Abstract
Objective: Recent studies have demonstrated the therapeutic effects of bone marrow–derived cells in tissue regeneration. The aim of this study was to investigate the effects of bone marrow mononuclear cell (BMMC) transplantation in a mouse model of acute renal failure (ARF) induced by mercuric chloride. Methods: BMMC was isolated from male BALB/c mice and injected into female mice treated with a lethal dose (LD90) of mercuric chloride. Survival rate, histopathological analysis, and assessment of urea, creatinine, sodium, potassium, and mercury levels were carried out. Results: Cellular therapy with BMMC significantly reduced the mortality induced by mercuric chloride (p < 0.05). This finding correlated with a decrease in serum levels of urea (p = 0.04) and potassium (p < 0.01). However, no differences in renal morphology were observed when BMMC-treated and control group were compared. Conclusion: Transplanted BMMC improve renal function and reduce mortality and, therefore, may represent a new therapeutic alternative to treat ARF.
INTRODUCTION
Acute kidney injury (AKI) is a common complication in hospitalized patients. Ischemic injury owing to cardio-circulatory dysfunction and toxic injuries, such as radiocontrast media, antimicrobial agents, and heavy metals,Citation1 are common causes of degeneration and necrosis of tubular epithelium in these patients, resulting in AKI. Acute tubular necrosis (ATN) involves cell death and detachment from the basement membrane.Citation2 Less severe forms of AKI may be reversed with hydration and supportive measures as the development of ischemic ATN in previously healthy individuals usually experience a good prognosis. However, in many patients undergoing hospitalization because of chronic diseases or major surgery, extensive burns and sepsis in the recovering period of tubular lesions may be extended by subsequent nephrotoxicity and ischemic injuries with additional threat to the patient’s life. Indeed, under conditions leading to multiorgan failure, the AKI-associated lethality may reach 50%.Citation3 Hence, new therapies aiming to treat ARF are urgently needed.
In the last decade, several studies have demonstrated the capacity of adult bone marrow–derived cells from both human and rodent cells to differentiate into multiple cell lineages, including tubular epithelial and mesangial cells.Citation4,Citation5 Recent experimental data have indicated that bone marrow cells migrate to the kidney lesions, ameliorate renal function, and reduce tubular injury in AKI, through differentiation within the tubular compartment and by secreting growth and trophic factors.Citation6 In addition, infusion of bone marrow–derived mesenchymal stem cells may contribute to improvement of kidney lesions by a paracrine action.Citation7 Therefore, cell therapy utilizing adult bone marrow–derived cells has appeared as an alternative therapeutic strategy to treat renal diseases.
Here, we used a nephrotoxic AKI mouse model induced by mercuric chloride (HgCl2) to evaluate if the treatment with transplanted BMMC improves kidney biochemical function and reduces mortality.
MATERIALS AND METHODS
Animals and Induction of ATN
Six-to-eight-weeks-old female or male BALB/c mice weighting 20–23 g raised and maintained at the Gonçalo Moniz Researcher Center/FIOCRUZ were used. Animal experiments were conducted in conformity with NIH guidelines and approval of a local animal ethics committee. To induce ATN, female mice received subcutaneous injection of mercuric chloride (Sigma-Aldrich, Inc. St. Louis, MO) at dose of 16 mg/kg dissolved in sterile 0.9% saline solution. The dose of HgCl2 was determined in accordance with preliminary experiments and corresponds to a lethal dose 90% (LD90). We determined the serum mercuric concentration by atomic absorption spectrophotometer PerkinElmer 1.100 B (PerkinElmer, Shelton, CT) at different time points (3, 6, and 12 h).
Bone Marrow Mononuclear Cell Therapy
BMMC were obtained from male BALB/c mice. Animals were killed by cervical dislocation and bone marrow of femurs and tibiae were removed by flushing. Bone marrow cell suspensions were separated by centrifugation on Ficoll gradient at 1000g for 15 min (Histopaque 1119 and 1077, 1:1; Sigma). The mononuclear cell fraction was collected and washed twice with DMEM medium (Dulbeco’s Modified Eagle Medium, Gibco, Carlsbad, CA), filtered over nylon wool, and resuspended in 0.9% saline solution. Groups of 10 mice were treated with one injection of BMMC (2 × 107 cells/mouse) by intravenous route (retro-orbital plexus) 3, 6, or 12 h after HgCl2 challenge and were evaluated during 10 days. Control group received saline solution in the same condition. The cell preparations were analyzed by flow cytometry to evaluate the presence of CD45, CD34, CD11b, CD117, and CD90 (BD Biosciences, Franklin Lakes, NJ).
Serum Biochemical Analysis
To access the renal and hydroelectrolyte functions, we determined serum levels of urea, creatinine, sodium, and potassium at 24, 48, or 72 h after cellular therapy. Urea and creatinine were spectrophotometrically measured using a total automated clinical chemistry analyzer system (A25, Biochemistry, Spain). Sodium and potassium were both accessed using an ion selective electrode EasyLyte Na/K analyzer (Medica, USA).
Histopathological Analyses
Groups of mice, transplanted or not with BMMC after 12 h of HgCl2 administration, were euthanized 24, 48, and 72 h after HgCl2 administration (n = 5/group/time point). Sections were analyzed by light microscopy after paraffin embedding and standard hematoxylin and eosin staining. Images were digitalized using a color digital video camera (CoolSnap cf, Media Cybernetics, Carlsbad, CA) adapted to an AX-70 microscope (Olympus, Tokyo, Japan). Histopathological analyses were performed at the same times, looking for luminal hyaline casts and tubular cell degenerative changes. Lesions were scored according to Jablonsky et al.Citation8
Statistical Analyses
The statistical analysis was carried out by Prism 4 (GraphPad, San Diego, USA), statistical package. After descriptive statistics and normality test (Kolmogorov–Smirnov), the inferential analysis was chosen and carried out by Log Rank, Student t-tests, and ANOVA followed by Turkey’s or Newman–Keuls multiple post test, when necessary. The level of significance chosen was 0.05 to an interval confidence of 95%.
RESULTS
To investigate the effects of BMMC transplantation in HgCl2-induced lethality, groups of BALB/c mice were treated with BMMC by intravenous route at different times after drug challenge. The BMMC cell preparations were analyzed by flow cytometry and contained 97% CD45, 1.6% CD34, 52% CD11b, 1.1% CD117, 3.15% CD90, and 98.1% cell viability. The survival rate of mice treated with BMMC after 12 h of HgCl2 challenge was significantly higher than that of the control group (60% vs. 25%; p < 0.05, Kaplan–Meier log rank test). In contrast, protection was not observed when BMMC was injected 3 and 6 h after HgCl2 administration ().
Figure 1. Survival curves of mice challenged with HgCl2 transplanted with BMMC. HgCl2-challenged mice (n = 10 per group) received saline or BMMC intravenously at different times after HgCl2 administration (3, 6, and 12 h; n = 8, on each group). Mortality was evaluated up to 10 days after challenge.Note: *p < 0.05, Kaplan–Meier log rank test.
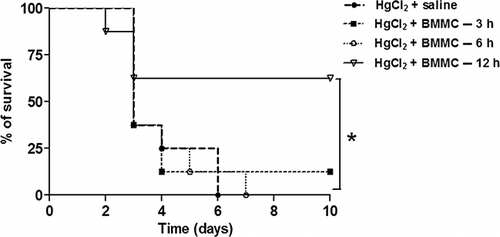
We hypothesized that the lack of protection when BMMC was given at 3 and 6 h could be caused by cytotoxicity because of highest mercury serum concentrations before 12 h of HgCl2 administration. To test this hypothesis, mercury serum levels were measured in mice treated with BMMC 3, 6, and 12 h after HgCl2 challenge. However, we did not find significant differences among HgCl2 concentrations in samples collected from the different time points ().
Figure 2. Serum mercuric concentration before and after BMMC transplantation. Groups of mice were challenged with HgCl2. Serum mercuric concentrations were determined 0, 3, 6, and 12 h after challenge (p > 0.05; ANOVA, Turkey’s Multiple Comparison test). Values represent individual mice, n = 8–10 per group.
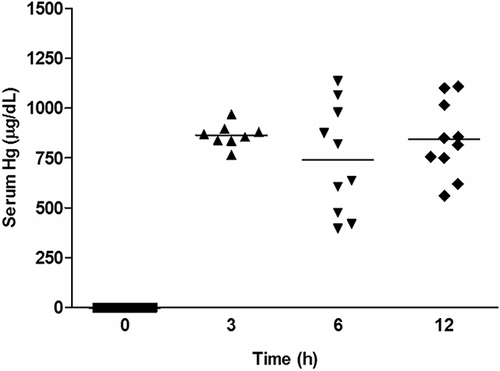
To evaluate if the cell therapy had effects on kidney function, we analyzed several biochemical parameters in the serum of animals treated or not with BMMC (). Transplantation of BMMC caused a decrease in serum creatinine and urea concentrations (p = 0.0409; ANOVA, Newman–Keuls Multiple Comparison Test) 48 h after HgCl2 challenge (A and B, respectively). The reduction in creatinine levels shows a tendency to achieve significance (p = 0.0598; ANOVA, Newman–Keuls Multiple Comparison Test). Hydroelectrolyte balance was accessed by determination of serum sodium and potassium concentrations. After cell therapy, serum potassium levels were significantly diminished in BMMC-treated animals (p < 0.01; ANOVA, Newman–Keuls Multiple Comparison Test).
Figure 3. Serum concentrations of urea, creatinine, potassium, and sodium in HgCl2-challenged mice transplanted or not with BMMC. Groups of mice were challenged with HgCl2 and transplanted with BMMC 12 h later. Serum urea (p = 0.0409) and potassium levels were significantly diminished in treated animals after cell therapy, (p < 0.01; ANOVA, Newman–Keuls Multiple Comparison Test) (3B and C). The reduction on creatinine and sodium levels did not achieve significance (p > 0.05; ANOVA, Newman–Keuls Multiple Comparison Test) (3A and D). Values represent the means ± SEM of 5 mice/group.
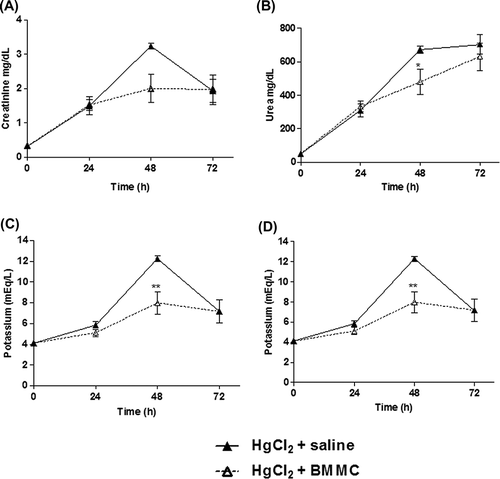
Figure 4. Histological analysis of kidney sections from HgCl2-injured mice treated (4B, D, and F) or not (4A, C, and E) with BMMC. Alterations in kidney morphology were observed 24 (4A and B), 48 (4C and D), and 72 h (4E and F) after HgCl2 injection. Magnifications 600×. Degeneration of tubular cells (black arrows), cellular debris (white arrows), and intratubular protein cylinder (blue arrows). Representative images of groups of mice euthanized at each time point (n = 5/group/time point).
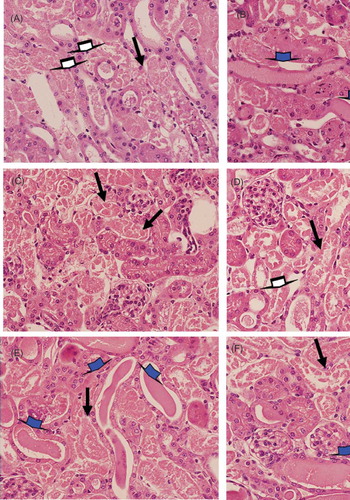
Independent of the fact that BMMC transplantation had ameliorated renal function and reduces mortality, it did not improve kidney lesions, which was confirmed by histopathology of renal tissue. In both groups, we observed type IV lesions, according to Jablonsky et al.,8 which represent the highest lesion score of ATN ().
DISCUSSION
Several studies in the literature have investigated the effects of cell therapy in AKI. Some were carried out using ischemia/reperfusion models.Citation9,Citation10 Others used drugs such as cisplatin or folic acid to induce AKI.Citation11,Citation12 Here, we used a model of nephrotoxic AKI induced by HgCl2, based on a standardized survival evaluation protocol already published.Citation13 Mercuric chloride at high doses causes an increase in serum creatinine, urea, and potassium. According to Eaton and Poorler,Citation14 uremia and increases in serum creatinine are indicative of a fall in glomerular filtration rate (GFR). In addition, mercuric chloride injection directly affects the heart, leading to induction of arrhythmias and ventricular fibrillationCitation15,Citation16 and deleterious effects in the immune system.Citation17,Citation18
It was demonstrated that transplanted BMMC suppress serum urea and creatinine levels in HgCl2-treated mouse after 48 h, indicating a protective therapeutic action in the kidney function. Furthermore, we observed an increase in survival when BMMC was injected 12 h after injury. Similar results were found by Li et al.10 Considering the current literature, this improvement of kidney function may be related to the prevention of the initial fall in GFR9, possibly through paracrine actions that contributes to proliferation of endogenous survivor cells or stimulates the proliferation and differentiation of kidney resident stem cells.10 By using BMMC obtained from GFP-transgenic mice, we found that the majority of the cells migrated to the spleen, bone marrow, and lungs of transplanted mice.Citation19 However, transplanted cells were rarely seen in kidney sections of HgCl2-challenged mice, reinforcing the paracrine effect of BMMC (our unpublished data). In fact, in previous studies, we observed a potent immunomodulatory action of transplanted BMMC in models of fulminant hepatitis, Chagas disease cardiomyopathy, and epilepsy.19–Citation22 Since HgCl2 induces the production of pro-inflammatory mediators, including TNF-α upon HgCl2 administration,17,18 it is also possible that cells in this model exert an anti-inflammatory action. Among the deleterious effects of TNF-α is cardiac dysfunction by induction of mitochondrial oxidative stress,Citation23 reinforcing the possibility of a protective effect of BMMC on HgCl2-induced cardiac alterations.
Independent of pharmacokinetic mechanisms occurring immediately after subcutaneous administration, there was no difference among serum mercuric chloride concentration at 3, 6, and 12 h. At the 12 h time point, serum mercuric concentrations remained elevated, suggesting that mercuric toxicity did not influence BMMC effects. In addition, BMMC were injected systematically and therefore should not be ruled out based solely on the hypothesis that these cells may be promoting protective effect in others organs. It is already known that mercuric chloride has direct effects in the heart, leading to the induction of arrhythmias and ventricular fibrillation.15,16 Since hyperkalemia affects cardiomyocyte membrane potential, which is a major cause of cardiac arrest, it may aggravate arrhythmias induced by HgCl2 injection as well. Thus, the observed reduction of hyperkalemia found in BMMC-treated animals suggests the possibility that BMMC cell therapy prevents arrhythmias prompted by normalization of serum potassium.
In histopathological analysis carried out by light microscopy, we did not observe a preservation of tubular structure in the BMMC-treated group. As shown by Morigi et al.,Citation24 on the first day, transplanted BMMC did not improve histopathological parameters when compared to the control group. However, after the fourth day, this was verified in ultrastructure analyses, improvement to the lesions from BMMC-treated group, which included diminution of tubular detachment. Although ultrastructural analysis was not performed in this study, a potential protection of tubular structure induced by BMMC was not sufficient to change the histological pattern of tubular regeneration in this model of HgCl2-induced nephrotoxicity. Since we did not find morphological alterations by histopathological analysis, one possible explanation for the changes in blood biochemistry are hemodynamic changes in the kidney, altering the GFR, or tubular reabsorption. Several studies have shown the production of angiogenic factors, such as VEGF, by bone marrow–derived cells.7,Citation25 VEGF also acts directly on endothelial cells upregulating the expression of endothelial nitric oxide synthase and subsequently increasing nitric oxide release.Citation26 This may account for an increase in vasodilation in our model, as previously proposed in patients with limb ischemia in which an increase in endothelium-dependent vasodilation after BMMC transplantation was observed.Citation27
Finally, our findings indicate that BMMC can ameliorate kidney function during AKI, which includes preventing hydroelectrolyte disturbances. In fact, this study is the first report that demonstrates cell therapy–mediated prevention of hyperkalemia. More studies are necessary to investigate the mechanisms of action of transplanted bone marrow cells in renal injury. In the context of regenerative medicine, administration of bone marrow cells and cytokines or growth factors will contribute to increase BMMC therapeutic potential.
Declaration of interest
The authors report no conflicts of interest. The authors alone are responsible for the content and writing of the paper.
This research was funded by grants from CNPq, FAPESB, and FINEP.
REFERENCES
- Pino CJ, Humes HD. Stem cell technology for the treatment of acute and chronic renal failure. Translational Research. 2010;156:161–168.
- Sharfuddin AA, Molitoris BA. Pathophysiology of ischemic acute kidney injury. Nat. Rev. Nephrol. 2011;4:189–200.
- Macedo E, Zanetta DM, Abdulkader RC. Long-term follow-up of patients after acute kidney injury: patterns of renal functional recovery. PLoS One. 2012;7:e36388.
- Lin F, Cordes K, Li L, Hematopoietic stem cells contribute to the regeneration of renal tubules after renal ischemia–reperfusion injury in mice. J Am Soc Nephrol. 2003;14:1188–1199.
- Masuya M, Drake CJ, Fleming PA, Hematopoietic origin of glomerular mesangial cells. Blood. 2003;101:2215–2218.
- Bussolati B, Hauser PV, Carvalhosa R, Camussi G. Contribution of stem cells to kidney repair. Curr Stem Cell Res Therap. 2009;4(1):2–8.
- Otto WR, Wright NA. Mesenchymal stem cells: from experiment to clinic. Fibrogenesis & Tissue Repair. 2011;4:20.
- Jablonski P, Howden B, Marshall V, Scott D. Evaluation of citrate flushing solution using the isolated perfused rat kidney. Transplantation. 1980;30:239–243.
- Duffield JS, Park KM, Hsiao LL, Restoration of tubular epithelial cells during repair of the postischemic kidney occurs independently of bone marrow-derived stem cells. J Clin Invest. 2005;115:1743–1755.
- Li B, Cohen A, Hudson TE, Mobilized human hematopoietic stem/progenitor cells promote kidney repair after ischemia/reperfusion injury. Circulation. 2010;121:2211–2220.
- Fang TC, Alison MR, Cook HT, Proliferation of bone marrow-derived cells contributes to regeneration after folic acid-induced acute tubular injury. J Am Soc Nephrol. 2005;16:1723–1732.
- Szczypka MS, Westover AJ, Clouthier SG, Ferrara JLM, Humes HD. Rare incorporation of bone marrow-derived cells into kidney after folic acid-induced injury. Stem Cells. 2005;23:44–54.
- Tanaka-kagawa T, Suzuki M, Naganuma YN, Imura N. Strain difference in sensitivity of mice to renal toxicity of inorganic mercury. J. Pharmacol. Exp. Therap. 1998;285:335–341.
- Eaton DC, Pooler JP. Fisiologia Renal De Vander. Porto Alegre: Artmed; 2005.
- Massaroni L, Rossoni LV, Amaral SM, Stefanon I, Oliveira EM, Vassallo DV. Hemodynamic and electrophysiological acute toxic effects of mercury in anaesthetized rats and in langendorff perfused rat hearts. Pharmacol Res. 1995;32(1–2):27–36.
- Clarkson TW. Inorganic and organometal pesticides. In: R Krieger, ed. Handbook of Pesticide Toxicology. 2nd ed., Vol. 1. San Diego, CA: Academic Press; 2001:1371.
- Zdolsek JM, Söder O, Hultman P. Mercury induces in vivo and in vitro secretion of interleukin-1 in mice. Immunopharmacology. 1994;28(3):201–208.
- Ghosh A, Sil PC. A protein from Cajanus indicus Spreng protects liver and kidney against mercuric chloride-induced oxidative stress. Biol Pharm Bull. 2008;31(9):1651–1658.
- de Freitas Souza BS, Nascimento RC, de Oliveira SA, Transplantation of bone marrow cells decreases tumor necrosis factor-α production and blood-brain barrier permeability and improves survival in a mouse model of acetaminophen-induced acute liver disease. Cytotherapy. 2012;14(8):1011–1021.
- Soares MBP, Lima RS, Rocha LL, Transplanted bone marrow cells repair heart tissue and reduce myocarditis in chronic chagasic mice. Am J Pathol. 2004;164:441–447.
- Soares MB, Lima RS, Souza BS, Reversion of gene expression alterations in hearts of mice with chronic chagasic cardiomyopathy after transplantation of bone marrow cells. Cell Cycle. 2011;10(9):1448–1455.
- Costa-Ferro ZS, Souza BS, Leal MM, Transplantation of bone marrow mononuclear cells decreases seizure incidence, mitigates neuronal loss and modulates pro-inflammatory cytokine production in epileptic rats. Neurobiol Dis. 2012;46(2):302–313.
- Mariappan N, Soorappan RN, Haque M, Sriramula S, Francis J. TNF-alpha-induced mitochondrial oxidative stress and cardiac dysfunction: restoration by superoxide dismutase mimetic tempol. Am J Physiol Heart Circ Physiol. 2007;293(5):H2726–H2737.
- Morigi M, Imberti B, Zoja C, Mesenchymal stem cells are renotropic, helping to repair the kidney and improve function in acute renal failure. J Am Soc Nephrol. 2004;15:1794–1804.
- Kinnaird T, Stabile E, Burnett MS, Epstein SE. Bone-marrow-derived cells for enhancing collateral development: mechanisms, animal data, and initial clinical experiences. Circ Res. 2004;95(4):354–363.
- Bauters C, Asahara T, Zheng LP, Recovery of disturbed endothelium-dependent flow in the collateral-perfused rabbit ischemic hindlimb after administration of vascular endothelial growth factor. Circulation. 1995;91:2802–2809.
- Higashi Y, Kimura M, Hara K, Autologous bone-marrow mononuclear cell implantation improves endothelium-dependent vasodilation in patients with limb ischemia. Circulation. 2004;109(10):1215–1218.