Abstract
The exposure to hexavalent chromium is often known to cause acute renal failure. It has been found that nonenzymatic antioxidants and the induction of heme oxygenase 1 have protective effects against nephrotoxicity induced by potassium dichromate in vivo. In this work, the effect of stannous chloride, an inducer of heme oxygenase 1, on potassium dichromate-induced toxicity in proximal tubular epithelial cells was studied. Hexavalent chromium levels, peroxynitrite content, reduced thiol content, heme oxygenase activity, reactive oxygen species production, and stannous chloride scavenging capacity were measured. It was found that stannous chloride protects proximal tubular epithelial cells from potassium dichromate-induced cell death. The decrease in extracellular and intracellular hexavalent chromium concentration, the induction of heme oxygenase 1, and the ability to scavenge reactive oxygen species and peroxynitrite are involved in the mechanism by which stannous chloride protects proximal tubular epithelial cells from potassium dichromate-induced toxicity.
INTRODUCTION
Hexavalent chromium [Cr(VI)] is an environmental pollutant that is generated by chrome plating, stainless steel, and other industrial processes. Cr(VI) is known to cause allergic dermatitis, carcinogenicity, and acute renal failure (ARF) in humans and in animals.Citation1,2 Reactive intermediates and free radicals generated during reduction process might be responsible for Cr(VI) toxicity.Citation3,4 K2Cr2O7 induces oxidative stress and nitrosative stress.Citation2,5,6 Antioxidants prevent the K2Cr2O7-induced kidney damage in vivoCitation7–9 and in vitro.Citation10 On the other hand, stannous chloride (SnCl2) is a reducing chemical agent used in several man-made products (conserve soft drinks and some fluoride toothpastes). SnCl2 is a potent inductor of heme oxygenase-1 (HO-1) in acute expose in rat and rabbit,Citation2,5,11 but induce kidney damage in chronic exposure in rabbit.Citation12 Previous studies have documented induction of HO-1 in proximal tubuli in response to SnCl2 and the protective effect in K2Cr2O7-induced ARF in Wistar rats.Citation2,5 Therefore, in an attempt to go deeper in the understanding about the protection of SnCl2 of Cr(VI) toxicity, in this study proximal tubular epithelial LLC-PK1 cells were used. Hence, the effect of SnCl2 on K2Cr2O7-induced cell death, HO activity, cellular Cr(VI) accumulation, reduced thiol content, and superoxide anion (O2•−), hydrogen peroxide (H2O2), hydroxyl radical (OH•), and peroxynitrite (ONOO−) production in LLC-PK1 cells as well as the effect of K2Cr2O7 on reactive oxygen species (ROS) and Cr(VI) content in culture medium were determined. Furthermore, it was studied whether SnCl2 is able to scavenge O2•−, H2O2, OH•, and ONOO−.
RESEARCH DESIGN AND METHODS
Reagents
The porcine kidney tubular epithelium cell line (LLC-PK1) was obtained from the American Type Culture Collection (ATCC, Manassas, VA, USA). SnCl2, K2Cr2O7, hydrogen peroxide (H2O2), and glutaraldehyde were obtained from JT Baker (Xalostoc, Edo. México, México). Hemin, stannous (II) protoporphyrin IX (SnPP), and cobalt (II) protoporphyrin IX (CoPP) were obtained from Porphyrin Products Inc. (Logan, UT, USA). Dihydrorhodamine 123 (DHR 123) was purchased from Cayman Chemical Co. (Ann Arbor, MI, USA). N-acetyl cysteine (NAC), glutathione (GSH), crystal violet, Tris-HCl, ethylenediaminetetraacetic acid (EDTA), Triton X-100, nitroblue tetrazolium (NBT), butylated hydroxytoluene, xylenol orange, ammonium ferrous sulfate, deoxyribose, ascorbic acid, glucose-6-phosphate, glucose-6-phosphate dehydrogenase, reduced form of nicotinamide adenine dinucleotide phosphate (NADPH), diphenyl carbazide (DFC), xanthine oxidase (XO), FeCl3, xanthine, sodium pyruvate ebselen, dimethyl thiourea (DMTU), tempol, and 5,5′-dithio-bis-(2-nitrobenzoic acid) (DTNB) were obtained from Sigma-Aldrich (St. Louis, MO, USA). Dulbecco’s modified Eagle medium (DMEM), fetal bovine serum (FBS), penicillin, and streptomycin were obtained from Gibco, Invitrogen Corporation (Grand Island, NY, USA).
Cell Culture
LLC-PK1 cells were cultured in 10-cm dishes with DMEM, supplemented with 10% FBS, 100 units/mL penicillin, and 100 μg/mL streptomycin at 37°C under 5% CO2 humidified atmosphere. The cells were plated in 96-well plates or 6-cm dishes and incubated for 24 h to confluence, and the experimental measurements were performed.
Study Design
To evaluate the protective effect of SnCl2 on the K2Cr2O7-induced toxicity, DMEM without FBS was used. Seven groups of conditions were studied (n = 3 per group, the experiments were performed three times at different days): (i) control cells, incubated with medium; (ii) K2Cr2O7-treated cells; (iii) K2Cr2O7- and SnCl2-treated cells; (iv) K2Cr2O7-, SnCl2-, and SnPP-treated cells; (v) K2Cr2O7- and SnPP-treated cells; (vi) SnCl2-treated cells; and (vii) SnPP-treated cells. Concentrations used in all different conditions were 30 μM K2Cr2O7, 200 μM SnCl2, and 40 μM SnPP. The cells treated with SnCl2 and/or SnPP were incubated for 1 h before the addition of K2Cr2O7. The cell groups were further incubated for 24 h with K2Cr2O7, and the viability and HO activity were evaluated. Light microscopy micrographs were also taken.
To evaluate the protective effect of antioxidants on K2Cr2O7-induced toxicity, DMEM without phenol red and FBS was used. The cells were incubated for 24 h with K2Cr2O7 and SnCl2 or K2Cr2O7 and with an antioxidant (10 μM NAC or 20 μM GSH), and the experimental measurements were performed. Cell viability, Cr(VI), ONOO−, reduced thiol content, O2•−, H2O2, and OH• in cells, and H2O2 in the culture medium were measured. A control group was always included for the studied conditions.
Cell viability measurement
For the determination of cell viability, two methods were carried out; the crystal violet assay is based on the inability of dead cells to remain adherent to cell culture plastic, as previously described.Citation13 An index of cellular injury was established based on the leakage of lactate dehydrogenase (LDH) from the renal cells into the culture medium. The activity of LDH released from the cells treated with K2Cr2O7 was measured using the supernatant obtained after centrifuging the culture medium. LDH activity was determined using a commercial kit (Spinreact, Spain).
A close correlation between both methods (crystal violet and LDH release) for the range of K2Cr2O7 concentrations of 0–100 μM (r = 0.9993, data not shown) was found. Based on the above information, only the crystal violet method was used to measure cell viability.
ROS and ONOO− determination
To evaluate ROS or ONOO− production in our biological model, the culture medium was removed; the cells were washed with 50 mM potassium phosphate buffer, pH 7.0, and briefly sonicated in the same buffer. The cell lysates or the culture medium samples were mixed with the corresponding reaction mixture. The ROS scavenging assays were modified to evaluate ROS production only to measure ROS.
ROS and ONOO− scavenging activity
Different SnCl2 solutions (0–200 μM) were evaluated for O2•−, H2O2, OH•, and ONOO− scavenging activity. Scavenging capacity was expressed as % and the inhibitory concentration (IC50) value is the concentration of SnCl2 that is able to scavenge or inhibit 50% of the ROS present or generated in each specific assay system.Citation10
Superoxide radical
Xanthine–xanthine oxidase system was used to determine the O2•− in the assay system, and xanthine oxidase activity was measured to evaluate NBT reduction (560 nm) and uric acid production (295 nm), respectively.Citation10,14 The specificity of the method was validated by adding the O2•− scavenger tempol (1 mM)Citation15 to cell lysates or culture medium. In fact, the NBT reduction was completely suppressed by tempol. Scavenging percent was obtained from the optical densities at 560 nm.
Concentrations of O2•− were determined using a molar extinction coefficient of 24,400 M−1 cm−1 at 560 nm.Citation16,17
Hydrogen peroxide
H2O2 was measured by the method described by Long et al.Citation18 (FOX reagent: butylated hydroxytoluene, xylenol orange, and ammonium ferrous.). The concentration of H2O2 was calculated from a standard curve prepared with increasing H2O2 concentrations.Citation10,14 To validate the specificity of the measurement, the H2O2 scavenger sodium pyruvate (1 mM)Citation19 was added to the standard curve, the cell lysates, and the culture medium. It was found that sodium pyruvate effectively suppressed H2O2 detection.
Hydroxyl radical
The OH• was generated with the H2O2–EDTA– FeCl3 system and was measured using the degradation of deoxyribose.Citation20 The degradation of deoxyribose was measured in terms of thiobarbituric acid reactive substances formation.Citation21 The degradation of deoxyribose was completely suppressed by the OH• scavenger DMTU (50 mM). The specificity of OH• measurement in cell lysates or culture medium was evaluated by the addition of this OH• scavenger.Citation22 The tubes were read at 532 nm against a standard curve of tetramethoxypropane.Citation21
Peroxynitrite
Peroxynitrite was synthesized as previously described.Citation23 Peroxynitrite scavenging activity was measured by monitoring the oxidation of DHR 123 by a modified method of Haddad et al.Citation10,14,24 The specificity of ONOO− measurement in cell lysates was evaluated by the addition of ebselen.Citation25 In fact, the DHR oxidation was completely suppressed by ebselen. Concentrations of ONOO− were determined using a molar extinction coefficient of 78,000 M−1 cm−1 at 500 nm.Citation24
Heme oxygenase enzyme activity
Cells microsomes were prepared and used for determination of enzyme activity as described.Citation2
Cr(VI) content
Cr(VI) content was determined with diphenyl carbazide (1 volume of 100 mg DFC in 95% ethanol and 1 volume of 10% H2SO4) in cell homogenates or culture medium (incubated with or without cells) at 540 nm. For calibration, a commercial 0.1% (w/v) Cr(VI) stock solution was used as standard. The reaction was linear up to 50 nmol Cr(VI).Citation26
Reduced thiol content
The cells were sonicated with 0.1 M phosphate buffer, pH 8. For determination of reduced thiol, cellular samples were mixed with 1 mM DTNB, and immediately the optical density at 412 nm was recorded (extinction coefficient of thiol compounds derivates by DTNB used was 13.2 mM−1cm−1).Citation26,27
Protein content
The protein content was determined according to Lowry, as previously described.Citation2
Statistical Analysis
Results are expressed as mean ± SE. Data were analyzed by one-way analysis of variance (ANOVA) followed by Dunnett or Bonferroni multiple comparisons post-test as appropriate using the software Prism 3 (GraphPad, San Diego, CA, USA). p-Value <0.05 was considered significant.
RESULTS
Effect of SnCl2 on K2Cr2O7-Induced Toxicity on LLC-PK1 Cells
Previous in vivo studies had been shown that K2Cr2O7 induced necrosis in 50% of tubuli for 24 h after its administration [2]. In vitro studies found that incubation of LLC-PK1 cells for 24 h with 30 μM K2Cr2O7 induced 50% of death. Therefore, this concentration was used to perform this study.
LLC-PK1 cells were incubated for 24 h with 30 μM K2Cr2O7 and 200 μM SnCl2. SnCl2 prevented K2Cr2O7-induced cell death ( and B and D). SnCl2-induced HO-1 activity which was blocked by SnPP, an inhibitor of the HO activity (HO-1 and HO-2) (). SnPP was able to partially block the protective effect induced by SnCl2 (), suggesting that HO-1 is involved, at least in part, in the protective effect against Cr(VI). We evaluated the potential protective effect of CoPP, a more specific HO-1 inductor. It was found that the protection was very small, and the addition of SnPP was able to partially block this small protective effect (data not shown).
Figure 1. Effect of stannous chloride (SnCl2) on potassium dichromate (K2Cr2O7)-induced toxicity and on HO activity in LLC-PK1 cells. K2Cr2O7 (30 μM), SnCl2 (200 μM), and stannous protoporphyrin (SnPP, 40 μM) were added for 24 h as indicated above. At the end of this period, viability or HO activity were measured. Data are expressed as mean ± SE, n = 9. ap < 0.001 versus control, bp < 0.001 versus K2Cr2O7, cp < 0.001 versus K2Cr2O7 + SnCl2.
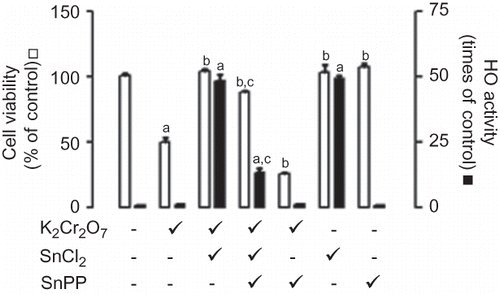
Figure 2. Effect of stannous chloride (SnCl2) on potassium dichromate (K2Cr2O7)-induced toxicity on LLC-PK1 cells. The cells were incubated without or with treatment for 24 h at the end of which light microscopy micrographs were taken (200×). (A) Control cells; (B) K2Cr2O7 (30 μM)-treated cells; (C) SnCl2 (200 μM)-treated cells; (D) K2Cr2O7- and SnCl2-treated cells.
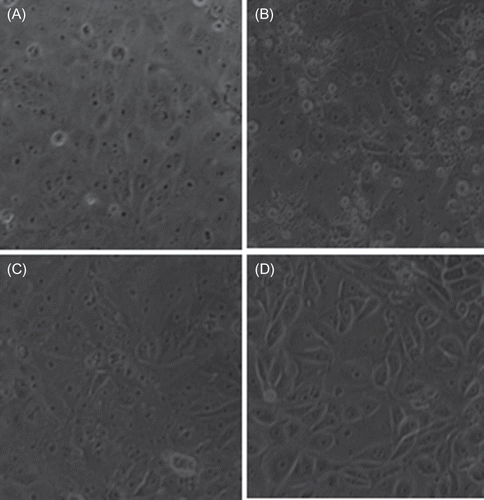
Effect of SnCl2 on the K2Cr2O7-Induced Changes in LLC-PK1 Cells
The data were compared with two well-known antioxidants (NAC and GSH). It was found that SnCl2 was able to prevent cell death, ONOO− formation, and the increase in reduced thiol content (A). In fact, the amount of reduced thiol is even lower when cells were co-incubated with K2Cr2O7 and SnCl2. SnCl2 was able to prevent the Cr(VI) entrance to the cells (A). However, the fact that SnCl2 decreases completely Cr(VI) levels in the culture medium incubated without or with cells (B) was a relevant finding. These protective effects were reproduced by NAC and GSH.
Figure 3. Comparative effect of stannous chloride (SnCl2, 200 μM), N-acetyl cysteine (NAC, 10 μM), and glutathione (GSH, 20 μM) on potassium dichromate (K2Cr2O7, 30 μM) induced changes on (A) cell viability, (A) peroxynitrite production, (A) reduced thiol content, (A) intracellular content of Cr(VI), (B) extracellular concentration of Cr(VI) in culture medium and (B) concentration of Cr(VI) in culture medium not exposed to cells. Data are expressed as mean ± SE, n = 9. ap < 0.001 versus control, bp < 0.001 versus K2Cr2O7.
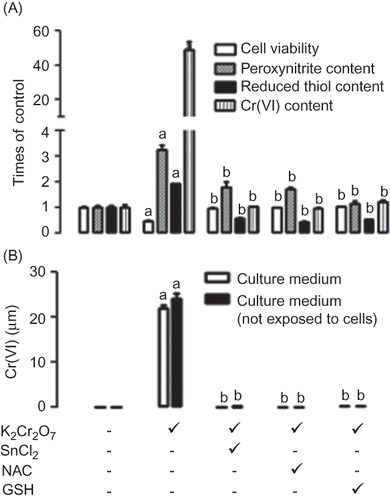
Furthermore, SnCl2 was able to prevent K2Cr2O7-induced O2•− and H2O2 production and to ameliorate OH• production (A) in LLC-PK1 cells. NAC was also able to prevent H2O2 and OH• formation but unable to prevent O2•− formation (A). GSH was able to prevent H2O2, O2•−, and OH• formation (A). SnCl2 ameliorates extracellular H2O2 formation, and NAC and GSH were able to completely prevent extracellular H2O2 formation (B). The protective effect of SnCl2 against extracellular H2O2 formation was evident even in the absence of cells (B).
Figure 4. Comparative effect of stannous chloride (SnCl2, 200 μM), N-acetyl cysteine (NAC, 10 μM), and glutathione (GSH, 20 μM) on potassium dichromate (K2Cr2O7, 30 μM) induced changes on (A) superoxide (O2•−) production, (A) hydrogen peroxide (H2O2) production, (A) hydroxyl radical (OH•) production, (B) extracellular hydrogen peroxide (H2O2) production, and (B) hydrogen peroxide (H2O2) production in culture medium not exposed to cells. Data are expressed as mean ± SE, n = 9. ap < 0.001 versus control, bp < 0.001 versus K2Cr2O7.
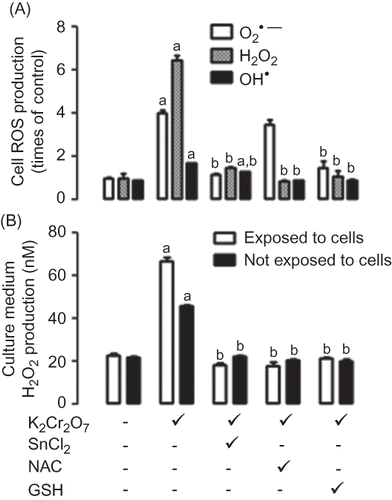
Scavenging Activity of SnCl2
SnCl2 was able to scavenge O2•−, H2O2, OH•, and ONOO− in a concentration-dependent way (). IC50 values of SnCl2 were the following: 2.19 μM for O2•−, 8.37 μM for H2O2, 9.08 μM for OH•, and 40.10 μM for ONOO−.
DISCUSSION
K2Cr2O7 was able to induce cell death, ROS, and ONOO− production, suggesting that LLC-PK1 cells may be a suitable model to study the mechanism by which cells may be protected against K2Cr2O7-induced toxicity. SnCl2 was able to protect LLC-PK1 cells against K2Cr2O7-induced cell death. In spite the fact that SnCl2 was able to increase HO activity, the inhibition of this enzyme with SnPP was unable to completely prevent the protection induced by SnCl2, suggesting that additional mechanisms of protection are involved in this particular cell type of porcine origin. The pre-induction of HO-1 prevents the kidney damage in rat.2,5 In contrast,Citation in this work was found that HO-1 induction is partially involved in the protective effect of SnCl2, which was confirmed using the specific HO-1 inductor CoPP. This may be due to the differences in both studies, that is, in vivo versus in vitro conditions and the species studied, that is, rat versus pig. In addition, it is possible that products of HO activity (such as biliverdin/bilirubin and carbon monoxide) generated in proximal tubuli in the in vivo study may exert beneficial effects in another cell types in kidney inducing recovery of glomerular function of rats.Citation2,5 In contrast, this type of cooperation is not possible in the cells in culture.
The K2Cr2O7-induced increase in intracellular ROS and ONOO− in LLC-PK1 is associated with the increase in the intracellular Cr(VI) content and its decrease in the culture medium. Furthermore, ROS increase is associated with the increase in reduced thiol content.
The protective effect of SnCl2 was associated with its ability to decrease extracellular and intracellular Cr(VI) concentrations as well as with the prevention of ROS and ONOO− production and in the increase in intracellular reduced thiol content. The prevention of intracellular Cr(VI) concentration is secondary, in all probability, to SnCl2-induced decrease in extracellular Cr(VI) concentration. We are tempting to speculate that SnCl2 reduces Cr(VI)-generating non-cell membrane permeant chromium species. In fact, it has been shown that reduced species of chromium such as Cr(III) are non-cell membrane permeant.Citation27,28 In addition, we are tempting to speculate that the preventive effect of SnCl2 on K2Cr2O7-induced ROS production may be secondary to its ability to decrease intracellular Cr(VI) content. SnCl2 was also able to prevent extracellular H2O2 production, which may also be involved in its protective effect. However, H2O2 generated in the culture medium in our assay conditions is not enough to induce cell death; in fact, damage to LLC-PK1 cells has been observed with 100-fold concentrations of H2O2.Citation29,30 In addition, for the first time, this work showed that the ROS scavenging ability of SnCl2 also may contribute to decrease ROS levels.
In addition, it was found that the antioxidants NAC and GSH induced similar effects to that observed with SnCl2.
In conclusion, the decrease in extracellular and intracellular Cr(VI) concentrations, the induction of HO-1, and the ability to scavenge ROS and ONOO− are involved in the mechanism by which SnCl2 protects LLC-PK1 cells from K2Cr2O7-induced toxicity.
Acknowledgments
The authors thank Dr. Ricardo Jasso-Chávez for providing advice with the technique for Cr(VI) and reduced thiol determinations and to Lilian A. Cruz for helping in some experiments.
Declaration of interest: The authors report no conflicts of interest. The authors alone are responsible for the content and writing of this article.
This work was supported by Programa de Apoyo a Proyectos de Investigación e Innovación Tecnológica de la Universidad Nacional Autónoma de México (PAPIIT-UNAM) IN205310 and IN201910 and Consejo Nacional de Ciencia y Tecnología (CONACYT) 47993-M.
REFERENCES
- Barceloux DG. Chromium. Clin Toxicol. 1999;37:173–194.
- Barrera D, Maldonado PD, Medina-Campos ON, Hernández-Pando R, Ibarra-Rubio ME, Pedraza-Chaverrí J. HO-1 induction attenuates renal damage and oxidative stress induced by K2Cr2O7. Free Radic Biol Med. 2003;34:1390–1398.
- Liu K, Shi X. In vivo reduction of chromium (VI) and its related free radical generation. Mol Cell Biochem. 2001;222:41–47.
- Stohs SJ, Bagchi D. Oxidative mechanisms in the toxicity of metal ions. Free Radic Biol Med. 1995;18:321–336.
- Barrera D, Maldonado PD, Medina-Campos ON, Hernández-Pando R, Ibarra-Rubio ME, Pedraza-Chaverrí J. Protective effect of SnCl2 on K2Cr2O7-induced nephrotoxicity in rats: the indispensability of HO-1 preinduction and lack of association with some antioxidant enzymes. Life Sci. 2003;73:3027–3041.
- Pedraza-Chaverrí J, Barrera D, Medina-Campos ON, . Time course study of oxidative and nitrosative stress and antioxidant enzymes in K2Cr2O7-induced nephrotoxicity. BMC Nephrol. 2005;6:4.
- Khan MR, Siddiqui S, Parveen K, Javed S, Diwakar S, Siddiqui WA. Nephro protective action of tocotrienol-rich fraction (TRF) from palm oil against potassium dichromate (K2Cr2O7)-induced acute renal injury in rats. Chem Biol Interact. 2010;186:228–238.
- Soudani N, Sefi M, Ben Amara I, Boudawara T, Zeghal N. Protective effects of selenium (Se) on chromium (VI) induced nephrotoxicity in adult rats. Ecotoxicol Environ Saf. 2010;73: 671–678.
- Yam-Canul P, Chirino YI, Sánchez-González DJ, . Nordihydroguaiaretic acid attenuates potassium dichromate-induced oxidative stress and nephrotoxicity. Food Chem Toxicol. 2008;46:1089–1096.
- Medina-Campos ON, Barrera D, Segoviano-Murillo S, . S-allylcysteine scavenges singlet oxygen and hypochlorous acid and protects LLC-PK(1) cells of potassium dichromate-induced toxicity. Food Chem Toxicol. 2007;45:2030–2039.
- Laniado-Schwartzman M, Abraham NG, Conners M, Dunn MW, Levere RD, Kappas A. Heme oxygenase induction with attenuation of experimentally induced corneal inflammation. Biochem Pharmacol. 1997;53:1069–1075.
- El-Demerdash FM, Yousef MI, Zoheir MA. Stannous chloride induces alterations in enzyme activities, lipid peroxidation and histopathology in male rabbit: antioxidant role of vitamin C. Food Chem Toxicol. 2005;43:1743–1752.
- Velasco-Velázquez MA, Maldonado PD, Barrera D, Torres V, Zentella-Dehesa A, Pedraza-Chaverrí J. Aged garlic extract induces proliferation and ameliorates gentamicin-induced toxicity in LLC-PK1 cells. Phytother Res. 2006;20: 76–78.
- Floriano-Sánchez E, Villanueva C, Medina-Campos ON, . Nordihydroguaiaretic acid is a potent in vitro scavenger of peroxynitrite, singlet oxygen, hydroxyl radical, superoxide anion, and hypochlorous acid and prevents in vivo tyrosine nitration in lung. Free Radic Res. 2006;40:523–533.
- Haj-Yehia AI, Nassar T, Assaf P, Nassar H, Anggård EE. Effects of the superoxide dismutase-mimic compound TEMPOL on oxidant stress-mediated endothelial dysfunction. Antioxid Redox Signal. 1999;1:221–232.
- Bielski BHJ, Shlue GG, Bajuk S. Reduction of nitro blue tetrazolium by CO2 and O2•− radicals. J Phys Chem. 1980; 84:830–833.
- Czerlinski GH, Anderson B, Tow J, Reid DS. Coupling of redox dyes into an enzymatic reaction cycle. J Biochem Biophys Methods. 1988;15:241–417.
- Long LH, Evans PJ, Halliwell B. Hydrogen peroxide in human urine: implications for antioxidant defense and redox regulation. Biochem Biophys Res Commun. 1999;262:605–609.
- Ramakrishnan N, Chen R, McClain DE, Bürger R. Pyruvate prevents hydrogen peroxide-induced apoptosis. Free Radic Res. 1998;29:283–295.
- Halliwell B, Gutteridge JM, Aruoma OI. The deoxyribose method: a simple “test-tube” assay for determination of rate constants for reactions of hydroxyl radicals. Anal Biochem. 1987;165:215–219.
- Okada Y, Okajima H, Shima Y, Ohta H. Hydroxyl radical scavenging action of capsaicin. Redox Rep. 2002;7:153–157.
- Fox RB. Prevention of granulocyte-mediated oxidant lung injury in rats by a hydroxyl radical scavenger, dimethylthiourea. J Clin Invest. 1984;74:1456–1464.
- Beckman JS, Chen H, Ischiropulos H, Crow JP. Oxidative chemistry of peroxynitrite. Meth Enzymol. 1994;233:229–240.
- Haddad IY, Crow JP, Hu P, Ye Y, Beckman J, Matalon S. Concurrent generation of nitric oxide and superoxide damages surfactant protein A. Am J Physiol. 1994;267:L242–L249.
- Arteel GE, Briviba K, Sies H. Protection against peroxynitrite. FEBS Lett. 1999;445:226–230.
- Jasso-Chávez R, Pacheco-Rosales A, Lira-Silva E, Gallardo-Pérez JC, García N, Moreno-Sánchez R. Toxic effects of Cr(VI) and Cr(III) on energy metabolism of heterotrophic Euglena gracilis. Aquat Toxicol. 2010;100:329–338.
- Cheng L, Sonntag DM, de Boer J, Dixon K. The role of chromium(V) in the mechanism of chromate-induced oxidative DNA damage and cancer. J Environ Pathol Toxicol Oncol. 2000;19:215–230.
- Chen W, Zhao Y, Seefeldt T, Guan X. Determination of thiols and disulfides via HPLC quantification of 5-thio-2-nitrobenzoic acid. J Pharm Biomed Anal. 2008;48:1375–1380.
- Reyes-Martin P, Alique M, Parra T, Hornedo JP, Lucio-Cazana J. Cyclooxygenase-independent inhibition of H2O2-induced cell death by S-ketoprofen in renal cells. Pharmacol Res. 2007; 5:295–302.
- Zhou X, Yang G, Davis CA, . Hydrogen peroxide mediates FK506-induced cytotoxicity in renal cells. Kidney Int. 2004;65:139–147.