Abstract
Background: Calcium-sensing receptor gene (CaSR) might be a strong component of the complex genetic background regulating calcium excretion and stone formation in kidney. The polymorphisms in different parts of the gene could be associated with calcium stone formation. Objective: The individual studies indicate a correlation between CaSR gene polymorphisms and urolithiasis but not sufficient to draw a precise result. We aimed to search literature to come to a reliable conclusion through a systematic review. Materials and methods: We have serached individiual studies regarding the issue and high quality reviews. We assessed all the studies to draw a conclusion. Conclusion: The genetic background of the neprolithiasis is an interesting issue deserving to be disscussed on. Regading genes of which, CaSR gene polymorphisms is the leading one, are studied in many individual studies but they are not sufficient to have a precise conclusion. Well-oriented and documented, phenotypically homogenous large cohort studies are needed for further evaluation.
Introduction
Urolithiasis is one of the most prevalent disorders in Urology practice which is estimated to be at 1–15% with the probability of having a stone varying according to age, gender, race, and geographic location. In the United States, the prevalence of stone disease has been estimated at 10–15%.Citation1–3 The most common component of urinary calculi is calcium, which is a major constituent of nearly 75% of stones. Calcium oxalate makes up about 60% of all stones; mixed calcium oxalate and hydroxyapatite, 20%; and brushite stones, 2%.
The calcium-sensing receptor (CaSR) is the key controller of extracellular calcium homeostasis via its effects on regulation of parathyroid hormone (PTH) secretion and renal calcium reabsorption. The CaSR is a G-protein-coupled membrane receptor that allows parathyroid and kidney tubular cells to regulate parathyroid hormone (PTH) secretion and tubular calcium reabsorption according to the plasma calcium concentration. The CaSR molecule comprises a large extracellular domain, a transmembrane domain, and an intracellular tail. Calcium binding to the extracellular domain stimulates the interaction of transmembrane and intracellular domains with G-proteins and activates a complex network of intracellular messengers, leading to phospholipase C-dependent inositol 1,4,5-triphosphate production and thus calcium release from intracellular stores as self-maintained low frequency repetitive calcium spikes. Through this cascade of events, CaSR activation inhibits PTH secretion and calcium reabsorption in the thick ascending limb. Thus, CaSR activation increases urinary calcium excretion by both a direct and a PTH-mediated effect on kidney tubular cells. While the activation of CaSR especially in thick ascending limb results in hypercalciuria which forms the main risk factor for renal stone formation, CaSR activation in collecting ducts of nephron decreases the risk to renal stone formation by urinary pH regulation.
Calcium-hypercalciuria
The regulation of calcium (Ca+2) metabolism is accomplished by the concerted action of the intestine, kidney, and bone. Ca+2 is absorbed from the diet in multiple segment of intestine.Citation4 Thirty percent to 40% of dietary calcium is absorbed from intestine, with most being absorbed in the small intestine, and only approximately 10% absorbed in the colon.Citation4 Calcium is absorbed in the ionic state, and incomplete calcium absorption due in part to formation of soluble calcium complexes in the intestinal lumen. Substances that complex calcium such as phosphate, citrate, oxalate, and sulfate reduce the availability of ionic calcium for absorption.Citation5 The active vitamin D is the most potent stimulator of intestinal calcium absorption. The conversion of 25-hydroxyvitamin D3 to 1,25 (OH)2D3 is succeeded in kidney with the stimulation of parathyroid hormone (PTH). A decrease in serum calcium increases secretion of PTH and it stimulates the enzyme 1α-hydroxylase, which is located in the mitochondria of the proximal renal tubule. PTH is a crucial hormone in maintaining serum calcium concentration within normal range. In response to serum calcium levels, extracellular CaSR regulates PTH secretion and renal tubular calcium reabsorption.Citation6 PTH stimulates osteoclasts, further raising serum calcium. At the kidney, PTH enhances renal calcium reabsorption and reduces renal tubular reabsorption of phosphate. It also stimulates synthesis of 1,25(OH)2D3, which leads to enhanced intestinal calcium and phosphate absorption. PTH does not affect intestinal absorption directly.
Hypercalciuria is the most common abnormality identified in calcium stone formers seen in up to 40% of the stone formers.Citation7–10 The majority of Ca++ reabsorption takes place paracellularly along the proximal tubule (PT: 60–65% of filtered Ca++) and the thick ascending limb of Henle’s loop (TALH: 25–30% of filtered Ca++). The distal convoluted tubule (DCT) reabsorbs the remaining 8–10% of filtered Ca++ transcellularly.Citation11 High urinary calcium concentrations lead to increased urinary saturation of salts.Citation12 Additionally, citrate, which is the common urinary inhibitor is reduced by the way of complication in hypercalciuric statement of urine.Citation13 Intestine, bone and kidney are the main sites regulating calcium transport and any dysregulation of these sites result in hypercalciuria. Although the classic classification, which is formed on the basis of unique pathophysiologic abnormalities, is used widely with its utility in simplifying the understanding and treatment of metabolic derangements, many have argued that hypercalciuria is associated with multiple, interrelated disturbances that cannot be simply separated into a specific organ system.Citation8
Calcium sensing receptor in kidney
Since the CaSR is expressed at multiple sites along the nephron, it is reasonable to discuss its function according to its cellular localization depends upon the region of the nephron where it is expressed. It was illustrated in .Citation14
Figure 1. Intrarenal localization and roles of the CaSR. Notes: Cellular polarity of the CaSR is apical [in the proximal tubule and outer/inner medullary collecting duct (OMCD/IMCD)] and basolateral [in the thick ascending limb (TAL) and, occasionally, in the cortical collecting duct (CCD)]. Species differences exist in the distal convoluted tubule (DCT)/connecting segment (CNT), where receptor expression can be detected apically and/or basolaterally/intracellularly. PCT/PST, proximal convoluted/straight tubule; tDL/tAL, thin discending/ascending limb; MTAL/CTAL, medullary/cortical thick ascending limb; JGA, juxtaglomerular apparatus; TRPV5, transient receptor potential vanilloid 5; PMCA, plasma membrane Ca2_-ATPase; VitD, vitamin D; VDR, vitamin D receptor; NKCC2, Na_-K_-2Cl_ cotransporter 2; ROMK, renal outer medullary potassium K_ channel; PTH, parathyroid hormone; AQP2, aquaporin 2.
![Figure 1. Intrarenal localization and roles of the CaSR. Notes: Cellular polarity of the CaSR is apical [in the proximal tubule and outer/inner medullary collecting duct (OMCD/IMCD)] and basolateral [in the thick ascending limb (TAL) and, occasionally, in the cortical collecting duct (CCD)]. Species differences exist in the distal convoluted tubule (DCT)/connecting segment (CNT), where receptor expression can be detected apically and/or basolaterally/intracellularly. PCT/PST, proximal convoluted/straight tubule; tDL/tAL, thin discending/ascending limb; MTAL/CTAL, medullary/cortical thick ascending limb; JGA, juxtaglomerular apparatus; TRPV5, transient receptor potential vanilloid 5; PMCA, plasma membrane Ca2_-ATPase; VitD, vitamin D; VDR, vitamin D receptor; NKCC2, Na_-K_-2Cl_ cotransporter 2; ROMK, renal outer medullary potassium K_ channel; PTH, parathyroid hormone; AQP2, aquaporin 2.](/cms/asset/e5d12589-92e4-4bf8-973d-a66e2a672b6a/irnf_a_937673_f0001_b.jpg)
CaSR in proximal convoluted tubule
CaSR is expressed on the apical membrane of the proximal tubule cells and responds to the luminal calcium.Citation15 PTH results in phosphate excretion in proximal tubules by degradation of phosphate reabsorption carriers (NPT2c and NPT2a) in subapical vesicles derived from brush border. Microperfusion studies in single proximal tubules isolated from mice demonstrated that CaSR stimulates phosphate reabsorption by inhibition of cyclic AMP production and PTH-mediated internalization of phosphate reabsorption carriers, NPT2c and NPT2a.Citation16 Studies conducted in proximal tubule-derived cell lines also suggest that 1α-hydroxylase activity is inhibited in the presence of high Ca2+.Citation17 In a study carried out with a murine model in which the full-length CaSR has been ablated have shown that CaSR diminishes the response to 1,25(OH)2D3 independently of PTH actions.Citation18 Thus CaSR exerts a tight control on circulating 1,25(OH)2D3 both at the level of its synthesis (in the proximal tubule) and in modulating its effects. Conversely, 1,25(OH)2D3,Citation19 PTH, and dietary phosphate modulate both CaSR gene and protein expression in the proximal tubule,Citation20 suggesting the existence of a local feedback loop for the regulation of Ca2+ and Pi excretion independently of systemic changes in calciotropic hormones.
CaSR in TAL of Henle’s loop
The loop of Henle consists of four segments; the thin descending limb (DLH), the thin ascending limb (ALH), the medullary thick ascending limb (mTALH), and the cortical thick ascending limb (cTALH). CaSR is expressed on the basolateral membrane of the thick ascending limb of Henle loop.Citation15 About 20–25% of the filtered calcium is reabsorbed in the loop of Henle, largely by the cortical (CTAL) and, to a lesser extent, by the medullary (MTAL) thick ascending limb, through both transcellular and paracellular routes.Citation21 The apical Na+-K+-2Cl− cotransporter (NKCC2) and the renal outer medullary potassium K+(ROMK) channel generate the “driving force” which is crucial for calcium and sodium reabsorption.Citation22 In in-vitro studies on microdissected or isolated microperfused tubules from laboratory animals, CaSR activation by serum-interstitial calcium enhanced the intracellular production of arachidonic acid and 20-hydroxyeicosatetraenoic acid (HETE) that inactivates the potassium ROMK channel and the Na-K-2Cl cotransporter.Citation23 The inactivation of these carriers inhibits the passive reabsorption of sodium, potassium and chloride and blocks the potassium recycling to the lumen through the specific ROMK channel.Citation23 In thick ascending limbs isolated from dogs, CaSR also inhibited the phosphorylation of claudin-16 through a mechanism mediated by the decrease in the protein kinase A activity. The unphosphorilated form of claudin-16 was not expressed in tight junctions and its absence reduced the tight junction permeability to calcium and magnesium, thus amplifying the calciuric effect of CaSR.Citation24 demonstrates the activities of CaSR in a cell model from the thick ascending limb.Citation25
Figure 2. CaSR activities in a cell model from the thick ascending limb (TAL). Notes: CaSR decreases paracellular calcium reabsorption by inhibition of renal outer medullary potassium channel (ROMK)-mediated potassium recycling to the lumen that supports the luminal positive electric gradient. This gradient is the driving force for the passive paracellular calcium reabsorption in the TAL. AA, arachidonic acid; 20-HETE, 20-hydroxyeicosatetraenoic acid; PKA, protein kinase A; PLA2, phospholipase A2.
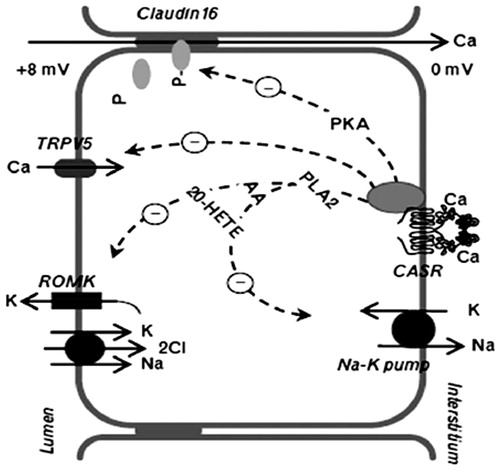
While NaCl reabsorption through NKCC2 is electroneutral (NKCC2 translocates 1 Na+, 1 K+, and 2 Cl− ions from the lumen into the cell), apical K+ represents the rate-limiting step of this process and K+ ions back-diffuse into the lumen through the ROMK channels.Citation21 Na+ and Cl− accumulated inside the cell are then transported into the bloodstream through basolateral Na+-K+-ATPase and Cl− channels, respectively. Overall, these processes yield a net cellular reabsorption of NaCl and the generation of a lumen-positive transepithelial potential difference, which drives nonselective cation reabsorption (largely Ca2+ and Mg2+ but also Na+) through the paracellular route.Citation21
During hypercalcemia, activation of the basolateral CaSR inhibits ROMK channelsCitation26 which contribute to the recycling of K+ into the lumen of the TAL.Citation27 This action of hypercalcemia limits the rate of Na+-K+-2Cl− cotransport by reducing the availability of luminal K+. Thus the greater the hypercalcemia, the greater is the inhibition of ROMK and NKCC2 and the faster the dissipation of the lumen-positive transepithelial voltage. Additionally, microperfusion experiments in mice single tubules evidenced that CaSR activation suppressed TRPV5 calcium channel expression in the apical membrane of TAL cellsCitation28 and transcellular PTH-induced calcium reabsorption in the cortical tract of the TAL.Citation29
In the MTAL water permeability is minimal because of the absence of luminal aquaporins, and water reabsorption does not follow paracellular divalent cation movements. The reduced permeability of this nephron segment would produce a rapid increase in basolateral NaCl and/or Ca2+/Mg2+ concentration, which could inhibit NKCC2, ROMK, and, possibly, CLN-16.
CaSR in distal convoluted tubule
Distal tubule accounts for 15% of total Ca2+ reabsorbed by the kidney. CaSR is located on the basolateral membrane of distal convoluted tubular cells. In cultured dog cells from this tubular segment CaSR was found to reduce the active calcium reabsorption by interfering with the calcium pump function through a phospholipase C-dependent mechanism. The signaling pathway for this effect requires activation of Gq proteins.Citation30 In DCT the transepithelial PD is against Ca2+ reabsorption and the paracellular permeability of Ca2+ ions is very low.Citation31 Ca2+ reabsorption is an active, transcellular process, which is regulated by PTH and 1,25(OH)2D3.Citation31,Citation32 CaSR reduces calcium pump activity (PMCA) and calcium active reabsorption in distal convoluted tubule ().Citation33
CaSR in collecting ducts
CaSR is represented on the apical membrane of principal and intercalated cells in collecting ducts of nephron segment. Experiments in hypercalciuric knockout mice for the TRPV5 calcium channel showed that CaSR stimulated proton-secreting H-pump in the type A intercalated cells and free water excretion by inhibition of aquaporin (AQP2) membrane expression in principal cells.Citation34,Citation35
The genetic background of stone formation
The development of calcium stone crystallization is a multifactorial disease in which genetic and environmental factors are included. Since 1960s, it is a well known fact that having stone-forming family relatives is a predisposition to have urolithiasis.Citation36–38 In a study conducted by Goldfarb DS et al.,Citation39 stone production was observed higher in monozygous than dizygous twins born in Vietnam. The proportion of kidney stone susceptibility attributable to genetic causesCitation39 was 56% indicating nephrolithiasis is a complex disease on which genetic and environmental factors have impact. That is why we should consider it as a complex disease like diabetes, hypertension and ischemic heart disease. It was also demonstrated in these family studies that nephrolithiasis transmission pattern was not Mendelian, complex polygenic pattern was available.
Recently, great importance is attributed to epigenetic modifications which is defined as regulation of gene expression without altering the DNA sequence. Epigenetic changes involve DNA methylation at cytosine-guanine sequences-CpG sites, histone posttranslational medications (histone methylation acetylation, phosphorylation, ubiquitination, simulation, and propionylation), and microRNAs.Citation40
The major aforementioned genes predisposing to susceptibility to renal stone forming are Claudin 14 (CLDN14), CaSR, Osteopontine (OPN), and Vit-D receptor (VDR). Because the genetic background of stone formation is a complicated issue including single-candidate genes as well as epigenetic process, it is not simple to identify which gene is more responsible from stone formation. Furthermore, environmental and dietary factors are also important that they might modify gene expressions at different biological levels, eventually influencing the phenotypic effects of gene products.
Calcium sensing receptor single nucleotide gene polymorphisms
Several studies have examined the possible impact of single nucleotide polymorphisms (SNPs) in the CaSR on Ca2+ homeostasis and kidney stone forming. In the CaSR gene, exon 1 is in the 50 untranslated region also including promoters, exon 2 presents the transcription starting site codon, and exon 7 encodes for the transmembrane domain and the intracellular tail.
One study found that CaSR SNPs and related haplotypes (a haplotype in this setting is a sequence of SNPs on a single strand of DNA) were a determinant of the normal range of serum Ca+2 concentration in the population.Citation41 This normal range is greater that measured in any given individual. Indeed, certain haplotypes of SNPs at positions 986, 990, and 1011 in the CaSR’s C tail were significantly associated with higher or lower serum ionized Ca+2 concentration within the normal range, and accounted for 17% of the variation in the normal range.Citation41 In some cases, other groups have had discordant results with regard to the A986S polymorphism,Citation42 perhaps because of small sample size or populations with differing frequencies of the SNPs. Clearly additional studies with larger sample sizes in well-characterized populations are needed.
The impact of CaSR SNPs on parameters related to renal function/dysfunction has also been studied. Having two glycine residues at position 990, instead of the more common alanine, has been associated with (1) lower PTH levels in hemodialysis patients,Citation43 (2) hypercalciuria,Citation44 and (3) greater suppressibility of PTH during induced hypercalcemia in hemodialysis patients.Citation45 In another study, there was a greater risk of stone disease in patients with PHPT and the ACG haplotype at positions 986, 990, and 1011.Citation46 Some associations of SNPs with traits relevant to the kidney have involved noncoding SNPs (those present in introns or the promoter of the CaSR gene), perhaps by modifying receptor expression. In African–Americans in the Indianapolis area, three SNPs were associated with systolic blood pressure and with urinary calcium excretion.Citation47 These same investigators observed an interaction between CaSR, the CLCNKB (the basolateral chloride channel in the thick limb), and NKCC genes that contributed to variation in diastolic blood pressure, perhaps through changes in sodium and/or calcium transport in the TAL.Citation48 These results indicate that the SNPs within the CaSR and other genes with which it interacts in the kidney could be a fruitful avenue of investigation. The availability of very large databases, with 1,000,000 SNPs for genomewide association studies (GWAS) should be very helpful in this regard. demonstrates the associated studies regarding calcium sensing receptor gene polymorphisms in patients with nephrolithiasis.
Table 1. The associated studies regarding calcium sensing receptor gene polymorphisms in patients with nephrolithiasis.
Conclusions
The findings of individual studies indicate that CaSR gene polymorphisms are implicated in the development of nephrolithiasis through different mechanisms. However, representing a cause and effect relationship for a suspected gene is not such simple. Many other factors, of which nutrition and environment are the most prominent, may include in the issue making it a quite complicated problem. The reasonable approach to the problem should be based on well-designed studies in which nutritional and lifestyle factors are described detailed with a large cohort examined.
Declaration of interest
The authors report no conflicts of interest. The authors alone are responsible for the content and writing of the article.
References
- Norlin A, Lindell B, Granberg PO, Lindvall N. Urolithiasis. A study of its frequency. Scand J Urol Nephrol. 1976;10:150–153
- Sierakowski R, Finlayson B, Landes RR, Finlayson CD, Sierakowski N. The frequency of urolithiasis in hospital discharge diagnosis in United States. Invest Urol. 1978;15:438–441
- Johnson CM, Wilson DM, O'Fallon WM, Malek RS, Kurland LT. Renal stone epidemiology: A 25-year study in Rochester, Minnesota. Kidney Int. 1979;16:624–631
- Hoenderop JG, Nilius B, Bindels RJ. Calcium absorbption across epithelia. Physiol Rev. 2005;85:373–422
- Allen LH. Calcium bioavailability and absorption: A review. Am J Clin Nutr. 1982;35:783–808
- Devuyst O, Pirson Y. Genetics of hypercalciuric stone forming disease. Kidney Int. 2007;72:1065–1072
- Pak CY. Medical management of nephrolithiasis. J Urol. 1982;128:1157–1164
- Coe FL, Parks JH, Webb DR. Stone-forming potential of milk or calcium-fortified orange juice in idiopathic hypercalciuric adults. Kidney Int. 1992;41:139–142
- Bushinsky DA. Nephrolithiasis. J Am Soc Nephrol. 1998;9:917–924 (review)
- Levy FL, Adams-Huet B, Pak CY. Ambulatory evaluation of nephrolithiasis: An update of a 1980 protocol. Am J Med. 1995;98:50–59
- Friedman PA. Renal calcium metabolism. In: Alpern RJ, Hebert SC, eds. Seldin and Giebisch's The Kidney Physiology and Pathophysiology. Vol. 2, Chapter 65. Academic Press; 2008:1851--1890
- Pak CY, Holt K. Nucleation and growth of brushite and calcium oxalate in urine of stone-formers. Metabolism. 1976;25:665–673
- Zerwekh JE, Hwang TI, Poindexter J, Hill K, Wendell G, Pak CY. Modulation by calcium of the inhibitor activity of naturally occurring urinary inhibitors. Kidney Int. 1988;33:1005–1008
- Riccardi D, Brown EM. Physiology and pathophysiology of the calcium-sensing receptor in the kidney. Am J Physiol Renal Physiol. 2010;298:F485–F499
- Riccardi D, Hall AE, Chattopadhyay N, et al. Localization of the extracellular Ca21/polyvalent cation-sensing protein in rat kidney. Am J Physiol Renal Physiol. 1998;43:F611–F622
- Ba J, Brown D, Friedman PA. Calcium sensing receptor regulation of PTH-inhibitable proximale tubule phosphate transport. Am J Physiol Renal Physiol. 2004;285:F1233–F1243
- Maiti A, Beckman MJ. Extracellular calcium is a direct effecter of VDR levels in proximal tubule epithelial cells that counter-balances effects of PTH on renal vitamin D metabolism. J Steroid Biochem Mol Biol. 2007;103:504–508
- Egbuna O, Quinn S, Kantham L, et al. The full-length calcium-sensing receptor dampens the calcemic response to 1alpha, 25(OH)2 vitamin D3 in vivo independent of parathyroid hormone. Am J Physiol Renal Physiol. 2009;297:F720–F728
- Canaff L, Hendy GN. Human calcium-sensing receptor gene. Vitamin D response elements in promoters P1 and P2 confer transcriptional responsiveness to 1,25-dihydroxyvitamin D. J Biol Chem. 2002;277:30337–30350
- Riccardi D, Traebert M, Ward DT, et al. Dietary phosphate and parathyroid hormone alter the expression of the calcium-sensing receptor (CaSR) and the Na+-dependent Pi transporter (NaPi-2) in the rat proximal tubule. Pflügers Arch 2000;441:379–387
- Hebert SC, Brown EM, Harris HW. Role of the Ca2+-sensing receptor in divalent mineral ion homeostasis. J Exp Biol. 1997;200:295–302
- Hebert SC. Molecular mechanisms. Semin Nephrol. 1999;19:504–523
- Yu M, Lopez B, DosSantos E, Falck JR, Roman RJ. Effects of 20-HETE on Na transport and Na-K-ATPase activity in the thick ascending loop of Henle. Am J Physiol Regul Integr Comp Physiol. 2007;292:R2400–R2405
- Ikari A, Okude C, Sawada H, et al. Activation of a polyvalent cation-sensing receptor decreases magnesium transport via claudin-16. Biochem Biophys. 2008;1778:283–290
- Vezzoli G, Terranegra A, Soldati L. Calcium-sensing receptor gene polymorphisms in patients with calcium nephrolithiasis. Curr Opin Nephrol Hypertens. 2012;21:355–361
- Wang WH, Lu M, Hebert SC. Cytochrome P-450 metabolites mediate extracellular Ca2+-induced inhibition of apical K+ channels in the TAL. Am J Physiol Cell Physiol. 1996;271:C103–C111
- Boim MA, Ho K, Shuck ME, et al. ROMK inwardly rectifying ATP-sensitive K+ channel. II. Cloning and distribution of alternative forms. Am J Physiol Renal Fluid Electrolyte Physiol. 1995;268:F1132–F1140
- Topala CN, Schoebera JPH, Searchfield LE, et al. Activation of the casensing receptor stimulates the activity of the epithelial Ca channel TRPV5. Cell Calcium. 2009;45:331–339
- Motoyama HI, Friedman PA. Calcium-sensing receptor regulation of PTHdependent calcium absorption by mouse cortical ascending limbs. Am J Physiol Renal Physiol. 2002;283:F399–F406
- Blankenship KA, Williams JJ, Lawrence MS, McLeish KR, Dean WL, Arthur JM. The calcium-sensing receptor regulates calcium absorption in MDCK cells by inhibition of PMCA. Am J Physiol. 2001;280:815–822
- Friedman PA, Coutermarsh BA, Kennedy SM, Gesek FA. Parathyroid hormone stimulation of calcium transport is mediated by dual signaling mechanisms involving protein kinase A and protein kinase C. Endocrinology. 1996;137:13–20
- Hoenderop JG, Bindels RJ. Epithelial Ca2+ and Mg2+ channels in health and disease. J Am Soc Nephrol. 2005;16:15–26
- Vezzoli G, Terranegra A, Rainone F, et al. Calcium-sensing receptor and calcium kidney stones. J Transl Med. 2011;9:201--209
- Renkema KY, Velic A, Dijkman HB, et al. The calcium-sensing receptor promotes urinary acidification to prevent nephrolithiasis. J Am Soc Nephrol. 2009;20:1705–1713
- Farajov EI, Morimoto T, Aslanova UF, et al. Calcium-sensing receptor stimulates luminal K-dependent H excretion in medullary thick ascending limbs of Henle’s loop of mouse kidney. Tohoku J Exp Med. 2008;216:7–15
- Resnick M, Pridgen DB, Goodman HO. Genetic predisposition to formation of calcium oxalate renal calculi. N Engl J Med. 1968;278:1313–1318
- McGeown MG. Heredity in renal stone disease. Clin Sci. 1960;19:465–471
- Curhan GC, Willett WC, Rimm EB, et al. Family history and risk of kidney stones. J Am Soc Nephrol. 1997;8:1568–1573
- Goldfarb DS, Fischer ME, Keich Y, et al. A twin study of genetic and dietary influences on nephrolithiasis: A report from the Vietnam Era Twin (VET) Registry. Kidney Int. 2005;67:1053–1061
- Gräff J, Kim D, Dobbin MM, Tsai LH. Epigenetic regulation of gene expression in physiological and pathological brain processes. Physiol Rev. 2011;91:603–649
- Scillitani A, Guarnieri V, De Geronimo S, et al. Blood ionized calcium is associated with clustered polymorphisms in the carboxyl-terminal tail of the calcium-sensing receptor. J Clin Endocrinol Metab. 2004;89:5634–5638
- Bollerslev J, Wilson SG, Dick IM, Devine A, Dhaliwal SS, Prince RL. Calcium-sensing receptor gene polymorphism A986S does not predict serum calcium level, bone mineral density, calcaneal ultrasound indices, or fracture rate in a large cohort of elderly women. Calcif Tissue Int. 2004;74:12–17
- Yano S, Sugimoto T, Kanzawa M, et al. Association of polymorphic alleles of the calcium-sensing receptor gene with parathyroid hormone secretion in hemodialysis patients. Nephron. 2000;85:317–323
- Vezzoli G, Terranegra A, Arcidiacono T, et al. R990G polymorphism of calcium-sensing receptor does produce a gain-of-function and predispose to primary hypercalciuria. Kidney Int. 2007;71:1155–1162
- Yokoyama K, Shigematsu T, Tsukada T, et al. Calcium-sensing receptor gene polymorphism affects the parathyroid response to moderate hypercalcemic suppression in patients with end-stage renal disease. Clin Nephrol. 2002;57:131–135
- Vezzoli G, Tanini A, Ferrucci L, et al. Influence of calcium-sensing receptor gene on urinary calcium excretion in stone-forming patients. J Am Soc Nephrol. 2002;13:2517–2523
- Jung J, Foroud TM, Eckert GJ, et al. Association of the calcium-sensing receptor gene with blood pressure and urinary calcium in African-Americans. J Clin Endocrinol Metab. 2009;94:1042–1048
- Jung J, Sun B, Kwon D, Koller DL, Foroud TM. Allelic-based gene-gene interaction associated with quantitative traits. Genet Epidemiol. 2009;33:332–343