaCitation34 found that Ang II induced the expression of FN mRNA through the activation of ERK1/2. We also found that it was ERK1/2 blocker (PD98059) and ARB (Losartan) but not p38 MAPK inhibitor (SB203580) and JNK inhibitor (SP600125) that inhibit Ang II-induced expression of FN mRNA, which was not in parallel with KiribayashiCitation16 report. The reason may lie in the fact that the signal pathway of Ang II-induced expression of FN in human mesothelial cells and that in rat is not the same.
A number of weaknesses of this study should be acknowledged. First, we used a rat cell line to study fibrotic pathways, and the obtained results thus obviously need confirmation in humans. In addition, while we used both negative controls and several inhibitors, we cannot exclude that other pathways than those studied are also activated by Ang II and contribute to the observed effects.
In conclusion, results from our study show that Ang II increases the expression of TGF-β1 and FN and upregulates the expression of TIPM and PAI-1 in RPMCs. Losartan and PD98059 suppress Ang II-induced expression of FN in RPMCs, indicating that AT1 receptor and ERK1/2 pathway are involved in Ang II-induced FN expression. These data suggest that blocking these pathways may provide a potential new therapy to prevent or treat PD-related peritoneal fibrosis.
Acknowledgment
The authors wish to thank Wu Kaiyin, He Cijiang, and Yang Jiajin for their suggestions to the study. This work was supported by grants from the Leading Academic Discipline Project of Shanghai Health Bureau (05III 001 and 2003ZD002) and Shanghai Leading Academic Discipline Project (T0201). Xie Jingyuan is supported by the ISN COMGAN Fellowship.
Declaration of interest: The authors report no conflicts of interest. The authors alone are responsible for the content and writing of this paper.
REFERENCES
- Nakamoto H, Kawaguchi Y, Suzuki H. Encapsulating peritoneal sclerosis in patients undergoing continuous ambulatory peritoneal dialysis in Japan. Adv Perit Dial. 2002;18:119–123.
- Shostak A, Pivnik K, Gotloib L. Daily short exposure of cultured mesothelial cells to lactated, high-glucose, low-pH peritoneal dialysis fluid induces a low-profile regenerative steady state. Nephrol Dial Transplant. 1996;11:608–613.
- Stojimirovic B, Trpinac D, Obradovic M, Milutinovic D, Obradovic D, Nesic V. Changes in peritoneal mesothelial cells in patients on peritoneal dialysis. Med Pregl. 2001;54:219–223.
- Ha H, Cha MK, Choi HN, Lee HB. Effects of peritoneal dialysis solutions on the secretion of growth factors and extracellular matrix proteins by human peritoneal mesothelial cells. Perit Dial Int. 2002;22:171–177.
- Dobbie JW. Pathogenesis of peritoneal fibrosing syndromes (sclerosing peritonitis) in peritoneal dialysis. Perit Dial Int. 1992;12:14–27.
- Margetts PJ, Kolb M, Galt T, Hoff CM, Shockley TR, Gauldie J. Gene transfer of transforming growth factor-beta1 to the rat peritoneum: Effects on membrane function. J Am Soc Nephrol. 2001;12:2029–2039.
- Wolf G, Neilson EG. Angiotensin II as a renal growth factor. J Am Soc Nephrol. 1993;3:1531–1540.
- Brown NJ, Vaughan DE, Fogo AB. The renin-angiotensin-aldosterone system and fibrinolysis in progressive renal disease. Semin Nephrol. 2002;22:399–406.
- Ruiz-Ortega M, Ruperez M, Esteban V, Egido J. Molecular mechanisms of angiotensin II-induced vascular injury. Curr Hypertens Rep. 2003;5:73–79.
- Duman S, Sen S, Duman C, Oreopoulos DG. Effect of valsartan versus lisinopril on peritoneal sclerosis in rats. Int J Artif Organs. 2005;28:156–163.
- Yao Q, Ayala ER, Qian JQ, Stenvinkel P, Axelsson J, Lindholm B. A combination of a PPAR-gamma agonist and an angiotensin II receptor blocker attenuates proinflammatory signaling and stimulates expression of Smad7 in human peritoneal mesothelial cells. Clin Nephrol. 2007;68:295–301.
- Ersoy R, Celik A, Yilmaz O, The effects of irbesartan and spironolactone in prevention of peritoneal fibrosis in rats. Perit Dial Int. 2007;27:424–431.
- Stylianou E, Jenner LA, Davies M, Coles GA, Williams JD. Isolation, culture and characterization of human peritoneal mesothelial cells. Kidney Int. 1990;37:1563–1570.
- Thellin O, Zorzi W, Lakaye B, Housekeeping genes as internal standards: Use and limits. J Biotechnol. 1999;75:291–295.
- Barber RD, Harmer DW, Coleman RA, Clark BJ. GAPDH as a housekeeping gene: Analysis of GAPDH mRNA expression in a panel of 72 human tissues. Physiol Genomics. 2005;21:389–395.
- Kiribayashi K, Masaki T, Naito T, Angiotensin II induces fibronectin expression in human peritoneal mesothelial cells via ERK1/2 and p38 MAPK. Kidney Int. 2005;67:1126–1135.
- Noh H, Ha H, Yu MR, Kim YO, Kim JH, Lee HB. Angiotensin II mediates high glucose-induced TGF-beta1 and fibronectin upregulation in HPMC through reactive oxygen species. Perit Dial Int. 2005;25:38–47.
- Mezzano SA, Ruiz-Ortega M, Egido J. Angiotensin II and renal fibrosis. Hypertension. 2001;38:635–638.
- Kyuden Y, Ito T, Masaki T, Yorioka N, Kohno N. TGF-beta1 induced by high glucose is controlled by angiotensin-converting enzyme inhibitor and angiotensin II receptor blocker on cultured human peritoneal mesothelial cells. Perit Dial Int. 2005;25:483–491.
- White ES, Baralle FE, Muro AF. New insights into form and function of fibronectin splice variants. J Pathol. 2008;216:1–14.
- Kadler KE, Hill A, Canty-Laird EG. Collagen fibrillogenesis: Fibronectin, integrins, and minor collagens as organizers and nucleators. Curr Opin Cell Biol. 2008;20:495–501.
- Huang Y, Noble NA. PAI-1 as a target in kidney disease. Curr Drug Targets. 2007;8:1007–1015.
- Wang JC. Importance of plasma matrix metalloproteinases (MMP) and tissue inhibitors of metalloproteinase (TIMP) in development of fibrosis in agnogenic myeloid metaplasia. Leuk Lymphoma. 2005;46:1261–1268.
- Martin J, Yung S, Robson RL, Steadman R, Davies M. Production and regulation of matrix metalloproteinases and their inhibitors by human peritoneal mesothelial cells. Perit Dial Int. 2000;20:524–533.
- Touyz RM, Berry C. Recent advances in angiotensin II signaling. Braz J Med Biol Res. 2002;35:1001–1015.
- Guo DF, Sun YL, Hamet P, Inagami T. The angiotensin II type 1 receptor and receptor-associated proteins. Cell Res. 2001;11:165–180.
- Sitter T, Haslinger B, Mandl S, Fricke H, Held E, Sellmayer A. High glucose increases prostaglandin E2 synthesis in human peritoneal mesothelial cells: Role of hyperosmolarity. J Am Soc Nephrol. 1998;9:2005–2012.
- Xu ZG, Kim KS, Park HC, High glucose activates the p38 MAPK pathway in cultured human peritoneal mesothelial cells. Kidney Int. 2003;63:958–968.
- Hirashima Y, Kobayashi H, Suzuki M, Tanaka Y, Kanayama N, Terao T. Transforming growth factor-beta1 produced by ovarian cancer cell line HRA stimulates attachment and invasion through an up-regulation of plasminogen activator inhibitor type-1 in human peritoneal mesothelial cells. J Biol Chem. 2003;278:26793–26802.
- Perlman A, Lawsin LM, Kolachana P, Saji M, Moore J Jr., Ringel MD. Angiotensin II regulation of TGF-beta in murine mesangial cells involves both PI3 kinase and map kinase. Ann Clin Lab Sci. 2004;34:277–286.
- Liu B, Yu J, Taylor L, Zhou X, Polgar P. Microarray and phosphokinase screenings leading to studies on ERK and JNK regulation of connective tissue growth factor expression by angiotensin II 1a and bradykinin B2 receptors in rat1 fibroblasts. J Cell Biochem. 2006;97:1104–1120.
- Cano E, Mahadevan LC. Parallel signal processing among mammalian MAPKs. Trends Biochem Sci. 1995;20:117–122.
- Fischer JW, Stoll M, Hahn AW, Unger T. Differential regulation of thrombospondin-1 and fibronectin by angiotensin II receptor subtypes in cultured endothelial cells. Cardiovasc Res. 2001;51:784–791.
- Uchiyama-Tanaka Y, Matsubara H, Nozawa Y, Angiotensin II signaling and HB-EGF shedding via metalloproteinase in glomerular mesangial cells. Kidney Int. 2001;60:2153–2163.