Abstract
Blood flow to several tissues changes during an acute bout of exercise. The kidney is one of the organs that are most affected by exercise-induced blood redistribution. The aim of the present study was to investigate possible exercise-induced vascular reactivity changes in renal resistance arteries in rats. Renal resistance arteries were isolated from rats that underwent 8 weeks of swimming and sedentary control rats, and the arteries were evaluated using wire myography. Similar dilation responses to acetylcholine, bradykinin, adenosine, isoproterenol, and sodium nitroprusside were observed in both groups. The vasoconstrictive agents vasopressin, endothelin-1, potassium chloride, and thromboxane A2 also induced similar responses in both groups; however, the trained group had an increased constrictive response to norepinephrine compared to the control rats. The results of our study show that renal resistance arteries of trained rats behave differently than conduit-type renal arteries and exhibit an increased contractile response to sympathetic agonists. This finding provides supporting evidence that renal blood flow markedly decreases during exercise in trained individuals.
Keywords:
INTRODUCTION
The adaptation processes that are induced by regular physical activity occur in various tissues, including blood vessels. During exercise, the release of mainly nitric oxide and other relaxing compounds that induce vascular resistance is decreased, and the response of vascular smooth muscle cells to constricting agents declines in resistance arteries in skeletal muscle and the myocardium, both of which are active during physical training.Citation1–4 However, relatively few studies have focused on the mechanisms that regulate blood flow in inactive tissues during exercise. Although the kidney is an important organ for maintaining homeostasis and is easily affected in many cardiovascular diseases, very few studies have investigated the effect of training on renal blood flow.
Previous studies on renal blood flow in trained animals are contradictory: some studies have reported that renal blood flow was decreased to a greater extent in trained animals compared to sedentary animals,Citation5 whereas other reports showed no differenceCitation6,7; however, despite these findings, it is generally accepted that renal blood flow is decreased to a lesser extent in trained animals compared to sedentary animals that are subjected to exercise of identical intensity.Citation8–10 Two principal factors are attributed to the differential response of trained animals. First, sympathetic discharge to the kidneys is decreased during bouts of exercise.Citation11 Renal vasoconstriction due to sympathetic discharge occurs during the first few seconds of exercise, whereas after prolonged exercise the production of local factors prevails and leads to an increase in vascular tonus by affecting the endothelial layer or the smooth muscles in renal arteries.Citation12 Second, the response to norepinephrine (NE) in renal vessels is attenuated.Citation10,13 The role of physical training in the differentiation of renal vascular responses to local vasoconstrictive factors was investigated in only one study in which the main renal artery obtained from exercise-trained pigs had a decreased contractile response to NE.Citation13 However, it is well known that local tissue perfusion is primarily controlled by resistance rather than conduit-type arteries. Therefore, determining possible exercise-training-induced alterations in the reactivity of renal small arteries is particularly important. In addition, it is also not clear whether regular physical activity affects the responses of renal resistance arteries to various local vasoactive agents.
The aim of the present study was to evaluate whether the putative alterations in both the relaxant and contractile responses of renal resistance arteries may be involved in the vasoregulatory processes that occur during exercise in trained rats. We hypothesized that the vasoconstrictive effect of local mediators in decreasing renal blood flow would be attenuated in trained animals.
MATERIALS AND METHODS
Forty adult female Wistar rats (8 weeks of age) weighing 200–220 g were used in present study. The animals were housed at 23 ± 2°C in a 12/12 h light/dark cycle and had unrestricted access to standard rat chow and drinking water. The rats were assigned randomly to two different groups: sedentary control (n = 20) and exercise training (n = 20). The animals in the training group underwent sessions of swimming exercise (60 min/day, 5 days/week for 6 weeks) in a glass tank that measured 100 × 50 cm (50 cm depth) and was filled with tap water (32–34°C). The duration of the first swimming session was 10 min, and this was increased by 10 min daily until a total of 60 min duration was reached. The experimental protocol was approved by the Animal Care and Usage Committee of Akdeniz University and followed the guidelines for using animals in experimental research.
The animals were sacrificed under ether anesthesia by exsanguination from the abdominal aorta 2 days after the last exercise bout in the training group. The kidneys were removed and transferred to a dissecting dish filled with ice-cold physiological saline solution (PSS) containing the following (in mM): 110 NaCl, 5 KCl, 24 NaHCO3, 1 KH2PO4, 1 MgSO4, 2.5 CaCl2, 0.02 EDTA, and 10 glucose. The decapsulated kidneys were cut longitudinally into two sections. The veins and arteries were carefully dissected under adequate magnification using a dissecting microscope (OLYMPUS-SZ61, Tokyo, Japan). Sections of the arcuate artery were dissected, and segments of up to 2 mm in length were isolated from the third branch and cleared of connective tissue. The vessel segments (200–250 μm in diameter) were then mounted on wire myography equipment (EMKA Technologies, Paris, France). Two fine tungsten wires (25 μm in diameter) were placed through the lumen of the vessel segments. While taking care to avoid damage to the inner surface, the rings were then horizontally mounted in organ bath chambers containing 10 mL of PSS solution according to the manufacturer’s instructions. One wire was anchored to the equipment’s removable mounting jaw and the other wire was connected to an isometric force transducer (ELG-S270B, Entran Sensors and Electronics, Toulouse, France), thereby allowing the wires to be fixed in two steps. The bath medium was bubbled with a mixture of CO2 (5%) and O2 (95%) and was maintained at 37°C (pH 7.4). The optimal resting tension (90 mmHg) of each ring was determined by constructing a passive diameter–tension curve in which the vessel’s length was kept constant using a computer program (Normalize v1.0, EMKA Technologies). The preparations were allowed to equilibrate under optimal resting tension for 60 min before the start of the experiment. During this resting period, the bath solution was changed every 15 min. After equilibration, tissue viability was confirmed by contraction in response to NE (10−7 M) in PSS containing 20 mM K+ solutions. Several washes were performed to remove the constricting agents, and the preparations were allowed to stabilize for 30 min. Endothelial integrity was then assessed by pre-contracting the tissues with NE (10−6 M) before recording the degree of relaxation elicited with acetylcholine (ACh, 10−6 M). Tissue that exhibited relaxation that was 80% greater than its pre-constriction value was considered to contain a functional endothelium. After an additional 30 min period that included a wash every 15 min, the contractility of the arteries in high K+ concentration (80 mM) was measured. In bath solutions containing elevated K+ levels, the additional K+ was replaced with equivalent amounts of Na+ to obtain a certain K+ concentration.
All preparations underwent an identical initial procedure and were then assigned to two different protocols in which the effects of relaxing and constricting agents were separately examined.
Relaxation Protocol
Vascular preparations were pre-contracted with a single submaximal concentration of NE (10−6 M). When the contractile response to the agonist was stable, increasing concentrations of ACh (10−9–3 × 10−5 M) were applied in a cumulative fashion. The following steps were performed with 30 min washing and re-equilibration intervals between applications of the various agents. After pre-constriction with NE (10−6 M), the following relaxing agents were administered sequentially: adenosine (10−9–3 × 10−5 M), bradykinin (10−15–3 × 10−8 M), sodium nitroprusside (10−9–3 × 10−5 M), and isoproterenol (10−9–3 × 10−5 M).
Contraction Protocol
Concentration–response curves were generated by sequentially applying the following vasoconstrictive agents (with 30 min washing and re-equilibration steps between each application): NE (10−83 × 10−5 M), thromboxane A2 (10−9–10−6 M), KCl (20, 40, and 80 mM), endothelin-1(10−11–10−8 M), and vasopressin (10−11–3 × 10−8 M).
The myograph recordings were analyzed using a computer program (IOX-Datanalyst 2.0.0.6, EMKA Technologies). The NE-induced contraction plateau was considered to be 100%, and the relaxation responses were calculated as percentages of this plateau. The contraction responses are presented numerically in grams and indicate the tension exerted by the vessel segments.
The results are presented as mean ± SE. The data obtained for the different concentrations of a given relaxing or contracting agent were compared by an analysis of variance for repeated measures (repeated measures ANOVA). Maximal responses observed in the concentration–response curves were analyzed with the Mann–Whitney U-test to evaluate the difference between the two experimental groups. Differences with a p-value of <0.05 were considered significant.
RESULTS
The body weight between the groups did not differ at the end of the study. The dose–response curves for the various relaxing agents are presented in . The curve patterns for the relaxing agents were not statistically different between the sedentary control and exercised groups. The maximum relaxation responses were also similar in these two groups ().
Figure 1. Cumulative concentration–response curves to acetylcholine (A), adenosine (B), bradykinin (C), isoproterenol (D), and sodium nitroprusside (E) in renal resistance arteries from experimental groups.
Note: Values are means ± SE.
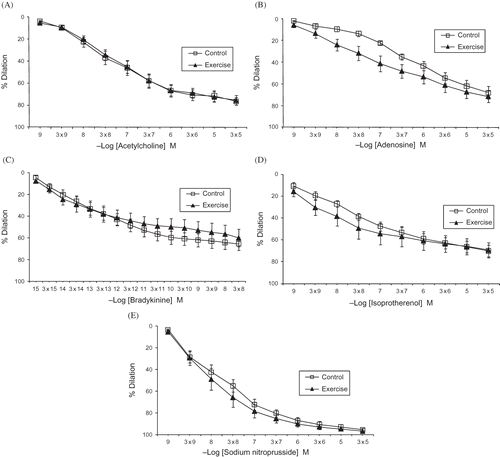
Figure 2. Cumulative concentration–response curves to norepinephrine (A), potassium chloride (B), endothelin (C), thromboxane A2 (D), and vasopressin (E) in renal resistance arteries from experimental groups.
Notes: Values are means ± SE.
*Denotes p < 0.01, difference from control group.
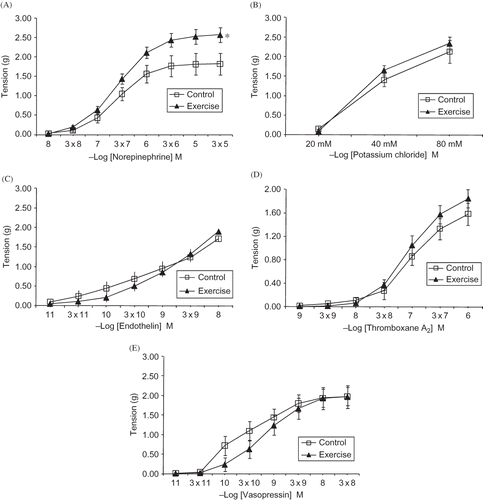
The dose–response curves obtained with the vasoconstrictor agents are presented in . NE was the only agent that generated a significantly different response (p < 0.01) between the experimental and control groups. The maximum response to NE in the exercised group was significantly greater than that in the control group (p < 0.05). The concentration–response patterns and maximum contractile responses to the other vasoconstrictive agents tested (thromboxane A2, KCl, endothelin-1, and vasopressin) were not statistically different between the control and exercised groups ().
DISCUSSION
Metabolic changes due to regular physical activity have been the subject of numerous detailed studies,Citation5–8 many of which investigated the underlying mechanisms that contribute to increased perfusion of active tissues during exercise, including skeletal muscle and myocardium. This process, referred to as “blood redistribution,” is required to supply the increased oxygen that is demanded by active tissues and is more effective in exercise-trained subjects than sedentary ones. However, the decline in blood supply to several tissues, including the kidney, also participates in the blood redistribution process. Although several investigators have suggested that the decrease in renal blood flow during exercise occurs to a lesser extent in trained subjects than in sedentary ones,Citation8–10 few studies have focused on the possible mechanisms that might contribute to this phenomenon. In the present study, we tested the responses of renal resistance arteries to various vasoactive substances in trained and sedentary rats to reveal possible exercise-training-induced alterations in renal vascular reactivity. Increased vasoconstrictive responsiveness to NE in the trained group indicates the necessity for re-evaluating the conclusions that suggest a lesser decrease in renal blood flow in trained animals.
Blood flow redistribution during a bout of exercise is explained by training-induced adaptations in cardiac output and vascular reactivity.Citation3 Several investigators have reported that vascular alterations in coronary, skeletal muscle, or intestinal vasculature can be induced by training.Citation14–18 It has been proposed that this training-related vascular adaptation is a complex process that is strongly dependent on the size and tissue location of the vessel. In other words, exercise training may trigger different adaptation mediators in different tissues that contain vessels of different sizes.Citation19,20 However, vascular adaptation to exercise training also occurs in the splanchnic organs and the kidney, in which blood flow decreases during a bout of exercise. Lash et al.Citation16 demonstrated that the intestinal vasculature exhibits an enhanced myogenic but suppressed adrenergic constrictor response in trained animals. In contrast, McAllister et al.Citation13 reported decreased constrictive responses in conduit renal arteries that were treated by cumulative doses of NE in trained pigs. However, the data obtained in conduit-type arteries cannot be extrapolated to the smaller resistance arteries in terms of regulating local tissue perfusion. It is also possible that exercise training alters the vascular responses of resistance arteries to relaxing agents.
We found no statistically significant differences in the dilation responses of renal resistance arteries to ACh or bradykinin between trained and sedentary rats. Moreover, the relaxation responses to sodium nitroprusside and isoproterenol (which are vasodilator agents with direct effects on vascular smooth muscle cells) did not differ between the trained and sedentary groups. Furthermore, high extracellular K+ concentrations (which induce vasoconstriction by membrane depolarization without involving specific receptors or post-receptor mechanisms) produced similar contractile responses in the two groups. Taken together, these results indicate that the contractile elements in vascular smooth muscle cells of renal resistance arteries were not affected in the trained rats. Our findings in rat renal resistance arteries are consistent with previous reports by other groups who studied conduit-type renal arteries from trained pigs.Citation13 Exercise training did not alter the responsiveness of renal resistance arteries to endothelium-derived vasodilatory agents at either the receptor or post-receptor level.
Table 1. Maximal dilation response (Emax) values to vasodilator agents in isolated renal resistance arteries in experimental rats.
The dose–response curves of vasoconstrictive mediators were evaluated in a subsequent set of protocols in which NE produced statistically stronger contractions in renal vessels from trained rats compared with sedentary controls. In addition, the maximal contraction responses to NE were also significantly higher in the trained rats compared to control group. In contrast, McAllister et al.Citation13 reported decreased contractile responses in renal arteries of trained pigs compared to control pigs. Although the reason for the contradictory findings is unclear, differences in the species studied, the duration of physical training, and, in particular, the vessel segment studied might all play a role in the observed differences. To our knowledge, the present study is the first to investigate exercise-training-induced alterations in the reactivity of renal resistance arteries from trained animals.
Table 2. Maximal constriction response (Emax) values to vasodilator agents in isolated renal resistance arteries in experimental rats.
There are several clues in the literature that might explain the increased NE responsiveness of resistance arteries from trained rats. Vascular alpha-adrenergic receptors undergo important changes in their number and/or their ligand affinity in response to altered levels of agonist stimulation (i.e., circulating levels of noradrenaline).Citation21 It is also well known that decreased catecholamine levels result in increased vascular sensitivity to alpha-adrenergic agonists by enhancing both receptor numbers and Ca2+ efflux.Citation22 The intensity of sympathetic stimuli and the levels of circulating adrenergic hormones are decreased by regular physical activity as compared to sedentary conditions.Citation10 Therefore, it might be suggested that the increased contractile responses of renal resistance arteries in trained rats that were observed in the present study might be due to an upregulation of adrenergic receptors and/or increased sensitivity of the post-receptor signaling pathway that is caused by a reduction in both local (through sympathetic nerves) and circulating levels of NE in these animals.
Because regular physical exercise has been reported to reduce the activity and expression (at both the mRNA and protein levels) of endothelial nitric oxide synthase, which results in a decrease in stable NO metabolites (e.g., nitrites/nitrates), an alteration in NO production might also contribute to the observed reduction in the contractile response of resistance arteries to NE in trained rats.Citation23 Moreover, Maeda et al.Citation24 reported that elevated endothelin-1 levels are responsible for renal NOS downregulation in trained rats.
Our results demonstrate that the constriction responses of renal resistance arteries to NE were stronger in trained animals compared to sedentary animals, whereas the dilation responses did not differ between these two groups. This assertion contradicts the generally accepted belief that renal blood flow decreases to a lesser extent in trained animals.Citation8–10 These findings also necessitate the re-evaluation of how kidney blood flow changes during exercise in trained animals and underscore the need for further investigation.
ACKNOWLEDGMENTS
This study was supported by the Akdeniz University Research Projects Unit (Project Number 2004.01.0200.004).
Declaration of interest: The authors report no conflicts of interest. The authors alone are responsible for the content and writing of the paper.
REFERENCES
- Chen HI, Chiang IP. Chronic exercise decrease adrenergic agonist-induced vasoconstriction in spontaneously hypertensive rats. Am J Physiol. 1996;271:H977–H983.
- Gren DJ, Maiorana A, O’Driscoll G, Taylor D. Effect of exercise training on endothelium-derived nitric oxide function in humans. J Physiol. 2004;561(pt. 1):1–25.
- Lash JM. Training-induced alteration in contractile function and excitation–contraction coupling in vascular smooth muscle. Med Sci Sports Exerc. 1998;30(1):60–66.
- Joyner MJ, Casey DP. The catecholamines strike back. What NO does not do. Circ J. 2009;73(10):1783–1792.
- Musch TI, Haidet GC, Ordway GA, Longhurst JC, Mitchell JH. Training effects on regional blood flow response to maximal exercise in foxhounds. J Appl Physiol. 1987;62(4):1724–1732.
- Clausen JP. Effect of physical training on cardiovascular adjustments to exercise in man. Physiol Rev. 1977;57(4):779–815.
- Musch TI, Terrell JA, Hilty MR. Effect of high-intensity sprint training on skeletal muscle blood flow in rats. J Appl Physiol. 1991;71(4):1387–1395.
- Armstrong RB, Laughlin MH. Exercise blood flow patterns within and among rat muscles after training. Am J Physiol. 1984;246:H59–H68.
- DiCarlo SE, Stahl LK, Bishop V. Daily exercise attenuates the sympathetic nerve response to exercise by enhancing cardiac afferents. Am J Phsiol. 1997;273:H1606–H1610.
- McAllister RM. Adaptations in control of blood flow with training: Splanchnic and renal blood flows. Med Sci Sports Exerc. 1998;30(3):375–381.
- DiCarlo SE, Bishop VS. Regional vascular resistance during exercise: Role of cardiac afferents and exercise training. Am J Physiol. 1990;258:H842–H847.
- Mueller PJ, O’Hagan KP, Skogg KA, Buckwalter JB, Clifford PS. Renal hemodynamic responses to dynamic exercise in rabbits. J Appl Physiol. 1998;85(5):1605–1614.
- McAllister RM, Kimani JK, Webster JL, Parker JL, Laughlin MH. Effect of exercise training on responses of peripheral and visceral arteries in swine. J Appl Physiol. 1996;80(1):216–225.
- Laughlin MH. Endothelium-mediated control of coronary vascular tone after chronic exercise training. Med Sci Sports Exerc. 1995;27:1135–1144.
- Koller A, Huang A, Sun D, Kaley G. Exercise training augments flow-dependent dilation in rat skeletal muscle arterioles: Role of endothelial nitric oxide and prostaglandins. Circ Res. 1995;76:544–550.
- Lash JM, Reilly T, Thomas M, Bohlen HG. Adrenergic and pressure-dependent vascular regulation in sedentary and trained rats. Am J Physiol. 1993;265:H1064–H1073.
- Chiba Y, Maehara K, Yaoita H, Yoshihisa A, Izumida J, Maruyama Y. Vasoconstrictive response in the vascular beds of the non-exercising forearm during leg exercise in patients with mild chronic heart failure. Circ J. 2007;71(6):922–928.
- Fujita M, Sasayama S. Coronary collateral growth and its therapeutic application to coronary artery disease. Circ J. 2010;74(7):1283–1289.
- Sessa WC, Pritchard K, Seyedi N, Wang J, Hintze TH. Chronic exercise in dogs increases coronary vascular nitric oxide production and endothelial cell nitric oxide synthase gene expression. Circ Res. 1994;74:349–353.
- Parker JL, Oltman CL, Muller JM, Myers PR, Adams HR, Laughlin MH. Effects of exercise training on regulation of tone in coronary arteries and arterioles. Med Sci Sports Exerc. 1994;26:1252–1261.
- Colucci WS, Gimbrone MA, Alexander RW. Regulation of the postsynaptic α-adrenegic receptor in rat mesenteric artery. Circ Res. 1981;48:104–111.
- Colucci WS, Alexander RW. Norepinephrine-induced alteration in coupling of α1-adrenegic receptor occupancy to calcium efflux in rabbit aortic smooth muscle cells. Proc Natl Acad Sci USA. 1986;83:1743–1746.
- Miyauchi T, Maeda S, Iemitsu M, . Exercise causes a tissue-specific change of NO production in the kidney and lung. J Appl Physiol. 2003;94:60–68.
- Maeda S, Miyauchi T, Iemitsu M, . Endothelin receptor antagonist reverses decrease NO system in the kidney in vivo during exercise. Am J Physiol Endocrinol Metab. 2004;286:E609–E614.