Abstract
Objective: Increased central arterial stiffening is the consequence of many disease states such as diabetes, atherosclerosis, and chronic renal disease. Symmetrical Ambulatory Arterial Stiffness Index (Sym-AASI) may provide a simple clinical approach to evaluate arterial stiffness. This study has tried to evaluate the relationship of Sym-AASI with cystatin C levels. Design and methods: The sample subjects were 53 males and 34 females (mean age = 59.3 ± 13.5 years). Kidney function was evaluated by measuring serum cystatin C and estimated glomerular filtration rate (eGFR). The ambulatory BP was measured noninvasively for 24 h. Results: Patients in the highest quartile showed an older age ( p < 0.001) and worse eGFR (p < 0.001). Pulse pressure (PP) increased as cystatin C was higher. Mean Sym-AASI showed an increase from the first to the last cystatin C quartile. Correlation test showed a significant relationship of Sym-AASI with age (r = 0.573), serum creatinine (r = 0.237), eGFR (–0.323), cystatin C (r = 0.427), systolic blood pressure (r = 0.525), and PP (r = 0.647). Multivariate regression analysis showed that age, cystatin C, nocturnal systolic blood pressure reduction, and nocturnal diastolic blood pressure fall were independently related to Sym-AASI. There was not any independent association between eGFR and Sym-AASI or between cystatin C and PP. Conclusions: Increased Sym-AASI seems to be independently associated with serum cystatin C levels. Sym-AASI seems to be better than PP to detect changes in the arterial wall. This could be a simple and easy method to evaluate arterial stiffness in hypertensive patients without needing more complex devices.
INTRODUCTION
The European Hypertension Society-European Cardiology Society guidelines for the management of arterial hypertension have included arterial stiffness among the indexes of subclinical organ damage able to influence prognosis of hypertensive patients.Citation1 Increased central arterial stiffening is a hallmark of the aging process and the consequence of many disease states such as diabetes, atherosclerosis, and chronic renal compromise. Arterial stiffening is also a marker for increased cardiovascular risk, including myocardial infarction, heart failure, stroke, dementia, renal disease, and total mortality.Citation2,Citation3
A number of direct and indirect methods of measuring arterial stiffness have previously been described but, so far, they are not available in most medical centers, even in those that are specialized in hypertension. The ambulatory arterial stiffness index (AASI) has been proposed as a novel measure of arterial stiffness and has been shown to predict stroke and cardiovascular death in a number of independent studies.Citation4–8 Recently it has been suggested that Symmetrical AASI (Sym-AASI) may provide a better clinical approach to evaluate arterial stiffness than the previously proposed AASI parameter and, most important, may enhance its predictive power.Citation9
On the other hand, high blood pressure is a well-known risk factor for the progression of renal disease and chronic kidney disease (CKD) to end-stage renal disease. As creatinine-based measures seems to be low sensitive markers of renal disease in persons with glomerular filtration rates (GFR) ≤60 mL/min,Citation10 cystatin C has been recommended to evaluate renal function.Citation11 Cystatin C appears to be more sensitive for detecting reduced GFR than either creatinine or estimated GFR.Citation12 Although both creatinine and cystatin C are freely filtered at the glomerulus, a major difference between them is that creatinine is secreted by renal tubules, whereas cystatin C is metabolized by the proximal tubule and only a small fraction appears in the urine.Citation13 Cystatin C has also been shown to be a stronger predictor of adverse outcomes than serum creatinine, so that it could be used as a cardiovascular risk marker.Citation14,Citation15
The aim of this study was to evaluate the relationship of Sym-AASI with a high specific marker of renal function, cystatin C. The results have been compared with estimated glomerular filtration rate (eGFR), obtained from serum creatinine concentration.
DESIGN AND METHODS
Eighty seven patients were studied: 53 males and 34 females; mean age was 59.3 ± 13.5 years; 76% were receiving antihypertensive drug treatment. Kidney function was evaluated by measuring serum cystatin C using a BNII nephelometer (Dade Behring Inc., Deerfield, IL, USA) that used a particle-enhanced immunonephelometric assay (N Latex Cystatin-C). The assay range is 0.195–7.330 mg/L, with the reference range for young healthy individuals reported as 0.53–0.95 mg/L. Microalbuminuria was measured in 24 h urine collection and 18.5% showed increased urine albumin excretion (≥30 mg/day). Highest microalbuminuria was 270 mg/day. Creatinine clearance was calculated by 24 h urine collection. GFR was estimated from serum creatinine using the abbreviated Modification of Diet in Renal Disease equation for every sex.Citation16,Citation17 Only Caucasian patients were included in the study, so that race was not included in calculation. Patients were classified according to K/DOQI stages of chronic renal diseaseCitation18: 16.1% were in stage III, 44.8% were in stage II, and the remaining patients had GFR higher than 90 mL/min (39.1%). The cut-off point for the highest quartile of serum cystatin C distribution was 1.03 mg/L. Causes for stages II and III renal failure were nephroangiosclerosis and aging-related renal function decrease. GFR was estimated from cystatin C using the Hoek formulation.Citation19
The ambulatory BP was measured noninvasively for 24 h by the Spacelab 90207–90217 devices (Redmond, WA, USA) programmed to measure BP every 15 min during daytime and every 20 min during nighttime. The definition of daytime and nighttime was made on the basis of wakefulness and sleep or bed rest periods, obtained from a diary kept by each subject. On the day of ambulatory monitoring, the subjects followed their usual activity, which implied a wide variety of social and manual activity levels, but only if compatible. The arm cuff was positioned on the nondominant upper limb. The subjects were asked to refrain from movement and to keep their upper limb immobile during each measurement. Mean number of measures was 66. Records containing less than 50 measures were excluded from evaluation. In every subject 24 h ambulatory BP mean, daytime BP mean, and nighttime BP mean were calculated.
Results are expressed as mean ± 1 standard deviation. All statistical tests were two sided. p-Values lower than 0.05 were considered as significant. For comparisons between groups, Student's t-test was used for continuous variables and chi-square test for categorical variables. Correlations were tested using the Pearson coefficient correlation test. Afterwards multivariate regression analysis was performed to confirm the independent predictive power of renal function. Using multivariable linear regression, we studied the association between kidney function (using either eGFR or serum cystatin C concentration) and Sym-AASI. This analysis was performed twice using eGFR and cystatin C separately because both measures are closely related and might interfere if they were introduced together. In these analyses, each measure was evaluated as a continuous variable. We controlled for secondary variables of interest [age, sex, body mass index, waist circumference, total cholesterol, low-density lipoprotein cholesterol, high-density lipoprotein cholesterol, triglycerides, systolic blood pressure (SBP) night fall, diastolic blood pressure (DBP) night fall, and antihypertensive treatment]. A two-tailed p-value ≥0.05 was considered significant.
All statistical tests were performed with the statistical package PAZW 17.0. This program was also used to calculate the systolic-on-diastolic slope obtained by standard linear regression from SBP plotted versus DBP as well as the diastolic-on-systolic slope obtained by standard linear regression from DBP plotted versus SBP. From these values we estimated AASI and Sym-AASI using the formulations published by Gavish et al.Citation9:
RESULTS
Baseline demographic, clinical, procedural, and laboratory findings of population divided according to the cystatin C quartiles are listed in . As expected the patients in the highest quartile of cystatin C distribution showed an older age (p < 0.001) and worse parameters of renal function (eGFR, p < 0.001). Pulse pressure (PP) increased as cystatin C was higher. SBP and DBP increased for every quartile of cystatin C, but they were lower in the highest quartile. Contrariwise, mean Sym-AASI (and also AASI) showed a steady state increase from the first to the last cystatin C quartile (see ).
Table 1. Baseline demographic, clinical, procedural, and laboratory findings of population divided according to the cystatin C quartiles
Figure 1. AASI and Sym-AASI increases as cystatin C increases (plotted by quartiles). Differences are significant (see values in ).
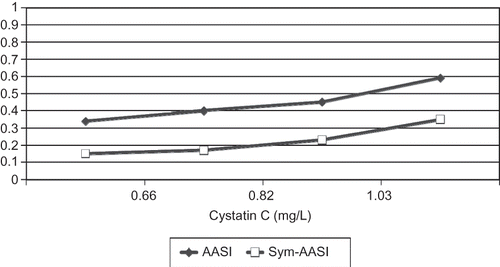
Correlation test showed a significant relationship of Sym-AASI with age (r = 0.573, p < 0.001), serum creatinine (r = 0.237, p = 0.027), eGFR (–0.323, p = 0.002), cystatin C (r = 0.427, p < 0.001), SBP (r = 0.525, p < 0.001), and PP (r = 0.647, p < 0.001). DBP was not correlated with Sym-AASI. Neither waist circumference nor body mass index showed any correlation.
Multivariate regression analysis showed that age (p < 0.001), cystatin C (p = 0.044), nocturnal SBP reduction (p = 0.045), nocturnal DBP fall (p = 0.001) and waist perimeter (p = 0.0045) were independently related to Sym-AASI (see ). A second multivariate regression analysis was performed including eGFR instead of serum cystatin C concentration, but there was not any independent association between eGFR and Sym-AASI (results are shown in ).
Table 2. Multivariate linear regression: Sym-AASI versus cystatin C
Table 3. Multivariate linear regression: Sym-AASI versus eGFR
As PP is regarded as a surrogate of arterial stiffness, a new multivariate regression analysis was performed looking for the relationships with this parameter [mean 24 h PP measured by ambulatory blood pressure monitoring (ABPM)]. This analysis was also made twice including one-time cystatin C levels and, afterwards, eGFR. No independent association was found for anyone of those latter parameters. Results are shown in .
Table 4. Multivariate linear regression: PP versus cystatin C
DISCUSSION
The main finding of this study is that Sym-AASI, a surrogate of arterial stiffness measured through 24 h ABPM, has a good relationship with cystatin C, a cardiovascular risk marker. This independent relationship was confirmed by multivariate regression analysis. Contrariwise, no independent correlation was found between PP, another commonly used arterial stiffness surrogate, and cystatin C.
The AASI has been proposed as a readily available alternative to more costly and specialized measures of arterial stiffness such as pulse wave velocity or augmentation index.Citation20 The use of Sym-AASI was proposed by Gavish et al.Citation9,Citation21 who suggested that neither the slope nor the AASI can be estimated correctly using standard regression, and that the use of standard regression applied in the determination of the AASI may lead to overestimation of arterial stiffness and mask its dependence on baseline characteristics, reflecting its artifactual dependence on r-value as well as on dipping. In this way, it has been reported that nondipping strongly affects the AASI,Citation22 so that such dependence may limit its prognostic predictive value. This study has used Sym-AASI as the main measure of arterial stiffness but AASI was also calculated for all cystatin C quartiles and only small differences have been found between these parameters as arterial stiffness markers, so that we cannot find advantages in the clinical ground for using one measure or another.
AASI has been associated with an increased risk of cardiovascular mortsality in hypertensive patientsCitation6 and stroke in the general populationCitation7, as well as an increased AASI has been found in metabolic syndrome patients.Citation23 It has been associated with preclinical renal organ damage in hypertension: patients with microalbuminuria, carotid abnormalities, or left ventricle hypertrophy (LVH) showed higher AASI as compared with those without itCitation24; but the relationship of LVH with AASI was not confirmed in the study of Schillaci et al.Citation22 Moreover, Ratto et al. reported that AASI was positively related to urinary albumin excretion and resistive index and was negatively related to estimated creatinine clearance and renal volume to the resistive index ratio.Citation25 More recently, Mulè et al. found that AASI was inversely related to GFR in a group of 143 untreated hypertensive subjects with serum creatinine <1.5 mg/dL.Citation26 Other authors have found similar correlations with creatinine clearance estimated from serum creatinineCitation24 or with GFR computed from the scintigraphic determination of the technetium-99m diethylenetriaminepentaacetic acid uptake within the kidneys, by the Gates method.Citation25 There is less experience with Sym-AASI but in a recent report Sym-AASI showed a significant relationship with creatinine clearance, serum creatinine, and eGFR estimated using Modification of Diet in Renal Disease-4 equation.Citation27 These correlations were also found in this study but the finding was not confirmed by multivariate regression analysis where only age, waist perimeter, cystatin C, and nighttime blood pressure fall were independently associated to Sym-AASI.
Cystatin C is a strong marker of kidney dysfunction and also a correlate of cardiovascular risk factors. Nevertheless, these two abilities of cystatin C seem not to be correlated in our study. In fact Sym-AASI was independently associated with cystatin C levels but this relationship was not found with eGFR. These findings can be interpreted in various ways. First of all, persons with high cystatin C levels but without CKD may have preclinical CKD and an associated elevated risk factor burden that is similar to persons with CKD. Because of measurement error involved in the quantification of eGFR, it is also possible that persons with high cystatin C but eGFR that is not in the CKD range are more accurately classified as renal patients by using cystatin C levels.Citation28 Alternatively, high cystatin C in persons without CKD may be the result of extra-renal sources of cystatin C variability and they were not related to kidney function. There is some evidence that serum cystatin C level is heritable and high serum cystatin C levels were associated with most major CVD risk factors. These associations persisted with the additional exclusion of persons with CKD or with microalbuminuria.Citation23 The relationship between cystatin C and Sym-AASI confirms the value of using this simple method to evaluate arterial stiffness and warrant wider studies to show a possible predictive effect on cardiovascular morbidity and mortality.
CONCLUSIONS
Some conclusions could be drawn from these results: Increased Sym-AASI – an improved method for detecting arterial stiffness based on ambulatory blood pressure monitoring – seems to be independently associated with serum cystatin C levels. These results confirm previous reports on the usefulness of Sym-AASI for cardiovascular risk assessment because cystatin C is a well-known marker of cardiovascular disease and mortality. In this regard, Sym-AASI seems to be better than PP – a classic surrogate of arterial stiffness – to detect changes in the arterial wall. As the measurement of Sym-AASI needs only the performance of ABPM this could be a simple and easy method to evaluate arterial stiffness in hypertensive patients without needing more complex devices. Wider studies are warranted to establish the correlation of Sym-AASI with other kinds of target organ disease due to hypertension and other cardiovascular risk factors.
ACKNOWLEDGMENT
The authors thank Professor Ian McPartlin for reviewing the English grammar.
Declaration of interest: The authors report no conflicts of interest. The authors alone are responsible for the content and writing of the paper.
REFERENCES
- Mancia G, De Backer G, Dominiczak A, 2007. Guidelines for the management of arterial hypertension: The Task Force for the Management of Arterial Hypertension of the European Society of Hypertension (ESH) and of the European Society of Cardiology (ESC). J Hypertens. 2007;25:1105–1187.
- Zieman SJ, Melenovsky V, Kass DA. Mechanisms, pathophysiology, and therapy of arterial stiffness. Arterioscler Thromb Vasc Biol. 2005;25:932–943.
- Safar ME, Levy BI, Struijker-Boudier H. Current perspectives on arterial stiffness and pulse pressure in hypertension and cardiovascular diseases. Circulation. 2003;107:2864–2869.
- Li Y, Wang JG, Dolan E, Ambulatory arterial stiffness index derived from 24-h ambulatory blood pressure monitoring. Hypertension. 2006;47:359–364.
- Dolan E, Thijs L, Li Y, Ambulatory arterial stiffness index as a predictor of cardiovascular mortality in the Dublin Outcome Study. Hypertension. 2006;47:365–370.
- Hansen TW, Staessen JA, Torp-Pedersen C, Ambulatory arterial stiffness index predicts stroke in a general population. J Hypertens. 2006;24:2247–2253.
- Kikuya M, Staessen JA, Ohkubo T, Ambulatory arterial stiffness index and 24-hour ambulatory pulse pressure as predictors of mortality in Ohasama, Japan. Stroke. 2007;38:1161–1166.
- Ben-Dov IZ, Gavish B, Kark JD, Mekler J, Bursztyn M. A modified ambulatory arterial stiffness index is independently associated with all-cause mortality. J Hum Hypertens. 2008;22:761–766.
- Gavish B, Ben-Dov IZ, Bursztyn M. Linear relationship between systolic and diastolic blood pressure monitored over 24 h: Assessment and correlations. J Hypertens. 2008;26:199–209.
- Rule AD, Larson TS, Bergstralh EJ, Slezak JM, Jacobsen SJ, Cosio FG. Using serum creatinine to estimate glomerular filtration rate: Accuracy in good health and in chronic kidney disease. Ann Intern Med. 2004;141:929–937.
- Fliser D, Ritz E. Serum cystatin C concentration as a marker of renal dysfunction in the elderly. Am J Kidney Dis. 2001;37:79–83.
- Newman DJ, Thakkar H, Edwards RG, Serum cystatin C measured by automated immunoassay: A more sensitive marker of changes in GFR than serum creatinine. Kidney Int. 1995;47:312–318.
- Laterza OF, Price CP, Scott MG. Cystatin C: An improved estimator of glomerular filtration rate? Clin Chem. 2002;48:699–707.
- Shlipak MG, Sarnak MJ, Katz R, Cystatin C and the risk of death and cardiovascular events among elderly persons. N Engl J Med. 2005;352:2049–2060.
- Shlipak MG, Sarnak MJ, Katz R, Cystatin-C and mortality in elderly persons with heart failure. J Am Coll Cardiol. 2005;45:268–271.
- Manjunath G, Sarnak MJ, Levey AS. Prediction equations to estimate glomerular filtration rate: An update. Curr Opin Nephrol Hypertens. 2001;10:785–792.
- Levey A, Bosch J, Lewis JB, Greene T, Rogers N, Roth D. A more accurate method to estimate glomerular filtration rate from serum creatinine: A new prediction equation. Ann Intern Med. 1999;130:461–470.
- National Kidney Foundation. K/DOQI clinical practice guidelines for chronic kidney disease: Evaluation, classification, and stratification. Kidney Disease Outcome Quality Initiative. Am J Kidney Dis. 2002;39(Suppl. 1):S1–S246.
- Hoek FJ, Kemperman FA, Krediet RT. A comparison between cystatin C, plasma creatinine and the Cockcroft and Gault formula for the estimation of glomerular filtration rate. Nephrol Dial Transplant. 2003;18:2024–2031.
- O'Brien E. Ambulatory blood pressure measurement: A trove of hidden gems? Hypertension. 2006;48:364–365.
- Gavish B, Ben-dov IZ, Bursztyn M. Ambulatory arterial stiffness index is not a specific marker of reduced arterial compliance [letter]. Hypertension. 2007;50:18.
- Schillaci G, Parati G, Pirro M, Ambulatory arterial stiffness index is not a specific marker of reduced arterial compliance. Hypertension. 2007;49:986–991.
- Leoncini G, Ratto E, Viazzi F, Metabolic syndrome and ambulatory arterial stiffness index in non-diabetic patients with primary hypertensión. J Hum Hypertens. 2007;21:802–807.
- Leoncini G, Ratto E, Viazzi F, Increased ambulatory arterial stiffness index is associated with target organ damage in primary hypertension. Hypertension. 2006;48:397–403.
- Ratto E, Leoncini G, Viazzi F, Ambulatory arterial stiffness index and renal abnormalities in primary hypertension. J Hypertens. 2006;24:2033–2038.
- Mulè G, Cottone S, Cusimano P, Inverse relationship between ambulatory arterial stiffness index and glomerular filtration rate in arterial hypertensión. Am J Hypertens. 2008;21:35–40.
- Robles NR, Mena C, Macias R, Garcia de Vinuesa E, Herrera J, Macias JF. Symmetrical ambulatory arterial stiffness index: Relationship with microalbuminuria and renal function. Eur J Intern Med. 2010;21:118–122.
- Shlipak MG, Katz R, Sarnak MJ, Cystatin C and prognosis for cardiovascular and kidney outcomes in elderly persons without chronic kidney disease. Ann Intern Med. 2006;145:237–246.