Abstract
Background: Kidney injury commonly occurs following hemorrhagic shock. This study aims to observe the effects of mesenteric lymph duct ligation (MLDL) on the adenosine triphosphate (ATP) levels and the cell membrane adenosine triphosphatase (ATPase) activity in the kidneys of rats subjected to hemorrhagic shock. Methods: Wistar rats were assigned into sham, shock, and ligation groups. The hemorrhagic shock model was established in the shock and ligation groups, and MLDL was performed in the ligation group after resuscitation. Renal homogenates were prepared to determine the ATP and ATPase levels at 90 min after hemorrhage and at 0, 1, 3, 6, 12, and 24 h after resuscitation. Results: The ATP levels, and the Na+–K+–ATPase, Mg2+–ATPase, Ca2+–ATPase, and Ca2+–Mg2+–ATPase activities in the renal tissue of the shock group were lower than those in the sham group at the multiple time points. Furthermore, the corresponding values in the ligation group were significantly higher than those in the shock group at multiple time points. Conclusion: MLDL improves energy metabolism and enhances the ATPase activity in the kidneys of hemorrhagic shock rats, along with other mechanisms that alleviate renal injury after hemorrhagic shock.
Introduction
The kidney is a common target organ for hemorrhagic shock, and kidney injury is one of the important reasons by which hemorrhagic shock induces multiple organ dysfunction syndrome (MODS).Citation1,Citation2 Acute kidney injury occurs in approximately 30% of patients admitted to the intensive care unit and is commonly associated with MODS.Citation3 A previous research found that blockage of mesenteric lymph treated using mesenteric lymph duct ligation (MLDL) alleviates multiple organ injury such as in the kidney, lung, liver, and myocardium, and decrease the death rate of two-hit rats subjected to hemorrhage and lipopolysaccharide (LPS).Citation4 MLDL also attenuates kidney injury in hemorrhagic shock rats, and the underlying mechanism might be related to reduced detainment of polymorph nuclear neutrophils (PMN) and decreased inflammatory response and oxidative stress.Citation5 Hence, post-hemorrhagic mesenteric lymph return plays an important role in multiple organ injury.Citation6,Citation7
Several studies have suggested that energy metabolism disturbances play an important role in organ injury after hemorrhagic shock, which can be alleviated by administering ATP–MgCl2 and sex steroids.Citation8 Membrane pump dysfunction is the major mechanism underlying the kidney injury induced by hypodermic HgCl2 injection, intramuscular glycerin injection, intravenous lipopolysaccharide infusion, and intravenous snake venom infusion. Thus, enhancing membrane pump activity could alleviate kidney injury.Citation9–11 However, the effects of MLDL on the ATP level and membrane pump activity in the kidneys after hemorrhagic shock remain unclear. Therefore, the present study aims to examine the effects of MLDL on the ATP level and the ATPase activity in renal tissue after hemorrhagic shock, and to determine the mechanism by which MLDL alleviates renal injury.
Methods
Animals and grouping
Seventy-eight healthy Wistar rats (purchased from the Chinese Academy of Medical Sciences Animal Breeding Center, Beijing, China), weighing 220–300 g, were randomly divided into a sham group, a shock group (at 90 min after hemorrhage, and at 0, 1, 3, 6, 12, and 24 h after resuscitation, all n = 6), and a ligation group (at 1, 3, 6, 12, and 24 h after resuscitation, all n = 6).
All rats were maintained under barrier-sustained conditions with 12 h light–dark cycles and free access to standard laboratory food and water. The research protocol, which was carried out after a minimal acclimatization period of 5 d, complied with the Guideline for the Care and Use of Laboratory Animals (NIH Publication, 1996) and was approved by the Institutional Animal Use and Care Committee of Hebei North University. Before the experiment, the rats were fasted for 12 h with unlimited drinking water. All efforts were made to minimize the suffering of the animals.
Hemorrhagic shock model
The rats were generally anesthetized with pentobarbital sodium (50 mg/kg). Under sterile conditions, the right common artery and the left jugular vein were cannulated for hemorrhage and fluid resuscitation; 0.5% heparin sodium (1 mL/kg, 700 U/mL) was injected via the jugular vein to systematically inhibit coagulation. After 10 min of stabilization, hemorrhage was performed using an automatic withdrawal–infusion machine (ZCZ-50, Zhejiang University Medical Ltd., Hangzhou, China) within 3 min, low pressure was maintained for 90 min, and fluid resuscitation was conducted within 20 min, as previously reported.Citation5 After resuscitation, all rats received abdominal operations: the mesenteric lymph duct was ligated in the rats of the ligation group, whereas only cotton was threaded below the mesenteric lymph duct of the rats in the shock group. The rats of the sham group were anesthetized and subjected to neck and abdominal surgery as described above, excluding the hemorrhage and resuscitation.
Collection of renal tissue
The left kidney was obtained from each rat at different time points according to the study protocol after intraperitoneal injection with pentobarbital sodium (120 mg/kg). Then, the kidney was split by a longitudinal midline incision, half of them, including medulla and cortex, was mixed with nine times the amount of 4 °C normal saline to produce a 10% renal homogenate at low temperature using a FJ-200 type high-speed dispersion machine (Shanghai, China). The supernatant was collected by centrifugation for 10 min at 850 × g using a low-temperature centrifuge (Labofuge 400R, Heraeus, Hanover, Germany). The supernatant was stored in a −80 °C refrigerator (Thermo Electron, Waltham, MA).
Assessment of the ATP level in renal homogenate
The ATP levels in the renal homogenate were detected using liquid chromatography, as previously reported.Citation12,Citation13 First, the renal homogenate (0.5 mL) was mixed with 0.5 mL of 10% (v/v) perchloric acid and the resulting mixture was centrifuged at 3400 × g for 10 min. The supernatant was obtained and filtered through a 0.45 µm syringe-like filter membrane to assess the ATP level. A total of 1.0 mg of standard ATP sample (National Institute for the Control of Pharmaceutical and Biological Products, Beijing, China) was used to adjust the final volume (1.0 mL) in the mobile phase. Then, 1.0 mg/mL standard solutions were scored using a mobile phase meter as they were filtered into a 0.45-µm syringe-like filter membrane and diluted into different gradient concentrations. Based on the chromatographic conditions (chromatographic column: hypersil BDS C18; mobile phase: KH2PO4 0.2 mol/L, methanol = 90:10; flow rate: 1.0 mL/min; detection wavelength: Ex = 254 nm, Em = 338 nm; column temperature: 25 °C; injection volume: 20 μL), the peak areas of the standard ATP samples with varying concentrations were obtained using an hP1100 chromatograph (Agilent, Technologies, Palo Alto, CA). The standard curve (ATP = 35.89 × C + 41.24, r = 0.99928) was constructed based on the least squares method. Finally, the samples were detected individually, and their ATP content was quantitatively calculated according to the relative retention time and the external standard curve.
Detection of ATPase activity in the renal homogenate
The Na+–K+–ATPase, Ca2+–ATPase, Mg2+–ATPase, and Ca2+–Mg2+–ATPase activities in the renal homogenate were detected with the phosphorus fixing method using a commercially available kit (Jiancheng Biotechnology, Nanjing, China), as previously reported.Citation9,Citation12,Citation14,Citation15 One unit of ATPase activity represents the amount of enzyme that will produce 1 mmol/h of organic phosphorus from the hydrolysis of ATP into ADP. The protein content of the homogenate was quantified via the Coomassie brilliant blue colorimetric method using a kit (Jiancheng Biotechnology, Nanjing, China).Citation12,Citation16 The results are expressed as units of ATPase per milligram of protein.
Statistical analysis
All data are presented as mean ± SD. Statistical analysis was performed using the SPSS 16.0 software (Polar Engineering and Consulting Inc., Chicago, IL). A two-way ANOVA was used to analyze differences between groups at multiple points, and a Student–Newman–Keuls (SNK) test was used within two groups. Differences with p values <0.05 were considered statistically significant.
Results
Effect of MLDL on the ATP level in the renal tissue of hemorrhagic shock rats
After subjecting the rats to hemorrhagic shock, the ATP content of the renal homogenate gradually declined and was markedly lower at any time point compared with the sham group. The ATP levels were low from 3 h to 12 h after resuscitation and were significantly less than those at 90 min after hemorrhagic shock, and at 0 h and at 1 h after resuscitation. The ATP levels increased at 1 h and 24 h after resuscitation ().
Figure 1. Effect of mesenteric lymph duct ligation on the ATP level in the renal tissue of hemorrhagic shock rats (mean ± SD, n = 6). *p < 0.05 versus the sham group; #p < 0.05 versus the shock at 90 min; △p < 0.05 versus the shock group after resuscitation 0 h; □p < 0.05 versus the shock group after resuscitate 1 h; ▴p < 0.05 versus the shock group at same time points.
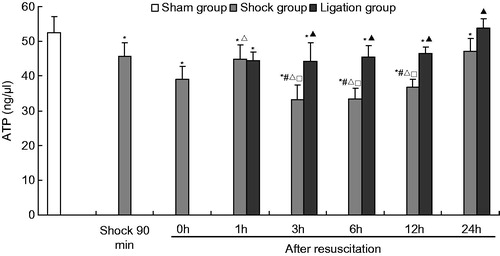
When MLDL was carried out on the rats, the ATP content in the renal tissue in the ligation group was also maintained at a relatively low level from 1 h to 12 h after fluid resuscitation, even lower than that in the sham group. However, the ATP levels in the ligation group were significantly higher than that of the shock group at the corresponding time points from 3 h to 24 h after resuscitation.
Effect of MLDL on the ATPase activity in the renal tissue of hemorrhagic shock rats
The Na+–K+–ATPase activity in the shock group at 90 min after hemorrhagic shock was higher than that of the sham group. No significant difference in the Mg2+–ATPase, Ca2+–ATPase, and Ca2+–Mg2+–ATPase activities were observed between the two groups ().
Figure 2. Effect of mesenteric lymph duct ligation on the Na+–K+–ATPase activity in the renal tissue of hemorrhagic shock rats (mean ± SD, n = 6). *p < 0.05 versus the sham group; #p < 0.05 versus the shock at 90 min; △p < 0.05 versus the shock group after resuscitation 0 h; ▴p < 0.05 versus the shock group at same time points.
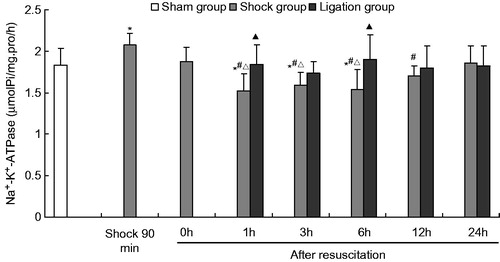
Figure 3. Effect of mesenteric lymph duct ligation on the Ca2+–ATPase activity in the renal tissue of hemorrhagic shock rats (mean ± SD, n = 6). *p < 0.05 versus the sham group; #p < 0.05 versus the shock 90 min; ▴p < 0.05 versus the shock group at same time points.
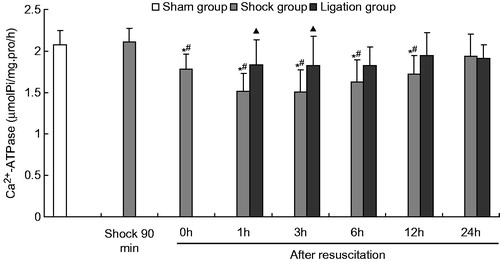
Figure 4. Effect of mesenteric lymph duct ligation on the Mg2+–ATPase activity in the renal tissue of hemorrhagic shock rats (mean ± SD, n = 6). *p < 0.05 versus the sham group; #p < 0.05 versus the shock at 90 min; △p < 0.05 versus the shock group after resuscitation 0 h; ▴p < 0.05 versus the shock group at same time points.
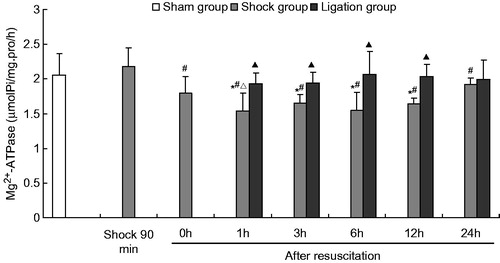
Figure 5. Effect of mesenteric lymph duct ligation on the Ca2+–Mg2+–ATPase activity in the renal tissue of hemorrhagic shock rats (mean ± SD, n = 6). *p < 0.05 versus the sham group; #p < 0.05 versus the shock at 90 min; ▴p < 0.05 versus the shock group at same time points.
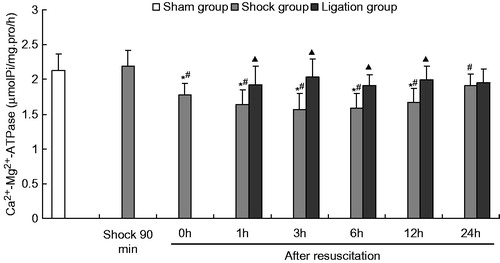
After fluid resuscitation, the ATPase activity in the shock group decreased and subsequently increased. The Na+–K+–ATPase activity at 1, 3, and 6 h; the Ca2+–ATPase and Ca2+–Mg2+–ATPase activities at 0, 1, 3, 6, and 12 h; and the Mg2+–ATPase activity at 1, 3, 6, and 12 h were significantly lower than those of the sham group. The Na+–K+–ATPase activity at 1, 3, 6, and 12 h; the Ca2+–ATPase and Ca2+–Mg2+–ATPase activities at 0, 1, 3, 6, and 12 h; and the Mg2+–ATPase activity at 1, 3, 6, 12, and 24 h were significantly lower than those at the 90 min after hemorrhage. Moreover, the Na+–K+–ATPase activity at 1, 3, and 6 h; and Mg2+–ATPase at 1 h was significantly lower those at 0 h after resuscitation.
Meanwhile, the Na+–K+–ATPase, Mg2+–ATPase, Ca2+–ATPase, and Ca2+–Mg2+–ATPase activities in the ligation group did not significantly differ from those in the sham group at any time point after resuscitation. In the ligation group, the Na+–K+–ATPase activity at 1 h (1.845 ±0.234 µmolPi/mg pro/h) and 6 h (1.899 ± 0.298 µmolPi/mg pro/h) after resuscitation, the Ca2+–ATPase activity at 1 h (1.838 ± 0.299 µmolPi/mg pro/h) and 3 h (1.826 ±0.358 µmolPi/mg pro/h) after resuscitation, and the Mg2+–ATPase and Ca2+-Mg2+-ATPase activities at 1 h (1.932 ±0.152, 1.918 ± 0.271 µmolPi/mg pro/h), 3 h (1.936 ± 0.160, 2.036 ± 0.259 µmolPi/mg pro/h), 6 h (2.065 ± 0.339, 1.906 ±0.156 µmolPi/mg pro/h), and 12 h (2.039 ± 0.172, 1.996 ±0.192 µmolPi/mg pro/h) after fluid resuscitation were higher than that of the shock group (1.526 ± 0.205, 1.543 ± 0.241, 1.516 ± 0.214, 1.504 ± 0.269, 1.535 ± 0.259, 1.636 ± 0.215, 1.647 ± 0.132, 1.571 ± 0.223, 1.543 ± 0.266, 1.592 ± 0.208, 1.643 ± 0.082, and 1.671 ± 0.199 µmolPi/mg pro/h, respectively). At 24 h after fluid resuscitation, the Na+–K+–ATPase, Mg2+–ATPase, Ca2+–ATPase, and Ca2+–Mg2+–ATPase activities did not significantly differ between the shock and the ligation groups.
Discussion
Mesenteric lymph return plays an important role during MODS and the systemic inflammatory response syndrome (SIRS) induced by bacteria/endotoxin translocation (BET) following hemorrhage, trauma, burn, and endotoxemia.Citation6,Citation17–22 In the present study, we found that MLDL enhances the ATP level and the ATPase activity in the kidneys of rats following hemorrhagic shock, which is beneficial for improving renal function and structure.
Microcirculation disturbances induced by trauma and hemorrhage are a major cause of disorders in energy metabolism, which is an important mechanism for cell injury after shock.Citation23 The results of the present study show that the ATP content of the kidneys in the shock group rats exhibited a downward trend at 90 min after hemorrhage and 0 h after resuscitation. We speculate that this trend may be related to hemorrhage-induced renal ischemia through the excitement of the sympathetic nerve and the adrenal medulla system, and the release of vasoactive substances; however, it needs new evidences. The ATP levels in the kidneys exhibited an upward trend at 1 h after resuscitation, which was induced by the recovery of perfusion in the kidneys. However, the ATP levels sequentially decreased and remained low from 3 h to 12 h, likely because of the kidney injury, which has been verified in a previous study.Citation5 Moreover, the ATP content increased at 24 h after resuscitation, which helped these animals survive. Hence, the energy metabolism disorder not only causes cell injury, but also results from cell injury.
In the ligation group, after mesenteric lymph blockage via MLDL, the ATP levels in the kidney exhibited an increasing trend from 3 h to 24 h compared with the shock group. This trend demonstrates the protective effect of the mesenteric lymph blockage on energy metabolism after hemorrhagic shock, which is one of the mechanisms by which MLDL alleviates kidney injury. MLDL limited the transport of poisonous components through the mesenteric lymph after hemorrhagic shock, thereby improving the microcirculation disturbance and the oxygen consumption in the kidneys, resulting to the balance of energy metabolism.
In the present study, the Na+–K+–ATPase, Ca2+–ATPase, Mg2+–ATPase, and Ca2+–Mg2+–ATPase activities decreased at multiple time points from 0 h to 12 h after fluid resuscitation. The decreased ATPase activity produced an abnormal electrochemical gradient and transported ions between the intracellular environment and the extracellular environment,Citation9,Citation10,Citation24 resulting in cellular swelling and injury.Citation25 ATPase dysfunction is related to the higher inflammatory mediators induced by hemorrhage and acidosis caused by the disorder in energy metabolism.Citation26 Therefore, these results demonstrate that ATPase dysfunction is an important link between hemorrhagic shock and kidney injury.
Interestingly, preventing the return of mesenteric through ligation of the mesenteric lymph duct significantly improved the ATPase activity in the ligation group, which is an important mechanism of MLDL alleviating kidney injury. The beneficial effect is realized by reducing the inflammatory response,Citation5 meanwhile, it is related to improving energy metabolism through the result of MLDL on ATP in this study. However, more research is needed to determine whether MLDL can lower lactic acid content and improve acid–base status. In addition, mitochondrial dysfunction and injury are important factors during the energy metabolism disturbances and organ injury following hemorrhagic shock,Citation27,Citation28 because mitochondria is a key organelle of ATP production. Therefore, whether MLDL can improve the mitochondrial structure and function needs further investigation.
In conclusion, MLDL after hemorrhagic shock improves the ATP levels and enhances ATPase activity, including Na+–K+–ATPase, Ca2+–ATPase, Mg2+–ATPase, and Ca2+–Mg2+–ATPase. These results further indicate that mesenteric lymph plays a negative effect in the pathogenesis of hemorrhagic shock-induced kidney injury. The present study provides novel insights into the prevention and cure of MODS caused by severe hemorrhagic shock, and suggests that the mesenteric lymph can be used as a therapeutic target for preventing kidney injury. However, the components of post-hemorrhagic shock mesenteric lymph need further examination.
Declaration of interest
No benefits in any form have been received or will be received from a commercial association related directly or indirectly to the subject of this article. The authors report no conflicts of interest. The authors alone are responsible for the content and writing of the paper.
This study was supported by the National Natural Science Foundation of China (30370561); the Key scientific and technological project of Hebei Province (11276103D-84); and the Foundation of Hundred Innovative Talents in Universities of Hebei Province (CPRC047 and CPRCII026).
References
- Mizock BA. The multiple organ dysfunction syndrome. Dis Mon. 2009;55(8):476–526
- Lee CJ, Peng TC, Hsu BG, et al. Lack of exercise decreases survival and increases organ damage after hemorrhagic shock in rats. Biol Res Nurs. 2010;12(2):198–205
- Faubel S. Acute kidney injury and multiple organ dysfunction syndrome. Minerva Urol Nefrol. 2009;61(3):171–188
- Niu CY, Li JC, Zhao ZG, et al. Effect of intestinal lymphatic circulation blockage in two-hit rats. World J Gastroenterol. 2006;12(36):5805–5812
- Niu CY, Zhao ZG, Ye YL, et al. Mesenteric lymph duct ligation against renal injury in rats after hemorrhagic shock. Ren Fail. 2010;32(5):584–591
- Deitch EA. Gut lymph and lymphatics: a source of factors leading to organ injury and dysfunction. Ann N Y Acad Sci. 2010;1207(Suppl. 1):E103–E111
- Fanous MY, Phillips AJ, Windsor JA. Mesenteric lymph: the bridge to future management of critical illness. JOP. 2007;8(4):374–399
- Jarrar D, Wang P, Chaudry IH. Metabolic disturbances in shock, and the role of ATP–MgCl2 and sex steroids. Med Princ Pract. 2004;13(1):2–9
- Zhao ZG, Niu CY, Zhang YP, et al. The mechanism of myocardium and pancreas injury in rabbits with acute renal failure might be related to myeloperoxidase and membrane pump activities. Ren Fail. 2010;32(10):1216–1222
- Zhao ZG, Niu CY, Zhang LL, et al. Exogenous normal lymph alleviates lipopolysaccharide-induced acute kidney injury in rats. Ren Fail. 2013;35(6):806–811
- Linardi A, Rocha e Silva TA, Miyabara EH, et al. Histological and functional renal alterations caused by Bothrops alternatus snake venom: expression and activity of Na+/K+-ATPase. Biochim Biophys Acta. 2011;1810(9):895–906
- Zhao ZG, Niu CY, Shang AM, et al. Mesenteric lymph reperfusion exacerbates brain injury in rats with superior mesenteric artery occlusion shock. Neural Regen Res. 2010;5(9):683–689
- Ran J, Guo X, Li Q, et al. Platelets of type 2 diabetic patients are characterized by high ATP content and low mitochondrial membrane potential. Platelets. 2009;20(8):588–593
- Zhao ZG, Niu CY, Zhang YP, et al. The mechanism of spleen injury in rabbits with acute renal failure. Ren Fail. 2011;33(4):418–425
- Fedorova OV, Lakatta EG, Bagrov AY. Endogenous Na, K pump ligands are differentially regulated during acute NaCl loading of Dahl rats. Circulation. 2000;102(24):3009–3014
- Bautista J, Corpas R, Ramos R, et al. Brain mitochondrial complex I inactivation by oxidative modification. Biochem Biophys Res Commun. 2000;275(3):890–894
- Reino DC, Pisarenko V, Palange D, et al. Trauma hemorrhagic shock-induced lung injury involves a gut-lymph-induced TLR4 pathway in mice. PLoS One. 2011;6(8):e14829
- Niu CY, Hou YL, Zhao ZG, et al. Role of intestinal lymphatic pathway in pathogenesis of intestine-derived bacteria/endotoxin translocation in rats in shock. Zhongguo Wei Zhong Bing Ji Jiu Yi Xue. 2007;19(5):266–269
- Levy G, Fishman JE, Xu D, et al. Parasympathetic stimulation via the vagus nerve prevents systemic organ dysfunction by abrogating gut injury and lymph toxicity in trauma and hemorrhagic shock. Shock. 2013;39(1):39–44
- Lee MA, Yatani A, Sambol JT, et al. Role of gut-lymph factors in the induction of burn-induced and trauma-shock-induced acute heart failure. Int J Clin Exp Med. 2008;1(2):171–180
- Gosain A, Gamelli RL. Role of the gastrointestinal tract in burn sepsis. J Burn Care Rehabil. 2005;26(1):85–91
- Lee SW, Hong YS, Park DW, et al. Lactic acidosis not hyperlactatemia as a predictor of in hospital mortality in septic emergency patients. Emerg Med J. 2008;25(10):659–665
- Hsieh YC, Frink M, Kawasaki T, et al. Downregulation of TLR4-dependent ATP production is critical for estrogen-mediated immunoprotection in Kupffer cells following trauma-hemorrhage. J Cell Physiol. 2007;211(2):364–370
- Wang Q, Lian QQ, Li R, et al. Lipoxin A (4) activates alveolar epithelial sodium channel, Na,K-ATPase, and increases alveolar fluid clearance. Am J Respir Cell Mol Biol. 2013;48(5):610–618
- Berger G, Guetta J, Klorin G, et al. Sepsis impairs alveolar epithelial function by downregulating Na–K–ATPase pump. Am J Physiol Lung Cell Mol Physiol. 2011;301(1):L23–L30
- Szabó C, Módis K. Pathophysiological roles of peroxynitrite in circulatory shock. Shock. 2010;34(Suppl. 1):4–14
- Wang X, Song R, Chen Y, et al. Polydatin – a new mitochondria protector for acute severe hemorrhagic shock treatment. Expert Opin Investig Drugs. 2013;22(2):169–179
- Song R, Bian H, Wang X, et al. Mitochondrial injury underlies hyporeactivity of arterial smooth muscle in severe shock. Am J Hypertens. 2011;24(1):45–51