Abstract
Cisplatin (CDDP) is an anticancer drug. The clinical limitations associated with CDDP have stimulated the development of macromolecular drug-carrier systems, in attempts to decrease its toxicity. A complex (CDDP-CSA-23) between CDDP and chondroitin sulfate (CSA), a natural polysaccharide with a mean molecular weight of 23 kDa, proved to have the same anticancer activity as CDDP. A toxicodynamic study was performed on perfused kidneys to determine the effect of CDDP-CSA-23 on renal functions and the extent of platinum accumulation. The results showed that CDDP-CSA-23 attenuates the reduction in urine flow and creatinine clearance induced by CDDP. Moreover, significantly lower amounts of platinum were excreted into the urine in the case of CDDP-CSA-23, compared with CDDP alone. Meanwhile, CDDP-CSA-23 effectively retarded the rapid perfusion of platinum into kidney tissues, as occurs when CDDP is being perfused alone. The cytoprotective effects of CDDP-CSA on human proximal tubular (HK-2) cells were examined by measuring the growth of HK-2 cells in the presence of CDDP or CDDP-CSA-23. Interestingly, CDDP-CSA-23 was found to have a significantly reduced cytotoxicity, compared to CDDP. These results suggest that CDDP-CSA-23 greatly decreased the negative effects of CDDP on glomerular filtration and tubular transport in kidneys at early stages of its administration.
INTRODUCTION
Cisplatin (CDDP) is one of the most potent anticancer drugs that is currently in use and is effective against a wide spectrum of tumors. Its efficacy appears to increase with dose,Citation1 but high doses are associated with severe adverse effects, especially nephrotoxicity.Citation2 Nephrotoxicity has been observed in as many as 38% of patients after a single dose of CDDP (100 mg/mCitation2 body surface area).Citation3 This could be due to the long biological half-life of CDDP in the kidney, compared to other tissues,Citation4 as a result of the selective uptake of CDDP by the kidney via the organic cation transporter 2 (OCT2).Citation5 The toxic metabolites of CDDP induce apoptosis, inflammation, and fibrogenesis that eventually results in tubular damage and tubular dysfunction leading to the loss of sodium, potassium, and magnesium. This renal injury is presented mainly as a reversible decrease in glomerular filtration, but irreversible injuries are not impossible.Citation2
Therefore, preventing platinum from accumulating in the kidney seems to be a reasonable and a potentially effective strategy for modifying its nephrotoxicity. Santoso et al.Citation6 reported that intravenous hydration and diuresis can be used clinically as protective methods, but the nephrotoxicity resulting from high doses of CDDP continues to be a pressing problem. A number of trials for overcoming this problem using additional agents have been attempted. The use of protective agents can, to some extent, be effective in controlling CDDP nephrotoxicity.Citation7,Citation8
We previously reported that chondroitin sulfate (CSA; mean molecular weight, 23 kDa), a naturally occurring polysaccharide that contains free carboxyl and sulfate groups, can be used as a macromolecular carrier because it binds with CDDP to form a soluble complex (CDDP-CSA-23).Citation9,10 This CDDP-CSA-23 complex was reported to be as effective as CDDP alone in inhibiting the growth of several types of cancer cell lines (SW4800 human colon cancer cells, Hela human cervix cancer cells).Citation11 In addition, a study using tumor cell bearing mice clearly demonstrated that while the distribution of CDDP-CSA-23 in cancer tissues was higher than CDDP alone, the accumulation of CDDP-CSA-23 in the kidney was suppressed compared to CDDP alone.Citation11 Moreover, CDDP-CSA-23 was reported to be effective in protecting the kidney against the nephrotoxicity associated with high doses of CDDP.Citation12
In this study, we report on the mechanism associated with preventing nephrotoxicity by the CDDP-CSA-23 complex using a perfused rat kidney model and human proximal tubular (HK-2) cells, with particular emphasis on renal function.
MATERIALS AND METHODS
Materials
CDDP was purchased from Sigma Chemical Company (St. Louis, MO, USA). Chondroitin 4-sulfate samples, with mean molecular weights of 23 kDa (CSA-23) were obtained from the Seikagaku Corporation (Kanagawa, Japan). Keratinocyte serum-free medium (K-SFM) and epidermal growth factor were purchased from Gibco Life Technologies (Carlsbad, CA, USA). Bovine pituitary extract was purchased from Kurabo (Osaka, Japan). All other chemicals used were of analytical grade.
Preparation of CDDP-CSA-23 Complex
The CDDP-CSA-23 complex was prepared as follows: CDDP (1 mg/mL) and CSA-23 (8.4 mg/mL) were mixed in deionized water. This mixture at a 1:5 molar ratio (drug:monomer unit) was then shaken for 2 days until equilibrium was reached, in which 80–85% the CDDP formed a complex with CSA as determined by measuring the amount of uncomplexed CDDP present using a CE990/990-10 type capillary electrophoresis system (Jasco Co., Tokyo, Japan) with a UV spectrophotometer (CE-970, Jasco Co.).
Measurement of Glomerular Filtration and Urine Flow in Perfused Kidney
Male Sprague–Dawley rats (7 weeks old) were used in this study. The operation for preparing the perfused kidney was done as described by Newton and Hook.Citation13 A middle incision was made from the bladder to the sternum, exposing the right kidney, ureter, and inferior vena cava and aorta. A 6–8 cm long PE10 tubing was inserted up from the ureter. Catheterization was performed on the aorta, as close to the renal artery as possible. Then the aorta above the point of catheterization between the renal arteries of right and left kidneys as well as below the point was ligated. Immediately, the kidney was perfused with perfusate, consisting of 114 mM NaCl, 5.0 mM KCl, 1.0 mM NaH2PO4, 25 mM NaHCO3, 1.2 mM MgSO4, 2.0 mM CaCl2, 3.0 mM sodium acetate, 5.5 mM glucose, and 4.0 mM creatinine. The pH of the perfusion solution was 7.4 when gassed with 95% O2/5% CO2. The kidney was perfused at a constant pressure of 100 mmHg. Following a 10 min equilibration period, urine samples were collected in pre-weighted microtubes for 10 min, and the kidney was then perfused with a perfusate containing CDDP (25 μg/mL) or CDDP-CSA-23 complex (equivalent to 25 μg/mL of CDDP). Urine samples were collected through ureteral catheter at 10 min intervals for periods of up to 30 min. At that time, a perfusate sample was collected. The urine volume was determined gravimetrically (assuming the gravity of urine to be 1). The average value for the clearance of creatinine for each urine collection was calculated as that of the mid-point of each sampling. The volume of urine flow and creatinine clearance obtained within 10 min prior to perfusion with the drug was used as normal levels in calculating the percentage decrease in renal function.
Detection of the CDDP in the Urine and Kidney
The kidney was perfused with a perfusate containing CDDP (150 μg/mL) or CDDP-CSA-23 complex (equivalent to 150 μg/mL of CDDP). Urine samples were collected for 10 min, and after collecting urine sample, the kidney sample was collected. The CDDP concentrations in all samples were determined as platinum as follows: 0.1 mL of urine was diluted 2–1000 times with deionized water; kidney sample (about 0.5 g) was added to 3.5 mL of nitric acid and was dissolved for 15 min with a microwave sample digestion system (MDS-2000, CEM Company, Matthews, Germany). The digested samples were diluted with deionized water and total platinum was detected by a flameless atomic absorption spectrophotometer as described previously.Citation14
Cell Culture of Human Proximal Tubular Cell Lines
The immortalized HK-2 cell line HK-2 (American Type Culture Center, Manassas, VA, USA) was cultured at 37°C in 5% CO2 in K-SFM, supplemented with 5 ng/mL human recombinant epidermal growth factor, and 0.05 μg/mL bovine pituitary extract. HK-2 cells were seeded to tissue culture flasks and further grown to confluence until used in cellular assays.
Cell Viability Assays
Cell viability was determined after 24 and 72 h posttreatment growth in 96-well culture plates with the Cell Counting Kit-8 (Dojindo Laboratories, Kumamoto, Japan) assays according to the manufacturer’s instructions. HK-2 cells (5 × 10Citation4 cells/well) were seeded to 96-well plate and cultured for 24 h. The culture medium was then removed and washed twice with Hank’s balanced salt solution. HK-2 cells were added to 5 μL of various compound solution diluted with deionized water and 195 μL of K-SFM medium (supplement free), and cultured for 24 or 72 h. Concentrations of compounds were based on the concentration of CDDP.
Data Analysis
Data are shown as the mean ± SD for the indicated number of animals. Statistical significance was evaluated using the two-tailed, unpaired Student’s t-test for comparisons between two means, or ANOVA analysis followed by Newman–Keuls method for more than two means. A value of p < 0.05 was regarded as statistically significant.
Figure 1. Time course for urine flow in a kidney perfused without (Δ) or with 25 μg/mL of CDDP (□) or CDDP-CSA-23 (○) (equivalent to 25 μg/mL of CDDP).
Notes: Values obtained within 10 min prior to perfusion were used as control levels. All values are the mean ± SD (n = 4–5). CDDP, Cisplatin; CSA, chondroitin sulfate.
*p < 0.05 versus control; **p < 0.05 versus complex.
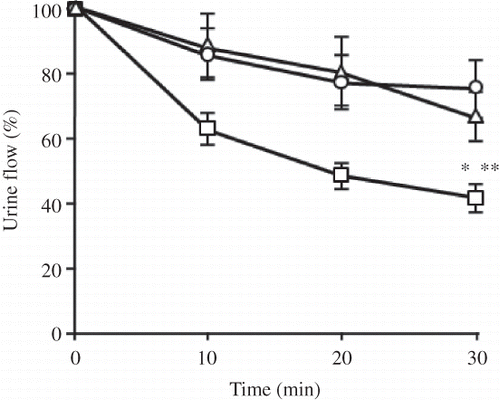
RESULTS
Effect of CDDP-CSA-23 Complex on Urine Flow
The volumes of the urine samples were measured at 10 min intervals to estimate urine flow. As shown in , a solution containing 25 μg/mL CDDP resulted in a significant decrease in urine flow in the first 10 min, compared to the control group and the CDDP-CSA-23 complex group, with approximately a 55% decrease in renal function being observed by 30 min. On the other hand, for the CDDP-CSA-23 complex group a slight decrease in urine flow occurred within 20 min, but the flow resumed within the next 10 min. However, it is noteworthy that there were no significant differences between the control and the CDDP-CSA-23 complex groups.
Effect of CDDP-CSA-23 Complex on Creatinine Clearance
CDDP is excreted from the kidney by both glomerular filtration and tubular secretion, and in this process, CDDP selectively causes injury to the proximal tubules. It is well known that the glomerular filtration rate (GFR) can be estimated based on creatinine clearance measurements. Therefore, calculating the clearance rate of creatinine is suitable for evaluating the total excretion function of the kidney.
As shown in , CDDP caused a marked decline in creatinine clearance within 10 min, and a 60% decrease was observed by 30 min, a value that is significantly lower than the control group. On the other hand, in the CDDP-CSA-23 complex group, creatinine clearance was reduced within 15 min, but recovered to the level of the control group by 30 min after perfusion.
Figure 2. Time course for the rate of clearance of creatinine in a kidney perfused without (Δ) or with 25 μg/mL of CDDP (□) or CDDP-CSA-23 (○) (equivalent to 25 μg/mL of CDDP).
Notes: Values obtained within 10 min prior to perfusion were used as control levels. All values are the mean ± SD (n = 4–5). CDDP, Cisplatin; CSA, chondroitin sulfate.
*p < 0.05 versus control; **p < 0.05 versus complex.
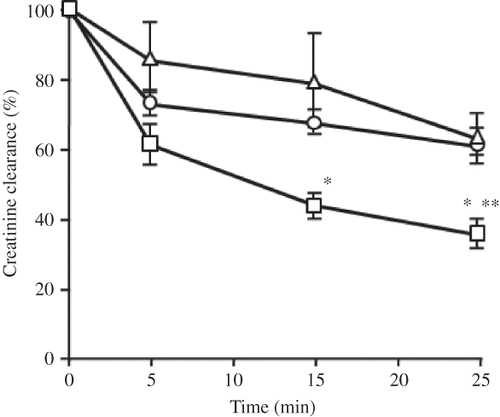
Effect of CDDP-CSA-23 Complex on Renal Excretion of CDDP and Rapid Perfusion of CDDP to Kidney
Urine samples were collected within the first 10 min of perfusion with 150 μg/mL solutions of CDDP or the CDDP-CSA-23 complex, and the concentrations of the drug in the urine samples were then determined. As shown in A, CDDP was largely excreted via the urine, accounting for half the concentration of the perfusate. On the other hand, less than one-fifth of the perfusate concentration could be accounted for in the urine of the CDDP-CSA-23 complex administered group.
Figure 3. Concentration of free platinum in urine (A) and in the kidney (B) perfused with 150 μg/mL CDDP or CDDP-CSA-23 complex (equivalent to 150 μg/mL of CDDP).
Notes: Urine was collected within the first 10 min from the perfused kidney. Values are expressed as the mean ± SD (n = 4). CDDP, Cisplatin; CSA, chondroitin sulfate.
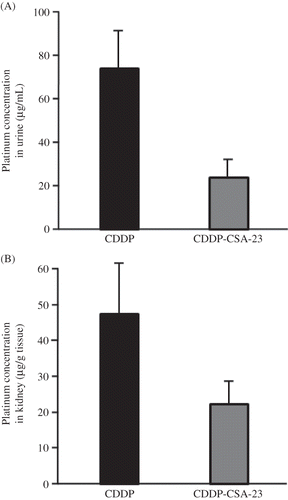
After a 10 min perfusion with 150 μg/mL of solution of CDDP or the CDDP-CSA-23 complex, the platinum content in the kidneys was determined and the results are shown in B. In addition to extensive excretion via the urine, substantial amounts of CDDP appear to have been retained in the kidney tissue and about one-third of the perfusate had accumulated within the 10 min period. On the other hand, CDDP-CSA-23 effectively controlled the rapid perfusion at a level equivalent to approximately half of that observed for the case of CDDP perfusion.
Cytoprotective Effects of CDDP-CSA-23 Complex in Human Proximal Tubular Cells
The cytoprotective effects of CDDP-CSA-23 in HK-2 cells were examined by measuring the growth of HK-2 cells for 24 and 72 h (). The cell viability of CDDP was decreased in a dose-dependent manner, whereas CDDP-CSA-23 complex significantly reduced cytotoxicity of CDDP for 24 h. Furthermore, similar trends can be observed for 72 h. These results suggest that CDDP-CSA-23 complex recovered not only the glomerular filtering function but also the proximal tubular function from CDDP nephrotoxicity.
Figure 4. Cytoprotective effects of CDDP-CSA-23 complex in HK-2 cells for 24 h (□) and 72 h (▪).
Notes: Concentrations of compounds were based on CDDP concentration. The concentration of CSA-23 alone (42 μg/mL) was equivalent to that of CSA-23 containing 5 μg/mL CDDP. Values are expressed as the mean ± SD (n = 6). CDDP, Cisplatin; CSA, chondroitin sulfate; HK-2, human proximal tubular cells.
*, **p < 0.01 compared with control and CSA-23 for 24 and 72 h, respectively.
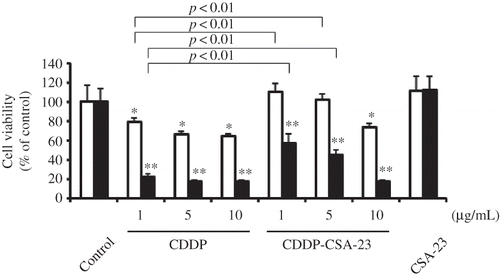
DISCUSSION
Nephrotoxicity is the most common dose-limiting side effect associated with the clinical use of CDDP. In general, nephrotoxicity caused by CDDP is characterized by a reduced renal function and severe renal injury that is mainly due to reactive oxygen species.Citation15–17 Unbound CDDP binds irreversibly to low-molecular-weight nucleophiles and macromolecules in the plasma and tissues, such as renal tubular cells, and causes tubular dysfunction that leads to a reduction in the GFR.
We previously reported that a CDDP-CSA-23 complex not only showed marked antitumor activity but also showed decreased renal accumulation over long-term administration.Citation12 As shown in and , CDDP-CSA-23 did not cause any impairment of urine flow and creatinine clearance, which corresponds to tubular dysfunction and GFR, respectively. This protective effect could be due to the stability of the CDDP-CSA-23 complex and the possibility that complexation prohibited CDDP from interacting with the low-molecular-weight nucleophiles and macromolecules within the kidney. In fact, the accumulation of platinum in urine and kidney was suppressed in the case of CDDP-CSA-23 as compared to CDDP alone ( and B).
Moreover, CDDP rapidly binds to plasma proteins such as albumin, and the protein-bound species have an extended half-life which is close to that of plasma protein turnover.Citation18,Citation19 In our previous study, we reported that about 30% of the CDDP is present in the plasma as the free drug per dose administered and that the majority of the platinum is irreversibly bound to plasma proteins. On the other hand, over 60% of the administered CDDP-CSA-23 complex was available as the complex in the plasma. It can thus be concluded that CDDP-CSA-23 is stable in the general circulation, so much so that the complexation prevents CDDP from binding with plasma proteins.Citation9
In the present study, the cytoprotective effects of CDDP-CSA-23 were evaluated in HK-2 cells. CDDP-CSA-23 was found to have a significantly reduced cytotoxicity, compared to CDDP alone (). HK-2 cells were assayed under serum-free conditions to exclude the effect of the binding of CDDP to proteins. This allows a direct and detailed evaluation of the cytotoxicity of CDDP and CDDP-CSA-23. Recently, the OCT2 and multidrug and toxin extrusion transporter in the kidney have been reported to play a role in the pharmacokinetics of platinum agents.Citation20 The administration of a single toxic dose of CDDP to rats reduces OCT2 mRNA levels and increases efflux transporter mRNA after 7 days, suggesting that a defense response is induced against subsequent exposure and the renal accumulation of CDDP.Citation21 In this study, HK-2 cells were exposed to CDDP for 24 h, so that the expression of the influx and efflux transporters of CDDP could not be affected in this experimental period. On the other hand, in human embryonic kidney 293 (HEK293) cells stably expressing OCT2, CDDP uptake was increased by only 1.3-fold compared with the control.Citation22 This, therefore, suggests that the cellular uptake of CDDP by OCT2 was inhibited by the complexation of CDDP with CSA-23. Moreover, CDDP-CSA-23 might also reduce the passive diffusion of CDDP into HK-2 cells. However, the precise mechanisms responsible for the cellular uptake of CDDP are largely unknown, although it is commonly assumed that both passive diffusion and facilitated or active transport mechanisms play substantial roles in the uptake of CDDP.Citation23 Thus, further studies are clearly needed to clarify the details of the mechanism of the cytoprotective effects and cellular uptake of CDDP-CSA-23.
The nephrotoxic effect of CDDP causes a decrease in the filtering capacity of the kidney, with a corresponding increase in creatinine and blood urea nitrogen, as has been observed in animals. We reported previously that renal injury caused by CDDP could be suppressed through complexation with CSA-23.Citation12 The results obtained in the present study are in agreement with our previous in vivo studies that CDDP-CSA-23 would suppress both renal injury and the deterioration of renal function.
However, in our previous and present studies, an isolated perfused kidney and renal function were being monitored within 30 min after perfusion with native CDDP or CDDP-CSA-23, which may not reveal a complete picture of the effect of nephrotoxicity. Hence, further long-term in vivo observations are required so that the data can be extrapolated for use in clinical settings for humans. Moreover, renal blood flow decreases after CDDP infusion, and consequently decreases the GFR. The changes in GFR and renal blood flow probably reflect an increased renal vascular resistance secondary to tubular-glomerular feedback from increased sodium chloride delivery to the macula densa.Citation24 In contrast, the glomerulus undergoes no obvious morphologic changes. In animal models, CDDP damages the proximal tubules, specifically the S3 segment of the outer medullary stripe.Citation25 Hence, CDDP treatment would be expected to result in impaired tubular function.Citation26 On the other hand, as the kidneys of rats are structurally different from humans, being unilobular and unipapillary with a direct flow of urine into the renal pelvis, further study on this anatomical species difference is needed.
In conclusion, in this study, using an isolated perfusion kidney as a model, we were able to show that the CDDP-CSA-23 complex suppresses the deterioration of renal function, compared to CDDP alone. CDDP-CSA-23 suppressed not only renal injury but also the deterioration of renal function, as evidenced by urine flow and GFR, compared to CDDP alone. Moreover, the complex was shown to have similar pharmacological activity to native CDDP and improved pharmacokinetic properties. The complexation of CDDP with CSA-23 is effective in reducing nephrotoxicity without compromising antitumor activity; thus it is a good candidate for future development as a novel anticancer drug with a much reduced renal toxicity.
Declaration of interest: The authors report no conflicts of interest. The authors alone are responsible for the content and writing of the paper.
REFERENCES
- Ozols RF, Ihde DC, Linehan WM, Jacob J, Ostchega Y, Young RC. A randomized trial of standard chemotherapy v a high-dose chemotherapy regimen in the treatment of poor prognosis nonseminomatous germ-cell tumors. J Clin Oncol. 1988;6:1031–1040.
- Yao X, Panichpisal K, Kurtzman N, Nugent K. Cisplatin nephrotoxicity: A review. Am J Med Sci. 2007;334:115–124.
- Shord SS, Thompson DM, Krempl GA, Hanigan MH. Effect of concurrent medications on cisplatin-induced nephrotoxicity in patients with head and neck cancer. Anticancer Drugs. 2006;17:207–215.
- Choie DD, del Campo AA, Guarino AM. Subcellular localization of cis-dichlorodiammineplatinum(II) in rat kidney and liver. Toxicol Appl Pharmacol. 1980;55:245–252.
- Filipski KK, Mathijssen RH, Mikkelsen TS, Schinkel AH, Sparreboom A. Contribution of organic cation transporter 2 (OCT2) to cisplatin-induced nephrotoxicity. Clin Pharmacol Ther. 2009;86:396–402.
- Santoso JT, Lucci JA, 3rd, Coleman RL, Schafer I, Hannigan EV. Saline, mannitol, and furosemide hydration in acute cisplatin nephrotoxicity: A randomized trial. Cancer Chemother Pharmacol. 2003;52:13–18.
- Ali BH, Al Moundhri MS. Agents ameliorating or augmenting the nephrotoxicity of cisplatin and other platinum compounds: A review of some recent research. Food Chem Toxicol. 2006;44:1173–1183.
- Hausheer FH, Kanter P, Cao S, . Modulation of platinum-induced toxicities and therapeutic index: Mechanistic insights and first- and second-generation protecting agents. Semin Oncol. 1998;25:584–599.
- Zhang JS, Shen-Feng MA, Suenaga A, Otagiri M. Stability of a cisplatin-chondroitin sulfate A complex in plasma and kidney in terms of protein binding. Biol Pharm Bull. 2001;24:970–972.
- Zhang JS, Anraku M, Kadowaki D, Spectroscopic studies of interactions of chondroitin sulfates with cisplatin. Carbohydr Res. 2011;346:631–637.
- Zhang JS, Imai T, Suenaga A, Otagiri M. Molecular-weight-dependent pharmacokinetics and cytotoxic properties of cisplatin complexes prepared with chondroitin sulfate A and C. Int J Pharm. 2002;240:23–31.
- Zhang JS, Imai T, Otagiri M. Effects of a cisplatin-chondroitin sulfate A complex in reducing the nephrotoxicity of cisplatin. Arch Toxicol. 2000;74:300–307.
- Newton Jr. JF, Hook JB. Isolated perfused rat kidney. Methods Enzymol. 1981;77:94–105.
- Imai T, Fujii K, Shiraishi S, Otagiri M. Alteration of pharmacokinetics and nephrotoxicity of cisplatin by alginates. J Pharm Sci. 1997;86:244–247.
- Blachley JD, Hill JB. Renal and electrolyte disturbances associated with cisplatin. Ann Intern Med. 1981;95:628–632.
- Rasoulian B, Jafari M, Mahbod M, . Pretreatment with oxygen protects rat kidney from cisplatin nephrotoxicity. Ren Fail. 2010;32:234–242.
- Chirino YI, Pedraza-Chaverri J. Role of oxidative and nitrosative stress in cisplatin-induced nephrotoxicity. Exp Toxicol Pathol. 2009;61:223–242.
- Ivanov AI, Christodoulou J, Parkinson JA, . Cisplatin binding sites on human albumin. J Biol Chem. 1998;273:14721–14730.
- Neault JF, Tajmir-Riahi HA. Interaction of cisplatin with human serum albumin. Drug binding mode and protein secondary structure. Biochim Biophys Acta. 1998;1384:153–159.
- Nakamura T, Yonezawa A, Hashimoto S, Katsura T, Inui K. Disruption of multidrug and toxin extrusion MATE1 potentiates cisplatin-induced nephrotoxicity. Biochem Pharmacol. 2010;80:1762–1767.
- Aleksunes LM, Augustine LM, Scheffer GL, Cherrington NJ, Manautou JE. Renal xenobiotic transporters are differentially expressed in mice following cisplatin treatment. Toxicology. 2008;250:82–88.
- Burger H, Zoumaro-Djayoon A, Boersma AW, . Differential transport of platinum compounds by the human organic cation transporter hOCT2 (hSLC22A2). Br J Pharmacol. 2010;159:898–908.
- Gately DP, Howell SB. Cellular accumulation of the anticancer agent cisplatin: A review. Br J Cancer. 1993;67:1171–1176.
- Cornelison TL, Reed E. Nephrotoxicity and hydration management for cisplatin, carboplatin, and ormaplatin. Gynecol Oncol. 1993;50:147–158.
- Vickers AE, Rose K, Fisher R, Saulnier M, Sahota P, Bentley P. Kidney slices of human and rat to characterize cisplatin-induced injury on cellular pathways and morphology. Toxicol Pathol. 2004;32:577–590.
- Lajer H, Kristensen M, Hansen HH, . Magnesium depletion enhances cisplatin-induced nephrotoxicity. Cancer Chemother Pharmacol. 2005;56:535–542.