Abstract
Background: Long-term peritoneal exposure to high glucose in the peritoneal dialysis (PD) solution may potentiate the development of peritoneal fibrosis in PD patients. The most important factor leading to peritoneal fibrosis may be injury of human peritoneal mesothelial cells (HPMC). Little is known about senescence of HPMC. It has been reported that Tanshinone IIA can ameliorate fibrosis. Whether Tanshinone IIA may delay senescence and protect HPMC against high glucose is not clear. The aim of this study is to investigate the protective role of Tanshinone IIA in senescence of HPMC induced by high glucose. Methods: HPMC were isolated and cultured with Roswell Park Memorial Institute 1640 medium containing high glucose concentrations (2.5%) and Tanshinone IIA (50 μmol/L and 100 μmol/L). The effects of high glucose and Tanshinone IIA on cellular senescence of HPMC were examined by observing cell generation, growth rate, cell cycle, positive rate of senescence-associated β-galactosidase (SA-β-gal) staining, telomere length, and expression of p16 and p21. Results: Compared with the control cells, HPMC cultured in high glucose showed decreased cell generations by four to five and suppression of growth rate, and the cell cycle was stopped at G1 phase. The positive rate of SA-β-gal staining was increased; the telomere length was shortened; and the expressions of p16 and p21 were increased. The characteristics in morphology of senescent cells appeared earlier. Tanshinone IIA may delay the process of senescence of HPMC induced by high glucose by increasing cell generations and growth rate, decreasing the rate of G1 phase and the positive rate of SA-β-gal staining, lengthening the telomere, and decreasing the expression of p16 and p21. Conclusions: Tanshinone IIA may protect HPMC through delaying cellular senescence induced by high glucose.
INTRODUCTION
Peritoneal dialysis (PD) has been established as one of the main renal replacement therapies for end-stage renal failure. However, long-term peritoneal exposure to high glucose and other unphysiologic contents in the PD solution may potentiate the development of peritoneal fibrosing syndrome (PFS) in PD patients. PFS is composed of a wide spectrum of peritoneal alterations, which has been observed in PD patients.Citation1 Better understanding of how the PD solution added with high glucose affects the mesothelial cell biology, together with technical advances aiming at reducing the effect of high glucose in dialysis solutions, may prolong the survival of the continuous ambulatory peritoneal dialysis technique.Citation2 The most important factor leading to PFS might be the injury of human peritoneal mesothelial cells (HPMC).Citation3 GotloibCitation4 found that high glucose induced a hypertrophic, senescent mesothelial cell phenotype after long in vivo exposure. Exposure to a high glucose concentration induced an early and short-lived acceleration of the mesothelial cell cycle, and with a longer exposure this was followed by a depletion of the growth capabilities of the exposed monolayer.Citation5 A recent study showed that chronic exposure to elevated glucose may result in the accelerated induction of senescence in HPMC in vitro, possibly through increased TGF-β1 release.Citation6 The results of Ksiazek’s study indicated that the accelerated senescence response in HPMC exposed to high glucose is strongly related to oxidative stress.Citation7 We hypothesized that high glucose may accelerate PFS by inducing a senescent mesothelial cell phenotype and delaying the senescence of HPMC may put off PFS.
Tanshinone IIA, a Chinese traditional medicine and a small compound extracted from Chinese medicine Danshen (the dried root or rhizome of Salvia miltiorrhiza Bunge), has been widely used in China and other countries for the treatment of cardiovascular and cerebrovascular diseases. Accumulating evidence suggests that Tanshinone IIA may suppress fibrosis and inflammationCitation8–12. And Tanshinone IIA significantly inhibits the PDF-induced upregulation of TGF-β1 and connective tissue growth factor (CTGF) in peritoneum and thus may potentially be used in clinic to prevent peritoneal fibrosis during long-term dialysis.Citation13 In our experiment, in order to probe into the mechanism of Tanshinone IIA-resisting peritoneal fibrosis, the effect of high glucose on cellular senescence of HPMC and resistance of Tanshinone IIA were examined.
MATERIALS AND METHODS
Isolation and Culture of HPMC
HPMC were isolated by enzymatic disaggregation of omentum as described in detail elsewhere.Citation14 Briefly, the specimens of omentum were obtained from consenting patients undergoing elective abdominal surgery. The tissue was incubated in a solution of 0.05% trypsin and 0.02% EDTA for 30 min at 37°C with gentle shaking. The cells obtained were washed and propagated in Roswell Park Memorial Institute (RPMI) 1640 culture medium supplemented with l-glutamine (2 mM), penicillin (100 U/mL), streptomycin (100 Jg/mL), hydrocortisone (0.4 Jg/mL), and 10% v/v fetal calf serum. The cultures were maintained at 37°C in a humidified atmosphere of 95% air and 5% CO2. Cells were identified as pure mesothelial by their typical cobblestone appearance at confluence and uniform positive staining for cytokeratin and HBME-1 antigen.Citation15 All cultures were established from individuals with no evidence of peritonitis and no overt diabetes, uremia, and peritoneal malignancy.
Morphologic Changes of HPMC
Primary HPMC were grown to confluence and then serially passaged at 3-day intervals. The cells of the second passage were cultured in RPMI 1640 culture medium (GIBCO, Grand Island, NY USA), RPMI 1640 culture medium added with high glucose (2.5%), and RPMI 1640 culture medium added with 2.5% mannitol (Sai Hong Rui biological science and technology Ltd., Nanjing, PR China). All cells were cultured from young to old.
Then the cells of the second passage were cultured in RPMI 1640 culture medium, RPMI 1640 culture medium added with high glucose (2.5%), added with high glucose (2.5%) and Tanshinone IIA (50 μmol/L) (purchased from Jiangsu Carefree Group Co., Nanjing, PR China), and added with high glucose (2.5%) and Tanshinone IIA (100 μmol/L). All cells were cultured from young to old. At last six groups of cells were investigated. They were termed as young HPMC, middle-aged HPMC, senescent HPMC, HPMC/Tanshinone1 (50 μmol/L), HPMC/Tanshinone2 (100 μmol/L), and HPMC/high glucose. Then cell morphologic changes of groups in different generations were observed.
Cell Growth Velocity by MTT
Totally, 2.5 × 103 HPMC per well were plated into 96-well plates. Ten microliters of 3-(4,5-dimethylthiazol-2-yl)-2,5-diphenyltetrazolium bromide (MTT; Sai Hong Rui biological science and technology Ltd.) (5 g/L) were added per well 4 h ahead of measurement at the indicated time point. After 200 μL of dimethyl sulfoxide (Sai Hong Rui biological science and technology Ltd.) was added per well in the dark for 20 min, optical density (OD) values were obtained from Microplate Reader (Bio-Rad, Hercules, CA, USA) at 492 nm wavelength.
Cell Cycle Analysis by Flow Cytometry
The cells at 70–80% confluence were washed with phosphate-buffered saline (PBS; Sai Hong Rui biological science and technology Ltd.), detached with 0.25% trypsin (Sai Hong Rui biological science and technology Ltd.), and fixed with 75% ethanol (Sai Hong Rui biological science and technology Ltd.) overnight; then they were resuspended in 1 mL PBS and stained with propidium iodide (Sai Hong Rui biological science and technology Ltd.) in the dark for 30 min. The DNA contents were measured with a fluorescence-activated cell sorting flow cytometry system (Becton Dickinson, San Jose, CA USA).
SA-β-Gal Staining
After being washed twice in PBS and fixed for 5 min at room temperature in 3% formaldehyde, the cells were incubated overnight at 37°C (without CO2) with freshly prepared senescence-associated β-galactosidase (SA-β-gal) stain solution (Sai Hong Rui biological science and technology Ltd.)(1 mg/mL X-gal, 40 mM citric acid/sodium phosphate, PH 6.0, 5 mM potassium ferrocyanide, 5 mM potassium ferricyanide, 150 mM NaCl, 2 mM MgCl2). To obtain the positive rate, six fields were chosen for each sample.
Telomere Length Assay by Southern Blot
A total of 10 μg of genomic DNA per sample was digested with restriction enzymes HinfI and RsaI (Sigma, St. Louis, MO USA), electrophoresed on a 0.7% agarose gel, and transferred onto nylon membranes (Sigma). The membrane was exposed in the ultraviolet instrument for 6 min and hybridized with a 5′-end [γ-32P] ATP-labeled telomeric (TTAGGG)5 repeat probe. After hybridization for 24 h, washing was carried out with 0.2 × SSC (sodium chloride sodium citric acid buffer; Sigma) and 0.1% sodium dodecyl sulfate (Sai Hong Rui biological science and technology Ltd.). The membrane was autoradiographed on Kodak X-ray film for 48–72 h at −70°C. The image was analyzed using VDS Image software (Beijing University, Beijing, PR China) . The mean telomere restriction fragment was defined as follows: ∑(ODi)/∑(ODi/Li), in which ODi is the densitometer output, and Li is the length of the DNA at position i.Citation16
The Expression of p16 and p21 by Western Blot
For western blotting, 40 μg denatured cell proteins were run on 10% and 15% sodium dodecyl sulfate–polyacrylamide gel, transferred onto nitrocellulose membrane (Bio-Rad), and probed with anti-p16 and anti-p21 monoclonal antibodies. The signals were detected with an enhanced chemiluminescence system.
Statistical Analysis
The experiments were performed five times. The results were expressed as the means ± SD. Differences between groups were analyzed by one-way ANOVA followed by Dunnett’s multiple comparison tests. The data were analyzed with SPSS software (SPSS Inc., Chicago, IL, USA). Differences with a p-value < 0.05 were considered to be statistically significant.
RESULTS
Proliferative Capacity and Generations of HPMC
The generations of the cells cultured in RPMI 1640 culture medium added with 2.5% mannitol were similar to the normal cells (PD8-11). The result inferred that osmotic pressure had no effect on the growth of HPMC. Compared with the control cells, the cells cultured in RPMI 1640 added with high glucose (2.5%) grew senescent ahead of 4–5 generations.
Serial passages of HPMC led to rapid exhaustion of their proliferative capacity. During the first few (1–3) passages, the seeding density of 3 × 104 cells/cm2 sufficed for HPMC to easily reach a monolayer within 3 days. However, this ability gradually declined. The calculated number of population doublings was (median and range) 8 (4–11). While all cell lines could be passaged at least 6 times, half of the cultures expired at their seventh or ninth passages, and only 25% of cultures reached a maximum of 10 passages. Even if late-passage cultures were regularly fed and maintained in culture for additional 2 months, the number of cells did not increase any further. We observed the generations of different cells at the same time. The results showed the generations of the cells cultured in RPMI 1640 culture medium added with high glucose (2.5%) and Tanshinone IIA (50 μmol/L and 100 μmol/L) were similar to the normal cells (PD8-11); Compared with the control cells, the cells cultured in RPMI 1640 culture medium added with high glucose (2.5%) grew senescent ahead of 4–5 generations.
Cell Growing Speed by MTT
MTT () showed that the growing rate of HPMC/high glucose (PD5) was slower than that of the HPMC (PD5) and similar to senescent cells (PD10). The growing rates of HPMC/Tanshinone were similar to that of middle-aged HPMC (PD5). For example, at the time point of 72 h, OD value by MTT dropped by 40% in the HPMC/high glucose.
Figure 1. Growth curves of young HPMC, HPMC/Tanshinone1, HPMC/Tanshinone2, middle-aged HPMC, senescent HPMC, and HPMC/high glucose by MTT [3-(4,5-dimethylthiazol-2-yl)-2,5-diphenyltetrazolium bromide] assay. The HPMC/high glucose (PD5) showed nearly complete growth inhibition like senescent HPMC (PD10). The growth rate or the growth potential of HPMC/Tanshinone1(PD5) and HPMC/Tanshinone2 (PD5) was similar to that of the middle-aged HPMC (PD5).
![Figure 1. Growth curves of young HPMC, HPMC/Tanshinone1, HPMC/Tanshinone2, middle-aged HPMC, senescent HPMC, and HPMC/high glucose by MTT [3-(4,5-dimethylthiazol-2-yl)-2,5-diphenyltetrazolium bromide] assay. The HPMC/high glucose (PD5) showed nearly complete growth inhibition like senescent HPMC (PD10). The growth rate or the growth potential of HPMC/Tanshinone1(PD5) and HPMC/Tanshinone2 (PD5) was similar to that of the middle-aged HPMC (PD5).](/cms/asset/697e2f45-8e7a-4551-ab36-6db020305aa8/irnf_a_623497_f0001_b.jpg)
Cell Cycle Changes
The cell cycle profile was analyzed by flow cytometry (). HPMC/high glucose had a similar percentage of cells in G1 phase as normal senescent HPMC and G1 phase percentage of HPMC/Tanshinone was similar to that of middle-aged HPMC (PD5). The results suggested that the G1 arrest was caused by high glucose and Tanshinone IIA might postpone G1 cell arrest. There were no differences between the effects of Tanshinone IIA of 50 μmol/L and 100 μmol/L.
Table 1. The rate of cell cycle of HPMC.
Cell Morphologic Changes and the SA-β-Gal Senescence-Associated Marker
The HPMC/high glucose (PD5) showed increasing gross enlargement and accumulation of granular cytoplasmic inclusions like the senescent cells (PD10). However, the morphologies of HPMC/Tanshinone (50 μmol/L and 100 μmol/L) (PD5) were similar to the middle cells (PD5) ().
Figure 2. Morphology and SA-β-gal senescence-associated marker. Cells were microphotographed at a magnification of 10 × 10. (A) Young HPMC (PD2), (B) middle-aged HPMC (PD5), (C) senescent HPMC (PD10), (D) HPMC/Tanshinone1 (PD5), (E) HPMC/Tanshinone2 (PD5), and (F) HPMC/high glucose (PD5).
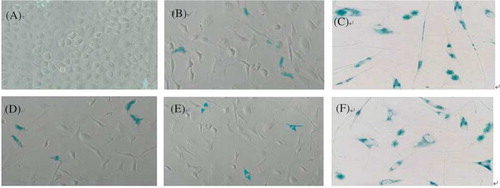
Figure 3. (A) The mean terminal restriction fragment (TRF) length of telomere for six groups of HPMC. M: marker; lane 1: young HPMC (PD2); lane 2: HPMC/Tanshinone1 (PD5); lane 3: middle-aged HPMC (PD5); lane 4: HPMC/Tanshinone2 (PD5); lane 5: senescent HPMC (PD10); lane 6: HPMC/high glucose (PD5). (B) The comparisons of the TRF length of telomere in six groups.
Note: *p < 0.05 versus the middle-aged HPMC (PD5).
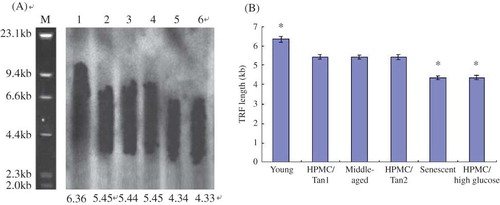
The specific senescence-associated marker, pH 6.0 optimum β-galactosidase (SA-β-gal), was assayed by X-gal staining (). Only sporadic SA-β-gal-positive cells were seen in young HPMC (PD2), whereas almost all of HPMC/high-glucose were strongly stained, as were the senescent cells (PD10). The positive rate in the HPMC/Tanshinone (50 μmol/L) (PD5) (27 ± 4%) was similar to that in the middle-aged cells (PD5) (28 ± 3%). There were no differences between the positive rate of two groups of HPMC cultured in the Tanshinone IIA of 50 μmol/L and 100 μmol/L (27 ± 3%) (p > 0.05).
Terminal Restriction Fragment Assay for the Length of Telomere
Southern blot and statistic analysis showed the mean terminal restriction fragment (TRF) length was 6.36 ± 0.15 kb in the young HPMC (PD2), 5.46 ± 0.12 kb in the HPMC/Tanshinone1 (PD5), 5.45 ± 0.10 kb in the middle-aged HPMC (PD5), 5.44 ± 0.13 kb in the HPMC/Tanshinone2 (PD5), 4.35 ± 0.10 kb in the senescent HPMC (PD10), and 4.36 ± 0.11 kb in the HPMC/high glucose.
The results suggested the TRF length of the young cells was longer than those of any other groups (p < 0.05) and the TRF length had no significant differences among three groups of two HPMC/Tanshinone and middle-aged HPMC (p > 0.05), whereas the TRF length in the HPMC/high glucose like the senescent cells was shorter than those of HPMC/Tanshinone and the middle-aged cells (A and B).
Figure 4. (A) Western blot showing the expression of p16 and p21 for six groups of HPMC—lane 1: young HPMC (PD2); lane 2: HPMC/Tanshinone1 (PD5); lane 3: HPMC/Tanshinone2 (PD5); lane 4: middle-aged HPMC (PD5); lane 5: senescent HPMC (PD10); lane 6: HPMC/high glucose (PD5). (B) The comparisons of p16/actin in six groups; *p < 0.05 versus the middle-aged HPMC (PD5). (C) The comparisons of p21/actin in six groups; *p < 0.05 versus the middle-aged HPMC (PD5).
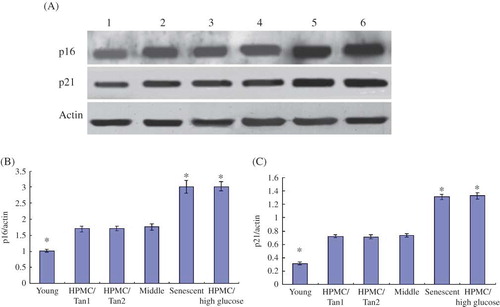
Measurement of Expression of p16 and p21 with Western Blot
The expressions of p16 and p21 in HPMC/high glucose (PD5) were similar to that in the senescent cells (PD10) (p > 0.05), which was more than middle-aged and young cells (p < 0.05); however, the expression of p16 and p21 had no significant difference among the two groups of HPMC/Tanshinone and middle-aged cells (p > 0.05). The expression of p16 and p21 in the young cells (PD2) showed the lowest in all groups (p < 0.05) (A–C). The results suggested that the expression of p16 and p21 in the normal HPMC increased with aging and high glucose led to the increased expression of p16 and p21. At the same time, Tashinone IIA might decrease the expression of p16 and p21.
DISCUSSION
Previous studies with HPMC commented on early cessation of cell growth and rapid appearance of enlarged vacuolated cells.Citation14,17 With this study we confirm these observations and show that the loss of proliferative capacity by HPMC is associated with the development of other features of a senescent phenotype. They include enlarged morphology, decreased cell generations, suppression of growth rate, increased percentage of cells arrested in the G1 phase of the cell cycle, and the presence of SA-β-gal, commonly used marker of senescence in vitro.Citation18 In addition, we detected shortened telomere length and increased expression of p16 and p21 in late-passage HPMC and HPMC cultured in high glucose. This effect was previously described in senescent human fibroblasts.Citation19
To protect the mesothelial cells effectively, many investigations and experiments had been done in China and other countries. Tanshinone IIA could pass to rise the SOD level inside the diabetic rats to lower its H2O2 and malondialdehyde content. Sulfotanshinone sodium can delay the interstitial fibrosis in diabetic nephropathy rats. Moreover, Tanshinone might restrain the proliferative activity of the peritoneal mesothelial cells and inhibit oxidative stress and TGF-β1 secretion.
It is not clear whether high glucose may cause cell senescence of HPMC and Tanshinone IIA can resist the effect of high glucose. To answer this question, high glucose and Tanshinone IIA were added into the RPMI 1640 culture medium. Compared with the controlled cells, high glucose accelerated the aging of HPMC. HPMC cultured in RPMI 1640 culture medium with high glucose showed decreased cell generation by four to five, significant suppression of cell growth rate by 40% with cell cycle arrested at G1 phase, increase of SA-β-gal positive staining, and early appearance of morphology change of senescent cells. Tanshinone IIA might resist the effect of high glucose in the aging of HPMC.
The telomere length is considered as the clock of cell division and reflects cell division ability. HarleyCitation20 and his colleagues measured the telomere length with TRF in 1990. Their result suggested the cell telomere length decreased with the increase of cell mitotic activity. The gradual losing of telomere is a mechanism for senescence of the somatic cells. ZglinickiCitation21 and his colleagues reported that the accumulation of single-strand breaks by oxidative stress was the major cause of telomere shortening. H2O2 might induce cells to manifest senescent morphology and accelerate telomere shortening.Citation21
With cell aging, cell divisions are ceased with G1 arrest and the expressions of cell cycle proteins are increased. We observed that the telomere length of HPMC/high glucose was similar to that of the senescent cells, whereas the telomere length had no difference between HPMC/Tanshinone and the middle-aged cells. The result suggested that the high glucose shortened the TRF length of telomere of HPMC. The cell cycle assay showed HPMC/high glucose and the aging cells were at G1 arrest. Western blot showed that high glucose led to the increased expression of p16 and p21. All results infer that the high glucose may accelerate the process of cell aging. At the same time, Tanshinone IIA may relay the process of cell aging caused by high glucose.
Both replicative senescence and stress-induced premature senescence are dependent on two major pathways. One is triggered by DNA damage, telomere damage, and/or shortening and involves the activation of the p53 and p21 (waf-1) proteins. The second pathway results in the accumulation of p16 (Ink-4a) with the MAP kinase signaling pathway as possible intermediate.Citation22 We observed the shortening of telomere length and the increased expression of p16 and p21 by high glucose. Our results suggest that the two senescent ways are involved in the senescence of HPMC by high glucose. It is inferred that loaded anergic metabolism after high glucose, the increase of oxidative stress damage, the increased metabolic products accelerating aging such as lipid peroxide and oxygenic free radix, telomere shortening, and the increased expression of p16 and p21 accelerate the cell senescence.
Recently, several studies have shown that Tanshinone IIA possesses remarkable antifibrosis properties.Citation8–12 Tanshinone IIA can attenuate cardiac fibrosis and modulate collagen metabolism in rats with renovascular hypertension.Citation23 It also prevents hepatic and renal fibrosis.Citation24,25 Our study is the first to show the beneficial effects of Tanshinone IIA on preventing the senescence of HPMC when it is combined with high glucose. In the other experiment, the cotreatment of PDF with either low- or high-dose Tanshinone IIA significantly inhibits PDF-induced increase of TGF-β1 and CTGF expression in both parietal peritoneum and omentum. This result indicates that Tanshinone IIA is an effective agent for preventing peritoneal fibrosis.Citation13 Tanshinone IIA is a diterpene that is thought to be a major bioactive molecule in Danshen, a dietary supplement derived from the root of S. miltiorrhiza, which is widely used in China and other Asian countries. The toxicity of Tanshinone IIA in vivo and in vitro has been well studied.Citation26 Data obtained from mice model show that the LD50 of Tanshinone IIA is 25.807 g/kg, which is equivalent to approximately 4000 times the intended clinical human oral dosage. Moreover, an oral dose of 2500 mg/kg Danshen extract (400 folds the human oral dosage) for 90 days was found to be nontoxic to rats.Citation27 Data from in vitro studies also indicate that Tanshinone IIA does not inhibit the viability of rat cardiac cells.Citation11 Consistently, we found that Tanshinone IIA had no effect on cell viability in cultured HPMC. Taken together, these data suggest that it is safe to use Tanshinone IIA in clinical practice to prevent peritoneal fibrosis in patients undergoing long-term PD.
In conclusion, our results demonstrate that high glucose may accelerate the aging process of HPMC and Tanshinone IIA may protect HPMC by delaying the cell aging induced by high glucose. The experiment provides a new theorical basis to alleviate the injuring of the peritoneum and delay the peritoneal fibrosis. The mechanism of delaying the senescence of HPMC by high glucose with Tanshinone IIA may be restraining loaded energetic metabolism, decreasing oxidative stress damage, and reducing metabolic products to enhance aging, such as arresting G1, telomere shortening, and the increased expression of p16 and p21.
ACKNOWLEDGMENTS
This study was supported by the Key Science and Technology Development Program of Nanjing City of the People’s Republic of China (ZKX08026). I am grateful for my tutor, Xiangmei Chen (Department of Nephrology, General Hospital of PLA, Beijing, PR China).
Declaration of interest: The authors report no conflicts of interest. The author alone is responsible for the content and writing of the paper.
REFERENCES
- Witowski J, Wisniewska J, Korybalska K, . Prolonged exposure high glucose degradation products impairs viability and function of human peritoneal mesothelial cells. J Am Soc Nephrol. 2001;12:2434–2441.
- Hung KY, Huang JW, Tsai TJ, . Peritoneal fibrosing syndrome: Pathogenetic mechanism and current therapeutic strategies. J Chin Med Assoc. 2005;68(9):401–405.
- Leavesley DI, Stanley JM, Faull RJ. Epidermal growth factor modifies the expression and function of extracellular matrix adhesion receptors expressed by peritoneal mesothelial cells from patients on CAPD. Nephrol Dial Transplant. 1999;14:1208.
- Gotloib L, Shostak A, Wajsbist V, . High glucose induced a hypertrophic, senescent mesothelial cell phenotype after long in vivo exposure. Nephron. 1999;82(1):164.
- Shostak A, Wajsbrot V, Gotloib L. High glucose accelerates the life cycle of the in vivo exposed mesothelium. Kidney Int. 2000;58(5):2044–2052.
- Ksiazek K, Korybalska K, Jörres A, . Accelerated senescence of human peritoneal mesothelial cells exposed to high glucose: The role of TGF-beta1. Lab Invest. 2007;87(4):345–356.
- Ksiazek K, Breborowicz A, Jörres A, . Oxidative stress contributes to accelerated development of the senescent phenotype in human peritoneal mesothelial cells exposed to high glucose. Free Radic Biol Med. 2007;42(5):636–641.
- Liu T, Jin H, Sun QR, Xu JH, Hu HT. The neuroprotective effects of tanshinone IIA on beta-amyloid-induced toxicity in rat cortical neurons. Neuropharmacology. 2010;59:595–604.
- Yang L, Zou XJ, Gao X, . Sodium tanshinone IIA sulfonate attenuates angiotensin II-induced collagen type I expression in cardiac fibroblasts in vitro. Exp Mol Med. 2009;41:508–516.
- Li YI, Elmer G, Leboeuf RC. Tanshinone IIA reduces macrophage death induced by hydrogen peroxide by upregulating glutathione peroxidase. Life Sci. 2008;83:557–562.
- Fu J, Huang H, Liu J, Pi R, Chen J, Liu P. Tanshinone IIA protects cardiac myocytes against oxidative stress-triggered damage and apoptosis. Eur J Pharmacol. 2007;568:213–221.
- Tang F, Wu X, Wang T, . Tanshinone IIA attenuates atherosclerotic calcification in rat model by inhibition of oxidative stress. Vascul Pharmacol. 2007;46:427–438.
- Chunming J, Miao Z, Cheng S, . Tanshinone IIA attenuates peritoneal fibrosis through inhibition of fibrogenic growth factors expression in peritoneum in a peritoneal dialysis rat model. Ren Fail. 2011;33(3):355–362.
- Stylianou E, Jenner LA, Davies M, . Isolation, culture and characterization of human peritoneal mesothelial cells. Kidney Int. 1990;37(6):1563–1570.
- Zhang XY, Pettengell R, Nasiri N, Kalia V, Dalgleish AG, Barton DP. Characteristics and growth patterns of human peritoneal mesothelial cells: Comparison between advanced epithelial ovarian cancer and non-ovarian cancer sources. J Soc Gynecol Investig. 1999;6:333–340.
- Harley CB, Futcher AB, Greider CW. Telomeres shorten during ageing of human fibroblasts. Nature. 1990;345(6274):458–460.
- Fischereder M, Luckow B, Sitter T, Schroppel B, Banas B, Schlondorff D. Immortalization and characterization of human peritoneal mesothelial cells. Kidney Int. 1997;51:2006–2012.
- Dimri GP, Lee X, Basile G, . A biomarker that identifies senescent human cells in culture and in aging skin in vivo. Proc Natl Acad Sci USA. 1995;92:9363–9367.
- Chen X, Cao D, Wang J, . Effects of human Na(+)/dicarboxylate cotransporter 3 on the replicative senescence of human embryonic lung diploid fibroblasts. J Gerontol A Biol Sci Med Sci. 2005;60(6):709–714.
- Harley CB, Futcher AB, Greider CW. Telomeres shorten during aging of human fibroblast. Nature. 1990;345:458–460.
- von Zglinicki V, Pilger R, Sitte N. Accumulation of single-strand breaks is the major cause of telomere shortening in human fibroblasts. Free Radic Biol Med. 2000;28(1):24–27.
- Toussaint O, Medrano EE, von Zglinicki T. Cellular and molecular mechanisms of stress-induced premature senescence (SIPS) of human diploid fibroblasts and melanocytes. Exp Gerontol. 2000;35(8):927–945.
- Fang J, Xu SW, Wang P, . Tanshinone IIA attenuates cardiac fibrosis and modulates collagen metabolism in rats with renovascular hypertension. Phytomedicine. 2010;18:58–64.
- Sun RF, Liu LX, Zhang HY. Effect of Tanshinone II on hepatic fibrosis in mice. Zhongguo Zhong Xi Yi Jie He Za Zhi. 2009;29:1012–1017.
- Tang J, Zhan C, Zhou J. Effects of Tanshinone IIA on transforming growth factor beta 1-Smads signal pathway in renal interstitial fibroblasts of rats. J Huazhong Univ Sci Technol Med Sci. 2008;28:539–542.
- Wang X, Morris-Natschke SL, Lee KH. New developments in the chemistry and biology of the bioactive constituents of Tanshen. Med Res Rev. 2007;27:133–148.
- Zhou L, Zuo Z, Chow MS. Danshen: An overview of its chemistry, pharmacology, pharmacokinetics, and clinical use. J Clin Pharmacol. 2005;45:1345–1359.