Abstract
Objectives: The aim of this study was to investigate the preventive effects of melatonin and vitamin C as antioxidants on renal injury in chronic alcohol consumption. Materials and methods: A total of 24 adult male Wistar rats weighing 200–250 g were used in the study. Rats were divided into four equal groups. Group I (control): rats were not fed on alcohol; Group II: rats were fed on alcohol; Group III: rats were fed on alcohol and 40 mg/kg vitamin C; and Group IV: rats were fed on alcohol and 4 mg/kg melatonin. Results: Light microscopic examination revealed atrophic renal corpuscles, dilatation and congestion of the peritubular vessels, and renal corpuscles with obscure Bowman’s space and a few foamy-appearing tubules due to alcohol consumption were observed. Expression of endothelial nitric oxide synthase (eNOS) was localized to glomerulus, distal, and collector tubules. eNOS staining decreased in alcohol treatment group and melatonin and vitamin C encore increased expression pattern of eNOS. Alcohol consumption increased malondialdehyde (MDA) level and superoxide dismutase (SOD) and catalase (CAT) activities significantly in the alcohol consumption groups compared with that in the control group, while in melatonin give group just MDA level was decreased statistically significant and SOD and CAT activities were also decreased numerically compared with the alcohol consumption groups. Conclusions: These results indicated that chronic alcohol consumption caused renal damage by increased lipid peroxidation and melatonin and vitamin C administration produced in some degree protection against alcohol-induced damage.
INTRODUCTION
Most world populations consume alcoholic beverages. It is generally accepted that excessive alcohol consumption can induce dramatic changes in the physiological and biochemical processes of the whole organism and in the cells.Citation1 Three enzymatic systems are able to fulfill the ethanol oxidation: alcohol dehydrogenase, microsomal ethanol-oxidizing system, and catalase (CAT). About 80% of ingested ethanol is metabolized in the liver. Therefore, the structural and functional alterations of liver because of alcohol abuse have been extensively studied.Citation2–5 Excessive alcohol consumption usually causes hepatic, gastrointestinal, nervous, and cardiovascular injuries. Some clinical and experimental studies have also been focused on the effects of ethanol feeding on renal function, gross and microscopic morphology of the kidney.Citation6–8 While acute alcohol consumption has a nephrotoxic effect on the kidney, alternatively, chronic use in humans may result in alcohol-induced hypertension, indirectly increasing the risk of chronic kidney disease.Citation9,10
Oxidative stress is an imbalance favoring prooxidants and/or disfavoring antioxidants, potentially leading to tissue damage.Citation11 Oxidative stress- and reactive oxygen species (ROS)-mediated toxicity have been considered the primary routes to alcohol-induced kidney injury.Citation12,13 Thus, chronic and excessive alcohol consumption may accelerate oxidative mechanism directly or indirectly, which eventually produces cell death and tissue damage.Citation14
Ascorbic acid (vitamin C) is a low molecular mass antioxidant that interacts directly with the oxidizing radicals and protects the cells from ROS.Citation15 Vitamin C scavenges the ROS by very rapid electron transfer that, thus, inhibits lipid peroxidation.Citation16 Melatonin is synthesized by the pineal gland, retina, gastrointestinal tract, and so on and is secreted by the pineal gland. In vivo and in vitro, melatonin has been known as a radical scavenger with the ability to remove ROS and reactive nitrogen species.Citation17 Regarding the protective effect of melatonin on renal alterations various studies have been carried out and it has been found to protect tissues against oxidative damage generated by a variety of toxic agents.Citation18
Nitric oxide (NO) is a free radical gaseous molecule with a biological half-life of a few seconds. NO is generated from the guanidine nitrogen of l-arginine by three isoforms of nitric oxide synthase (NOS), that is, neuronal NOS (nNOS, NOS-1), endothelial NOS (eNOS, NOS-3), and inducible NOS (iNOS, NOS-2). NO acts as an intra- and intercellular messenger in different tissues.Citation19 In the kidney, NO acts as a signaling molecule and is generated by mesangial cells and renal tubular epithelial cells.Citation20 Under physiological conditions, NO plays a prominent role in the regulation of systemic and glomerular and medullar hemodynamics, tubular function (tubular fluid and electrolyte transport), tubuloglomerular feedback, and renin release.Citation21
Oxidative stress and endothelial dysfunction, which are inter-related, play a role in many diseases, including several nephropathies. Thus, in this article we report the consequences of long-term ethanol administration in rat kidney on the level of the free radical scavenger and antioxidant enzyme activities, including superoxide dismutase (SOD) and CAT. In addition, the content of lipid peroxidation product, malondialdehyde (MDA), and the expression of eNOS were examined.
MATERIALS AND METHODS
Animals
The study was conducted in Erciyes University Hakan Çetinsaya Experimental and Clinic Research Center. Ethical approval for study was obtained from Erciyes University Animal Researches Local Ethics Committee and all procedures conformed to the “Guide for the Care and Use of Laboratory Animals.” A total of 24 adult male Wistar rats, 200–250 g in weight at the beginning of the experiments, were used. They were housed in a quiet and temperature- and humidity-controlled room (21 ± 3°C and 60 ± 5%, respectively) in which a 12 h light:12 h dark cycle was maintained (07:00–19:00 h light). Animals were randomly separated into four groups of six rats each: Group I served as control and received liquid diet not containing ethanol. Group II was the ethanol treatment group: rats were fed with alcohol-containing liquid diet for 28 days. Group III received alcohol-containing liquid diet and 40 mg/kg/day ascorbic acid (Redoxon, Bayer AG, Leverkusen, Germany) were injected intraperitoneally. Group IV received alcohol-containing liquid diet and 4 mg/kg/day melatonin (Merck, cat. no. 814537, Darmstadt, Germany) was injected intraperitoneally.Citation22
Chronic Ethanol Treatment
For chronic ethanol exposure, the rats were housed individually and ethanol was given in the modified liquid diet as previously described.Citation23 At the beginning of the study, modified liquid diet without ethanol was given to rats for 7 days. Then liquid diet with 2.4% ethanol was administered for 3 days. The ethanol concentration was increased to 4.8% and 7.2% for the following 4 and 21 days on a liquid diet, respectively. Control rats were pair fed with an isocaloric liquid diet not containing ethanol. Liquid diet was freshly prepared daily and presented at the same time of the day (10:00 h). Animals were weighted and recorded everyday and ethanol intake was also measured and expressed as g/kg. The animals were not given access to water in addition to liquid diet with or without ethanol. The daily ethanol consumption of the rats ranged from 11.3 to 15.1 g/kg.
At the end of the experimental period, animals were killed by decapitation under intraperitoneal ketamine (75 mg/kg) + xylazine (10 mg/kg) anesthesia. After decapitation, kidney tissues were quickly removed. Some of the kidney tissues were used for biochemical analyses and the other tissues were used at histological procedures.
Biochemical Analysis of Kidney Tissues
For biochemical analysis, the tissues were weighed and homogenized in four volumes of ice-cold Tris-HCl buffer (50 mM, pH 7.4) containing 0.50 mL/L Triton X-100 with a homogenizer (IKA Ultra-Turrax T 25 Basic, IKA, Staufen, Germany) for 2 min at 13,000 rpm. All procedures were performed at +4°C. Tissue homogenates were then centrifuged at 5000 × g for 60 min to remove debris. Clear supernatant fluids were separated and kept at −40°C until the enzyme activity measurements were performed.
Determination of MDA Level
The MDA level was determined with the method modified by Ohkawa et al.Citation24 The MDA is the end product of fatty acid peroxidation and reacts with thiobarbituric acid to form a pink complex. The color was determined at 532 nm.
Determination of SOD Activity
Total (Cu–Zn and Mn) SOD (EC 1.15.1.1) activity was determined based on the method of Sun et al.Citation25 The principle of the method is based on the inhibition of nitro blue tetrazolium (NBT) reduction by the xanthine–xanthine oxidase system as a superoxide generator. Activity was assessed in the ethanol phase of the supernatant after addition of 1 mL of ethanol–chloroform mixture (5:3, v/v) to the same volume of sample and centrifuged. Total SOD activity was measured after incubation for 20 min at 25°C spectrophotometrically at 560 nm. The SOD (1U) was defined as the amount of enzyme causing 50% inhibition in the NBT reduction rate. The SOD activity was expressed as U/g protein.
Determination of CAT Activity
The CAT activity was determined based on the reaction of the enzyme with methanol in the presence of an optimal concentration of H2O2. The CAT activity was expressed as nmol/min/mL.
Histological Procedures
Parts of the tissues were fixed in 10% formalin for 24 h and rinsed under running tap water for 24 h, followed by dehydration through a graded alcohol series. Tissues were made transparent in xylol and embedded in paraffin. Sections of 5 μm thickness were stained with hematoxylin–eosin (H&E) and Masson’s trichrome and photographs were taken with an Olympus BX-51 photomicroscope (Olympus, Tokyo, Japan).
Immunohistochemistry
The expression of eNOS was detected immunohistochemically in the kidney using a rabbit polyclonal antibody (sc.654; Santa Cruz Biotechnology, Santa Cruz, CA, USA) and the streptavidin–biotin peroxidase technique. The procedure was performed under identical conditions for all sections. Paraffin sections (5 μm) were dewaxed in xylene. The sections were rehydrated, rinsed in deionized water, and subjected to 2N HCl solution for 20 min to antigen retrieval. Endogenous peroxidase activity was inhibited in 3% H2O2 in methanol for 10 min. The specimens were washed in phosphate-buffered saline (PBS) three times (5 min each time) and preincubated in a 1.5% normal goat serum (NGS; sc.2043, Santa Cruz Biotechnology) in PBS for 20 min at room temperature in a humidified chamber. Then, the sections were incubated overnight at 4°C with eNOS antibody (2 μg/mL diluted in PBS with 1.5% NGS). Negative control sections were done by replacing the eNOS antibody by PBS. The sections were then incubated with biotinylated goat anti-rabbit IgG (1 μg/mL diluted in PBS with 1.5% NGS) (sc.2040; Santa Cruz Biotechnology) for 30 min at room temperature in a humidified chamber, followed by streptavidin horseradish peroxidase conjugate (ready-to-use) (catalog no: 50–420Z; Zymed Laboratories Inc., South San Francisco, CA, USA) for 30 min and AEC (red) substrate kit (catalog no: 00–2007; Zymed Laboratories) for 5 min. Finally the sections were counterstained with hematoxylin, rinsed in deionized water, and mounted with clearmount solution (ready-to-use) (catalog no: 00–8010; Zymed Laboratories). The sections were examined and photographed under a light microscope (Bx-51).
Immunohistochemical eNOS-staining intensity in the kidney tissues of all groups was evaluated semiquantitatively by two independent histologists in a blind fashion. The intensity of eNOS expression was scored as follows: no staining (−), low (+), moderate (++), and strong (+++).
Statistical Analysis
All statistical analyses were performed with the Statistical Package for Social Sciences software package (SPSS for Windows 11.5; SPSS, Chicago, IL, USA). The data were expressed as mean ± standard deviation (SD). The data were statistically analyzed using one-way analysis of variance (ANOVA). Statistical significance was set at p < 0.05.
RESULTS
No statistically significant differences were determined in body weight between groups. Light microscopic examinations exhibited normal renal corpuscles and tubules in the control group (A). Collagen staining of the glomerulus was more pronounced (B) in rats in Group II compared with the controls. Atrophic renal corpuscles in some of fields, dilatation and congestion of the peritubular vessels (C), renal corpuscles with obscure Bowman’s space, and a few foamy-appearing tubules (D) due to alcohol consumption were observed. Distinctive collagen staining in the glomerulus and persistence of dilatation and congestion of the peritubular vessels could be seen even when melatonin (A) or vitamin C (B) was given to alcohol-administered rats. eNOS expression was seen in all groups. In control group immune staining was localized to glomerulus (+), especially more evident in distal and collector tubule (++) (C). Decreasing eNOS staining was determined in alcohol treatment group (D). Melatonin (A and B) and vitamin C (C) encore increased expression pattern of eNOS. Negative controls where incubation with the primary antisera was omitted were completely unlabelled, as seen in D.
Figure 1. (A) Group I—Glomerulus, Bowman’s space, and proximal and distal tubular structures can be differentiated (H&E). (B) Group II—Prominence collagen (arrow) staining compared with the control group can be observed in the glomerulus and peritubular region (Masson’s Trichome). (C) Group II—Dilatation and congestion of the vessels (arrow) due to alcohol consumption can be seen (H&E). (D) Renal corpuscle with obscure Bowman’s space (arrow) and foamy-looking tubules (*) can be observed in alcohol consumption rats (H&E).
Note: H&E, hematoxylin–eosin.
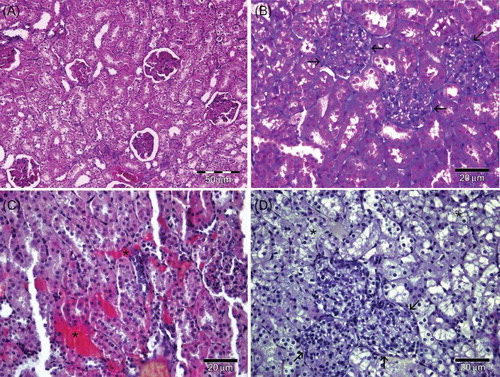
Figure 2. (A) Group IV—Dilatation and congestion of the peritubular vessels (*) and prominent collagen (arrow) staining can be seen (Masson’s Trichome). (B) Group III—Dilatation and congestion peritubular vessels (*) and increased collagen (arrow) staining compared to control group can be seen in administrated vitamin C (Masson’s Trichome). (C) Group I—The presence of eNOS in the glomerulus and tubules can be observed. (D) Group II—The eNOS immunoreactivity is lesser expressed compared with the control.
Note: eNOS, endothelial nitric oxide synthase.
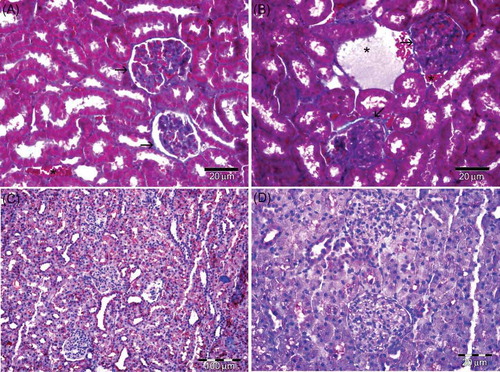
Figure 3. (A) Group IV—eNOS immunoreactivity is observed near the control in melatonin treatment group. (B) Group IV—eNOS immunoreactivity localized especially collector tubules in medulla. (C) Group III—eNOS immunoreactivity is observed near the control in administrated vitamin C. (D) Group II—Negative control.
Note: eNOS, endothelial nitric oxide synthase.
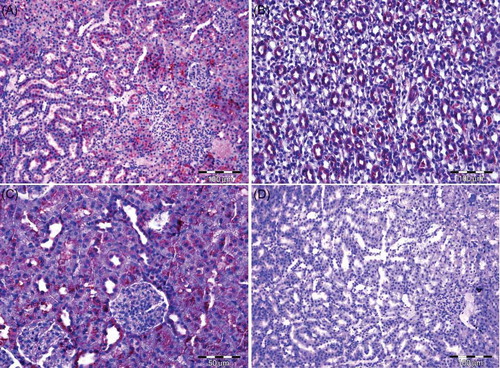
Biochemical analyses revealed that alcohol consumption increased MDA levels and SOD and CAT activities significantly in the alcohol consumption groups compared with the control group, while melatonin statistically significantly decreased MDA levels and SOD and CAT activities compared with alcohol consumption groups ().
Table 1. MDA and SOD levels and CAT activity in the kidney.
DISCUSSION
Oxidative stress is defined as imbalance between production of ROS and antioxidant pathways. It has long been known that ROS are harmful for cells, because they injure lipids, proteins, and nucleic acids, which leads to structural and functional impairments.Citation26,27 Oxidative stress is included in the pathophysiology of many renal diseases, involving acute renal failure and chronic renal disease. ROS cause glomerular and tubulointerstitial damage by activating and maintaining cytokine release, leukocyte infiltration, fibrosis, and mesangial and endothelial cell apoptosis.Citation28 Ethanol is used to generate oxygen radicals, inhibit glutathione (GSH) synthesis, deplete GSH levels in tissues, increase MDA levels, and generally impair the antioxidative defense system in humans and experimental animals. Chronic ethanol ingestion accounts for the formation of ROS and can induce a decrease of antioxidant defense.Citation29,30 The liver is the primary organ responsible for the oxidation of ingested ethanol. However, kidney may contribute to ethanol metabolism.Citation31 Experimental studies on animal models have also indicated that ethanol enhanced the fatty acid oxidation by kidney microsomes and peroxisomes and affected the activities of some kidney lysosomal hydrolases.Citation32
It is widely accepted that alcohol consumption causes disruption in the structure and function of the kidneys. White et al.Citation33 demonstrated that, in Australian adults, self-categorization as a moderate or heavy drinker or an average consumption of 30 g of ethanol per day or more was associated with significantly elevated risks of developing albuminuria over 5 years of follow-up, compared with light alcohol consumption. Kronborg et al.Citation34 reported that, among males, consumption of seven or more units of alcohol per week was associated with a significant increase in estimated glomerular filtration rate (eGFR) over 7 years of follow-up. Assadi et al.Citation35 reported that ethanol treatment rats showed cytoplasmic mitochondrial atrophy and vacuolar structures of the epithelial cells of the distal tubules and collecting ducts. In another study, the dilated tubules with cloudy swelling were shown in the kidney samples of alcohol-administered rats.Citation36 In this study, light microscopic examination of the renal tissue of rats subjected to alcohol consumption revealed atrophic renal corpuscles in some of fields and renal corpuscles with obscure Bowman’s space. In addition, dilatation and congestion of the peritubular vessels and a few foamy-appearing tubules could be seen. These findings clearly indicate alcoholic damage in kidney.
Enzymatic and nonenzymatic systems protect the oxidant/antioxidant status, but they are overcome during oxidative stress, a metabolic disorder due to an instability caused by excessive generation of ROS or a diminished capacity of the antioxidant defense system. Alcohol consumption results in excessive generation of free radicals, which change the biomembranes and cause damage. To scavenge ROS, cells have several antioxidant enzymes including CAT, GSH peroxidase (GPO), and SOD.Citation36 Adaramoye et al.Citation37 demonstrated that in ethanol-treated rats, serum urea, creatinine, urinary glucose, gamma-glutamyltransferase, and protein levels were increased, while creatinine clearance significantly decreased.They also found that serum and kidney MDA levels were increased, and CAT, SOD, and GSH activities were decreased in the kidney of ethanol-treated rats. Pari et al.Citation36 reported significant decreases in the enzymatic activities of some antioxidants in alcohol-treated rats compared with that in controls. In this study, biochemical analyses of renal tissue of rats subjected to alcohol consumption revealed increased MDA level and SOD and CAT activities significantly compared with that of the control group. The biochemical findings were corroborated by histopathological examination of the kidney.
In this study, melatonin and vitamin C were used as protective agents against alcohol-induced toxicity. Besides acting as a direct scavenger of free radicals, melatonin has indirect antioxidant actions. Melatonin has been shown to support several intracellular enzymatic antioxidant enzymes, including SOD, GSH reductase, CAT, glucose-6-phosphate dehydrogenase, and GSH peroxidase.Citation38–40 Yurtcu et al.Citation41 demonstrated that melatonin is a potent antioxidant agent in preventing testicular ischemia–reperfusion (I–R) injury. Ilbey et al.Citation18 found that melatonin treatment inhibited the increase in lipid peroxidation in acetaminophen-induced nephrotoxicity. In another study, Abraham et al.Citation42 reported that melatonin pretreatment reduced methotrexate-induced oxidative stress and alteration in the activity of antioxidant enzymes. Previously, we reported in our laboratory that melatonin decreased MDA and GSH levels in the kidney of smoke-inhaled rats.Citation22 In this study, melatonin statistically significantly decreased MDA levels and SOD and CAT activities compared with alcohol consumption groups. These findings agree with the previous studies. A number of studies have shown that melatonin is better than the classic antioxidants in resisting free-radical-based molecular destruction. In this study, we also showed that melatonin was more effective than vitamin C.
NO plays important roles in the control of renal and glomerular hemodynamics, tubuloglomerular feedback response, release of renin and sympathetic transmitters, tubular ion transport, and renal water and sodium excretion.Citation43 Alteration of renal NO production has been associated with glomerular hyperfiltration, vascular permeability, albuminuria, glomerulosclerosis, and tubulointerstitial fibrosis.Citation44 All three isoforms of NOS, namely, nNOS, iNOS, and eNOS, are reported to contribute to NO synthesis in the kidney. It was determined that eNOS is strongly expressed in renal vascular endothelium and tubular epithelium.Citation45 In this study, eNOS immune staining was localized to glomerulus and tubules. We also found decreasing eNOS staining in alcohol treatment group compared with that in control. Increased ROS activity can enhance NO inactivation and reduce bioactivity of NO. This can cause a compensatory upregulation of NOS isotype expression. Therefore, Satoh et al.Citation46 found that the expression of eNOS mRNA was higher in diabetic glomeruli, which is an oxidative condition. On the other hand, Majhi et al.Citation47 reported that arsenic exposure for an extended period can reduce eNOS expression and activity in rat kidney. It appeared that in previous findings there were contradictions. These differences may be due to experimental procedure and/or linking diverse pathogenic mechanism of oxidative stress. Being administrated to protective effect melatonin and vitamin C encore increased the expression pattern of eNOS.
The results of biochemical tests together with histological observations suggest that alcohol leads to serious changes in the histology of kidney. The increased formation of lipid peroxides and associated ROS leads to damage in membrane integrity and other pathological changes in kidney. The efficacy of any protective drug is essentially dependent on its capacity of either reducing the harmful effects or maintaining the normal physiology of cells and tissues. The membrane protective properties and antioxidant nature of melatonin might be helpful to alleviate the pathological changes caused by alcohol in kidney.
ACKNOWLEDGMENT
The authors thank Dr. Eser KILIÇ technical support.
Declaration of interest: The authors report no conflicts of interest. The authors alone are responsible for the content and writing of the paper.
REFERENCES
- You M, Crabb DW. Recent advances in alcoholic liver disease II. Minireview: Molecular mechanisms of alcoholic fatty liver. Am J Physiol Gastrointest Liver Physiol. 2004;6:1–6.
- Arteel GE, Iimuro Y, Yin M, Raleigh JA, Thurman RG. Chronic enteral ethanol treatment causes hypoxia in rat liver tissue in vivo. Hepatology. 1997;25:920–926.
- Lieber CS. Alcoholic liver disease: New insight in pathogenesis leads to new treatment. J Hepatol. 2000;32(1):113–128.
- Lieber CS. Relationships between nutrition, alcohol use, and liver disease. Alcohol Res Health. 2003;27:220–231.
- Nechifor M, Dinu D, Dumitru IF, . Chronic ethanol consumption affects the activity of antioxidant enzymes in the rat liver. Rom J Physiol. 2001;38:127–134.
- Assadi FK, Zajac CS. Ultrastructural changes in the rat kidney following fetal exposure to ethanol. Alcohol. 1992;9:509–512.
- Rodrigo R, Thielemann L, Olea M, Munoz P, Cereceda M, Orellana M. Effect of ethanol ingestion on renal regulation of water and electrolytes. Arch Med Res. 1998;29:209–218.
- Brzoska MM, Moniuszko-Jakonink J, Pilat-Marcinkieewicz B, Sawicki B. Liver and kidney function and histology in rats exposed to cadmium and ethanol. Alcohol Alcohol. 2003;38:2–10.
- Corrao G, Rubbiati L, Bagnardi V, . Alcohol and coronary heart disease: A meta-analysis. Addiction. 2000;95:1505–1523.
- Parekh RS, Klag MJ. Alcohol: Role in the development of hypertension and end-stage renal disease. Curr Opin Nephrol Hypertens. 2001;10:385–390.
- Sies H. Oxidative stress: Introduction. In: Sies H, ed. Oxidative Stress: Oxidants and Antioxidants. San Diego, CA: Academic Press; 1991:XV–XXII.
- Scott RB, Reddy KS, Husain K, Schlorff EC, Rybak LP, Somani SM. Dose response of ethanol on antioxidant defense system of liver, lung, and kidney in rat. Pathophysiology. 2000;7:25–32.
- Rodrigo R, Trujillio MD, Bosco C, Orellana M, Thielmann L, Araya J. Changes in (Na+K)-adenosine triphosphatase activity and ultrastructure of lung and kidney associated with oxidative stress induced by acute ethanol intoxication. Chest. 2002;121:589–596.
- Sun AY, Ingelman-Sundberg M, Neve E, . Ethanol and oxidative stress. Alcohol Clin Exp Res. 2001;25(5):237–243.
- Jones DP, Kagan VE, Aust SD, Reed DJ, Omaye ST. Impact of nutrients on cellular lipid peroxidation and antioxidant defense system. Fundam Appl Toxicol. 1995;26:1–7.
- Patra RC, Swarup D, Dwivedi SK. Antioxidant effects of alpha tocopherol, ascorbic acid and L-methionine on lead induced oxidative stress to the liver, kidney and brain in rats. Toxicology. 2001;162(2):81–88.
- Mahieu S, Contini Mdel C, González M, Millen N. Melatonin reduces oxidative damage induced by aluminium in rat kidney. Toxicol Lett. 2009;190(1):9–15.
- Ilbey YO, Ozbek E, Cekmen M, . Melatonin prevents acetaminophen-induced nephrotoxicity in rats. Int Urol Nephrol. 2009;41(3):695–702.
- Toda N, Imamura T, Okamura T. Alteration of nitric oxide-mediated blood flow regulation in diabetes mellitus. Pharmacol Ther. 2010;127(3):189–209.
- Trachtman H, Jacques C, Citron J, Futterweit S. Effect of triiodothyronine on nitric oxide production in mesangial cells and renal tubular epithelial cells. Res Commun Mol Pathol Pharmacol. 1996;93:69–78.
- Kone BC, Baylis C. Biosynthesis and homeostatic roles of nitric oxide in the normal kidney. Am J Physiol. 1997;272:561–578.
- Ozan E, Sonmez MF, Ozan S, Colakoglu N, Yilmaz S, Kuloglu T. Effects of melatonin and vitamin C on cigarette smoke-induced damage in the kidney. Toxicol Ind Health. 2007;23(8):479–485.
- Uzbay IT, Kayaalp SO. A modified liquid diet of chronic ethanol administration: Validation by ethanol withdrawal syndrome in rats. Pharmacol Res. 1995;31(1):37–42.
- Ohkawa H, Ohishi N, Yagi K. Assay for lipid peroxides in animal tissues by thiobarbituric acid reaction. Anal Biochem. 1979;95(2):351–358.
- Sun Y, Oberley LW, Li Y. A simple method for clinical assay of superoxide dismutase. Clin Chem. 1988;34(3):497–500.
- Freeman BA, Crapo JD. Biology of disease: Free radical and tissue injury. Lab Invest. 1982;47:412–426.
- Mantle D, Preedy VR. Free radicals as mediators of alcohol toxicity. Adverse Drug React. 1999;18:235–252.
- Presti RL, Carollo C, Caimi G. Wine consumption and renal diseases: New perspectives. Nutrition. 2007;23(7–8):598–602.
- Oh SI, Kim CI, Chun HL, Park SC. Chronic ethanol consumption affects glutathione status in rat liver. J Nutr. 1997;128:758–763.
- Lieber CS. Biochemical and molecular basis of alcohol induced injury to liver and others tissues. N Engl J Med. 1988;319(25):1639–1650.
- Orellana M, Vald´es E, Fernandez J, Rodrigo R. Effects of chronic ethanol consumption on extramitochondrial fatty acid oxidation and ethanol metabolism by rat kidney. Gen Pharmacol. 1998;30:719–723.
- Witek B, Kolataj A. Effect of ethanol administration on activities of some lysosomal hydrolases in the mouse. Gen Pharmacol. 1999;32:163–168.
- White SL, Polkinghorne KR, Cass A, Shaw JE, Atkins RC, Chadban SJ. Alcohol consumption and 5-year onset of chronic kidney disease: The AusDiab study. Nephrol Dial Transplant. 2009;24(8):2464–2472.
- Kronborg J, Solbu M, Njolstad I, . Predictors of change in estimated GFR: A population-based 7-year follow-up from the Tromso study. Nephrol Dial Transplant. 2008;23:2818–2826.
- Assadi FK, Zajac CS. Ultrastructural changes in the rat kidney following fetal exposure to ethanol. Alcohol. 1992;9(6):509–512.
- Pari L, Suresh A. Effect of grape (Vitis vinifera L.) leaf extract on alcohol induced oxidative stress in rats. Food Chem Toxicol. 2008;46(5):1627–1634.
- Adaramoye OA, Aluko A. Methanolic extract of Cnidoscolus aconitifolius attenuates renal dysfunction induced by chronic ethanol administration in Wistar rats. Alcohol Alcohol. 2011;46(1):4–9.
- Swiderska-kolacz G, Klusek A. The effect of melatonin on glutathione and glutathione transferase and glutathione peroxidase activities in the mouse liver and kidney in vivo. Neuro Endocrinol Lett. 2006;27:365–368.
- Tomas-Zapico C, Coto-Montes A. A proposed mechanism to explain the stimulatory effect of melatonin on antioxidative enzymes. J Pineal Res. 2005;39:99–104.
- Sener G, Tugtepe H, Velioglu-Ogunc A. Melatonin prevents neutrophil mediated oxidative injury in Escherichia Coli induced pyelonephritis in rats. J Pineal Res. 2006;41:220–227.
- Yurtçu M, Abasiyanik A, Avunduk MC, Muhtaroğlu S. Effects of melatonin on spermatogenesis and testicular ischemia-reperfusion injury after unilateral testicular torsion-detorsion. J Pediatr Surg. 2008;43(10):1873–1878.
- Abraham P, Kolli VK, Rabi S. Melatonin attenuates methotrexate-induced oxidative stress and renal damage in rats. Cell Biochem Funct. 2010;28(5):426–433.
- Zou AP, Cowley Jr AW. Role of nitric oxide in the control of renal function and salt sensitivity. Curr Hypertens Rep. 1999;1(2):178–186.
- Hohenstein B, Hugo CP, Hausknecht B, Boehmer KP, Riess RH, Schmieder RE. Analysis of NO-synthase expression and clinical risk factors in human diabetic nephropathy. Nephrol Dial Transplant. 2008;23(4):1346–1354.
- Mount PF, Power DA. Nitric oxide in the kidney: Functions and regulation of synthesis. Acta Physiol (Oxf). 2006;187(4):433–446.
- Satoh M, Fujimoto S, Haruna Y, . NAD(P)H oxidase and uncoupled nitric oxide synthase are major sources of glomerular superoxide in rats with experimental diabetic nephropathy. Am J Physiol Renal Physiol. 2005;288:F1144–F1152.
- Majhi CR, Khan S, Leo MD, Manimaran A, Sankar P, Sarkar SN. Effects of acetaminophen on reactive oxygen species and nitric oxide redox signaling in kidney of arsenic-exposed rats. Food Chem Toxicol. 2011;49(4):974–982.