Abstract
Background/aim: Hyperphosphatemia is a well-recognized complication of chronic kidney disease, and phosphorus kinetics during hemodialysis (HD) remains a vague area of investigation. We studied the inorganic phosphorus homeostasis during the first hour of an HD session. Materials/methods: Twelve patients were studied twice, in two consecutive HD sessions. Total (TPR), extracellular (EPR), and intracellular (IPR) phosphorus mass removal was determined using the direct dialysate quantification (DDQ) method. Alterations of serum inorganic phosphorus (sP), erythrocyte intracellular phosphorus (PERY), and 2,3-diphosphoglycerate (2,3-DPG) concentrations were measured before HD initiation and at 1, 2, 3, 4, 5, 10, 30, and 60 min. Results: The contribution of IPR to TPR was negative in the first 10 min of both HD sessions (–27.2 ± 6.5 and −26.4 ± 58 mmol, respectively, p = ns) while the contribution of the IPR to TPR increased as the time elapsed. Intracellular phosphorus and 2,3-DPG remained almost unchanged during the 60 min of HD session. Conclusions: Unchanged PERY concentration during the first hour of an HD session does not reject the hypothesis of a simultaneous efflux and influx of phosphorus from/to intracellular compartment.
INTRODUCTION
Hyperphosphatemia is a well-recognized complication of chronic kidney disease (CKD) which plays a major role in the pathogenesis of secondary hyperparathyroidism.Citation1 Moreover, it has been linked to increased cardiovascular mortality.Citation2 Therefore, achieving normal phosphate serum levels, as outlined by National Kidney Foundation/Disease Outcomes Quality Initiative (NKF/DOQI) guidelines,Citation3 is of paramount importance for the treatment of end-stage CKD patients. Restriction of dietary phosphorus intake, phosphorus binder’s administration, and enhancement of phosphorus removal through hemodialysis (HD) constitute the main current treating procedure for hyperphosphatemia.Citation4,5 A number of previous studies have investigated phosphorus removal during HD.Citation5–8 Phosphorus kinetics is characterized by (1) a rapid decline in serum phosphorus concentration during the first half of the HD session, (2) an almost stabilized concentration during the rest of the HD time in which phosphorus removal continues, and (3) a rebound phenomenon which starts before HD session ends.Citation9–13 These observations suggest that inorganic phosphorus is transferred from intracellular compartment or other phosphorus pools to intravascular compartment at different mass rates. However, data are limited regarding the contribution of intracellular compartment to total inorganic phosphorus mass removal during the early period of an HD session, while the existing data suggest that this contribution is negligible or negative.Citation7,14
The aim of this study was to investigate in depth the inorganic phosphorus homeostasis (intra- and extracellular) during the early period of an HD session through multiple and consecutive measurements of phosphorus indexes.
PATIENTS AND METHODS
Patients
Twelve anuric (urine output <100 mL/day) patients (8 male, 4 female), on chronic HD program (three sessions/week), were included in this study. Median age was 56 (range 25–80) years and their mean dry body weight (wt) was 70.5 ± 13.8 kg. They were on HD for 38 ± 15 (range 28–75) months through an arteriovenous fistula (10 patients) and a synthetic graft (2 patients). Recirculation fraction was <5% as this was calculated by the slow flow method.Citation15 Exclusion criteria were diabetes mellitus, hypoalbuminemia (serum albumin <35 g/L), known uncured malignancy, active infection, history of liver or heart failure (stage IV New York Heart Association (NYHA)), and previous surgery on the gastrointestinal tract. All patients were receiving calcium carbonate as phosphorus binder in a stable dose of 2 g (range 1.5–3.5 g) daily and none of them were taking gastric acid secretion inhibitors. Vitamin D and recombinant erythropoietin dose were unchanged during the study period (0.2 μg and 9000 IU/week, respectively). An informed consent was obtained from all patients.
Study Design
We investigated intra- and extracellular inorganic phosphorus homeostasis during the first hour of an HD session. Each patient was studied twice during two consecutive HD sessions, the first (long interval between HD sessions of 72 h) and midweek (short interval between HD sessions of 44 h) sessions of the week.
Dialysis Procedure
Duration of HD session was 240 min in all patients. Blood flow was 300 mL/min (machine set point), dialysate flow was 500 mL/min, and its composition was Na+ 132 mmol/L, K+ 2.0 mmol/L, Cl− 103 mmol/L, Ca++ 1.75 mmol/L, Mg2+ 0.75 mmol/L, acetate 5 mmol/L, and bicarbonate 35 mmol/L.
Food and fluid intake were restricted during the study period. Ultrafiltration rate during the first hour of the HD session was negligible in order to eliminate possible effect of hemoconcentration on intra- and extracellular inorganic phosphorus concentrations.
Blood/Dialysate Samples
Blood samples were obtained through arterial line before (t0) and at 1, 2, 3, 4, 5, 10, 30, and 60 min from HD initiation. Serum inorganic phosphorus (sP) levels were measured using a photometric method (Olympus AU 600, Tokyo, Japan). Intracellular phosphate was estimated by measuring erythrocyte intracellular phosphorus (PERY) and 2,3-diphosphoglycerate enzyme (2,3-DPG) concentrations. Although erythrocytes do not specifically reflect the intracellular phosphate compartment, they represent a simple and reproducible model for its evaluation. 2,3-DPG is in fact the major phosphorylated intermediate in the erythrocyte, presenting higher concentrations than adenosine triphosphate or inorganic phosphate. PERY and 2,3-DPG were determined by a previously described method.Citation16 Arterial blood gas and serum bicarbonate concentrations were measured before and at 60 min after HD initiation using a blood gas analyzer (Blood gas system 288, ABL 555, Radiometer, Copenhagen, Denmark).
Dialysate samples were taken from all dialysate fluid spent in partial (0–10, 10–30, and 30–60 min) and in total (0–60 min) studied periods. The dialysate effluent of the partial studied periods was collected and acidified in three different water tanks of 5, 10, and 15 L, respectively, which were placed on a calibrated bed scale. Finally, a big water tank of 30 L was used in order to collect the total dialysate volume during the first hour of the HD session. In the above samples, inorganic phosphorus (dP) was measured using a photometric method (Olympus AU 600).
Calculations
Total (TPR), extracellular (EPR), and intracellular (IPR) mass of inorganic phosphorus removed during each sampling period were determined using the following equations:Citation14
where Vd is the total dialysate fluid volume (L), dP the dialysate inorganic phosphorus concentration (mmol/L), and (i) the time over which measurements were made.
where VD is the inorganic phosphorus distribution volume (as 20% of total body water) and Δs(sP) the change in sP concentration (mmol/L).
Statistical Analysis
Results were expressed as mean ± standard deviation. p-Values of less than 0.05 were considered statistically significant. All p-values were two tailed. Analyses between mean values were performed with the use of independent sample t-test to determine the least significant difference in means. Groups with normal distribution were analyzed using Student’s t-test. Wilcoxon test was used for data not normally distributed. The results obtained were analyzed using SPSS 15 (SPSS, Inc., Chicago, IL, USA).
RESULTS
Temporal profiles of sP concentrations during the first hour of the HD session in the first and midweek HD sessions are shown in . Pre-HD sP concentrations were higher in the first than in the midweek HD session, as expected (1.91 ± 0.28 vs. 1.62 ± 0.24 mmol/L, respectively).
Figure 1. Changes in serum phosphorus concentrations over the first hour of the HD sessions (long and short HD intervals) (A) first session; (B) second session.
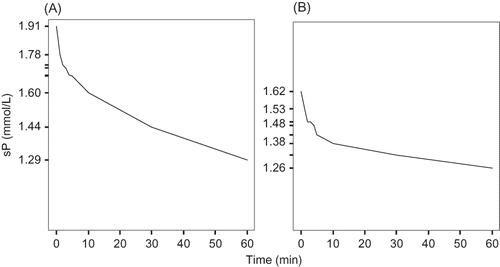
Fluctuations of PERY and 2,3-DPG in the first 10 min, as well as the temporal profiles during the rest 40 min of both HD sessions, are shown in (no statistically significant difference was noted).
Figure 2. Changes in intracellular phosphorus and 2,3-DPG enzyme concentrations during the first hour of the HD sessions (long and short HD intervals) (A) first session; (B) second session.
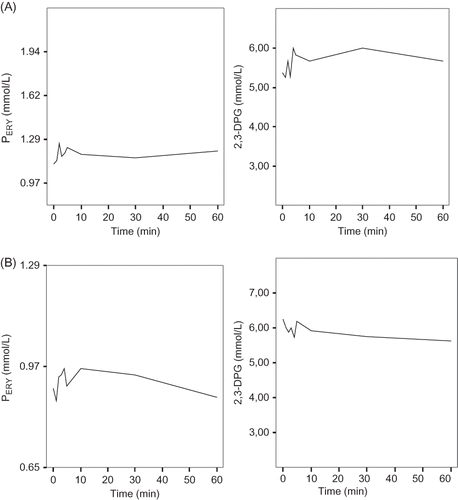
There was no statistically significant difference in TPR between the first and midweek HD sessions (91.2 ± 20.6 vs. 84.2 ± 18.5 mmol, p = ns) (). The contribution of IPR to TPR was negative in the first 10 min of both HD sessions (–27.2 ± 6.5 and −26.4 ± 58 mmol, respectively, p = ns) while the contribution of IPR to TPR increased as time elapsed ().
Figure 3. Total (TPR), extracellular (EPR), and intracellular (IPR) mass of phosphorus removed during the first hour of the HD sessions (long and short HD intervals) (A) first session; (B) second session.
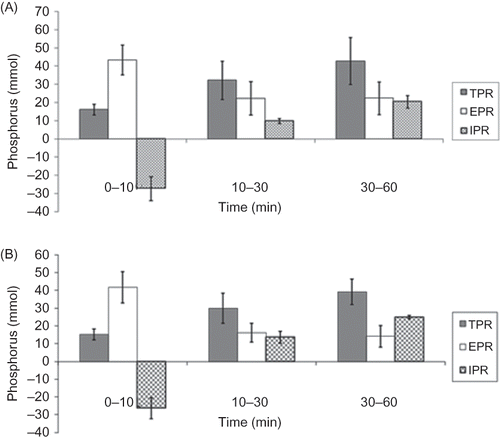
A statistically significant increase in serum bicarbonate concentration was observed 60 min after the HD initiation in both HD sessions [from 21.0 ± 2.1 to 24.0 ± 1.9 mmol/L, p < 0.05 (first HD) and from 22.1 ± 1.8 to 24.8 ± 1.5 mmol/L, p < 0.05 (second HD)].
DISCUSSION
Phosphorus kinetics constitutes a difficult HD research area and it has been stated that no matter how complex the model used to explain phosphate behavior during HD, it would still remain an oversimplification of natural events.Citation14
The results of our study showed a progressive fall in sP concentration during the first hour of the HD session. Moreover, we found a negative contribution of IPR to TPR in the first 10 min of the HD session which is in accordance with the results of two previous studies.Citation7,14 Messa et al.Citation14 showed that during the first 45 min the extracellular phosphate removal was greater than total, which could be explained either by an influx of phosphate into the intracellular space or alternatively by a possible overestimation of the effective distribution volume of phosphate, which during the first 45 min might be lower than that estimated. We chose to investigate alterations in extra- (serum) and intracellular (RBC) inorganic phosphorus concentrations during the first hour of the HD session as detailed data are lacking for this early period of time and it has been shown that this first quadrant of an HD session is critical as more than 30% of the total phosphorus mass removal occurs during it. Moreover, regardless of the duration of HD, a characteristic plateau phase is rapidly reached in the first hour, below which phosphorus levels do not fall further. Through multiple and consecutive measurements during the first 10 min, we found a probable “real” negative contribution of IPR to TPR, as ultrafiltration rate was negligible. Following that, IPR contribution to TPR progressively increased.
Blood could be considered a two-phase medium composed of a homogenous solution, plasma, with the erythrocytes in suspension. It has been shown that the permeability of different solutes across the RBC membrane varies widely.Citation17,18 Thus, during HD session, solutes which equilibrate very rapidly are cleared from both plasma and RBC (i.e., urea), while other solutes which equilibrate slowly are cleared mainly from plasma (i.e., creatinine).Citation17,18
Regarding inorganic phosphorus, the available data are conflicting. A number of investigators have proposed that intracellular and extracellular (serum) phosphorus equilibrate during HD session,Citation19 while others have found no changes in the intracellular RBC inorganic phosphorus during HD.Citation20,21 We found an almost unchanged concentration of intracellular inorganic phosphorus and 2,3-DPG during the first hour of the HD session which indicates that, during the short time of blood transit through the dialyzer, only a negligible amount of inorganic phosphorus is removed from RBC. This is also supported by previous dataCitation19,22 and can satisfactorily explain the clinical observation that the in vivo removal of phosphorus is systematically less than that expected from the in vitro dialyzer clearance values.Citation23
However, another possible explanation for the unchanged intracellular inorganic phosphorus and 2,3-DPG concentration during the first hour of the HD session, in combination with the initial negative contribution of IPR to TPR, could be that the mass of inorganic phosphorus removed from extracellular compartment and calculated as intracellular (RBC) compartment (negative contribution) may be removed from other phosphorus pools during HD process. The complexity of phosphate kinetics mechanisms has been nicely approached by Spalding et al.Citation24 who have proposed an accurate four pools phosphate model.
In vitro studies have shown that efflux of intracellular inorganic phosphorus from RBC is not only slow, but also a quantitatively limited phenomenon.Citation25 Indeed, the net changes in sP concentration did not exceed 9% after 4 h HD.Citation26,27 The latter, in combination with a small decrease in intracellular inorganic phosphorus concentration after a 4 h HD session,Citation23 indicates that during an HD session inorganic phosphorus is transferred from extracellular phosphorus pools to intravascular compartment and after that, through dialyzer membrane, to dialysate fluid.
Despite the fact that inorganic phosphorus is transferred from intra- to extracellular compartment and vice versa or released from other storage inorganic phosphorus pools to intra- and extracellular compartment during an unsteady-state condition, as an HD session, the complexity of inorganic phosphorus global kinetics may be explained by the patient’s body effort to retain sufficient inorganic phosphorus stores (intracellular) in order to use them for energy, enzyme reaction, or cellular pumps activity.
In conclusion, the intracellular inorganic phosphorus and 2,3-DPG enzyme concentrations remained almost unchanged during the first hour of dialysis session. However, this finding does not contradict the simultaneous efflux and influx of inorganic phosphorus from/to intracellular compartment.
ACKNOWLEDGMENT
The authors thank Mrs. Aleka Papageorgiou for the skilled secretarial assistance.
Declaration of interest: The authors report no conflicts of interest. The authors alone are responsible for the content and writing of the paper.
REFERENCES
- Delmez JA, Slatopolsky E. Hyperphosphatemia: Its consequences and treatment in chronic renal failure. Am J Kidney Dis. 1992;19:303–317.
- Eifinger F, Wahn F, Querfeld U, . Coronary artery calcifications in children and young adults treated with renal replacement therapy. Nephrol Dial Transplant. 2000;15:1892–1894.
- National Kidney Foundation. K/DOQI clinical practice guidelines for bone metabolism and disease in chronic kidney disease. Am J Kidney Dis. 2003;42(Suppl. 3):S1–S201.
- Hercz G, Cobum JW. Prevention of phosphate retention and hyperphosphatemia in uremia. Kidney Int. 1987;32(Suppl. 22):S215–S220.
- DeSoi CA, EJmans JG. Phosphate kinetics during high-flux hemodialysis. J Am Soc Nephrol. 1993;4:1214–1218.
- Haas T, Hillion D, Dongradi G. Phosphate kinetics in dialysis patients. Nephrol Dial Transplant. 1991;6(Suppl. 2):S108–S113.
- Fischbach M, Hamel G, Simeoni U, Geisert J. Phosphate dialytic removal: Enhancement of phosphate cellular clearance by biofiltration (with acetate-free buffer dialysate). Nephron. 1992;62:155–160.
- Man NK, Chauveau P, Kuno T, Pognet JL, Yanai M. Phosphate removal during hemodialysis and hemofiltration. ASAIO Trans. 1991;37:M463–M465.
- Cristinelli L, Mioni G, Cecchettin M, . Multicompartmental analysis of the phosphate pool in dialyzed uremic patients. Minerva Nephrol. 1976;23:292–300.
- Hou SH, Zhao J, Ellman CF, . Calcium and phosphorus fluxes during hemodialysis with low calcium dialysate. Am J Kidney Dis. 1991;18:217–224.
- Pogglitsch H, Petek W, Ziak E, Sterz F, Holzer H. Phosphorus kinetics during haemodialysis and haemofiltration. Proc Eur Dial Transplant Assoc Eur Ren Assoc. 1985;21:461–468.
- Sugisaki H, Onohara M, Kunitomo T. Dynamic behavior of plasma phosphate in chronic dialysis patients. Trans Am Soc Artif Intern Organs. 1982;28:302–307.
- Sugisaki H, Onohara M, Kunitomo T. Phosphate in dialysis patients. Trans Am Soc Artif Intern Organs. 1983;29:38–43.
- Messa P, Gropuzzo M, Cleva M, . Behaviour of phosphate removal with different dialysis schedules. Nephrol Dial Transplant. 1998;13(Suppl. 6):S43–S48.
- NKF-K/DOQI. Clinical Practice Guidelines for hemodialysis adequacy. Am J Kidney Dis. 2001;37(Suppl. 1):S9–S61.
- Challa A, Bevington A, Anger CM, Asbury AJ, Preston CJ, Russell RGG. A technique for the measurement of orthophosphate in human erythrocytes and some studies of its determinants. Clin Sci. 1985;69:429–434.
- Descombes E, Perriard F, Fellay G. Diffusion kinetics of urea, creatinine and uric acid in blood during hemodialysis. Clinical implications. Clin Nephrol. 1993;40:286–295.
- Colton CK, Smiths KA, Merrill EW, Reece JM. Diffusion of organic solutes in stagnant plasma and red cell suspensions. Chem Eng Prog Symp Ser. 1970;66:85–100.
- Pogglitsch H, Estelberger W, Petek W, Zitta S, Ziak E. Relationship between generation and plasma concentration of anorganic phosphorus. In vivo studies on dialysis patients and in vitro studies on erythrocytes. Int J Artif Organs. 1989;12:524–532.
- Liano F, Santirso R, Aschcroft R, . Effect of hemodialysis on plasma and red cell inorganic and total phosphate levels (abstract). Proc Eur Dial Transplant Assoc. 1982;19:86.
- Liano F, Santirso R, Aschcroft R, . Compartment of inorganic and total phosphate during hemodialysis (abstract). Proc Eur Dial Transplant Assoc. 1982;19:86.
- Zucchelli OP, Santoro A. Inorganic phosphate removal during different dialytic procedures. Int J Artif Organ. 1987;1:173–178.
- Katopodis KP, Chala A, Koliousi E, . Role of the dialyzer membrane on the overall phosphate kinetics during hemodialysis. Blood Purif. 2005;23:359–364.
- Spalding EM, Chamney PW, Farrington K. Phosphate kinetics during hemodialysis: Evidence for biphasic regulation. Kidney Int. 2002;61:655–667.
- Preston CJ, Challa A, Phillips JE, Foden SE, Douglas DL, Russell RGG. Response of red cell phosphate to acute and chronic changes in plasma phosphate in man. Adv Exp Med Biol. 1980;128:239–248.
- Walter U. Effect of ouabain and furosemide on erythrocyte sodium and phosphate transport. Clin Pharmacol Ther. 1981;30:709–717.
- Walter U, Becht E. Red blood cell sodium transport and phosphate release in uremia. Nephron. 1993;34:35–41.