Abstract
Vitamin E is the generic term for a group of tocopherols and tocotrienols (T3). Hyperlipidemia has been known to cause progressive chronic renal dysfunction (CRD). Several investigators have reported that T3 have hypolipidemic and nephroprotective activity against free radical-related diseases. This study was conducted to determine if T3 as tocotrienol-rich fraction (TRF) from palm oil would protect against lipid-induced CRD in rats. For the induction of atherosclerosis and hyperlipidemia, Wistar male rats were fed an atherogenic diet containing 1.25% cholesterol, 0.5% cholic acid and 21% beef tallow (42.6% calories from fat). The atherogenic diet was given for 14 weeks to induce atherosclerosis. The control rats were given normal rat chow and drug control animals treated with TRF (100 mg/kg bw; orally). The first group was taken as disease control in which the animals were left untreated and given normal rat chow for six weeks, while the second group was treated with 100 mg TRF/kg bw. Atherosclerosis and renal functions were evaluated after six weeks of TRF treatment. Feeding an atherogenic diet to rats for 14 weeks resulted in dyslipidemia and impaired renal functions with decreased glomerular filtration rate. The treatment with TRF significantly reduced dyslipidemia and inhibited the development of CRD caused by atherogenic factors. These findings show that low-dose treatment of TRF may provide significant health benefits in the prevention of lipid-induced CRD. The study suggests that TRF is effective in preventing lipid-induced CRD.
Introduction
Cholesterol is essential to life, widely distributed in animal tissues and required for variety of purposes, including steroid synthesis, bile acid synthesis and as a constituent of cell membrane. It is well established from early studies of dietary cholesterol in animal models, that either high level of plasma low-density lipoprotein cholesterol (LDL-C) and/or low concentration of plasma high-density lipoprotein cholesterol (HDL-C) have detrimental effect on cardiovascular (CV) health. In addition, oxidized LDL is injurious to renal tubular epithelial cells and may contribute to tubulointerstitial disease,Citation1 stimulates the mesangial cells to proliferate and produce excess basement membrane material and could play a role in the pathogenesis of glomerular injury.Citation2
The augmented generation of reactive oxygen species and increased oxidative stress have been implicated in the pathogenesis of renal injury, which may subsequently lead to both functional and structural renal abnormalities.Citation3 Antioxidants such as vitamin E have been shown to attenuate the redox-sensitive mechanisms,Citation2 oxidative modification of LDL,Citation4 ameliorate the chronic renal injury associated with focal segmental glomerulosclerosis in rats,Citation5 improves glomerular filtration rate (GFR), reduces intra-renal inflammation, glomerulosclerosis and prevents renal interstitial fibrosis in hypercholesterolemia.Citation6 Antihyperlipidemic agents are known to lower circulatory lipids and minimize glomerular injury.Citation7
Vitamin E is the generic term for a group of four tocopherols (TOC) and four tocotrienols (T3). T3 qualitatively exhibit the biological activity of α-TOC. TOC and T3 share structural features, namely a common chromanol head and a side chain at the C-2 position. The difference between TOC and T3 is that the former have a saturated phytyl tail, whereas the later possess an unsaturated isoprenoid (farnesyl) side chain. TOC and T3 are further separated into eight individual compounds (α-, β-, γ-, δ- TOC or T3) differing in the number and position of methyl substitutions on the chromanol ring. T3 have been poorly studied as compared to TOC.Citation8 Several investigators reported that T3 have strong antioxidant activity and protects efficiently against some free radical-related diseases.Citation9 At nanomolar concentration, α-T3 prevents stroke-related neurodegeneration.Citation9 We have also reported that T3 attenuates K2Cr2O7-induced renal damage and diabetic nephropathy.Citation10,Citation11 Palm oil (PO) contains more than 70 % T3, which consists of α-, β-, γ- and δ-tocotrienols.Citation12 Although, the pharmacotherapeutic properties of tocotrienol-rich fraction (TRF) from PO are well known, the nephroprotective role of TRF against cholesterol induced nephropathy has not previously been investigated. This study was conducted to determine if the supplement of TRF from PO may reduce the severity of the atherosclerosis and renal dysfunction of the different segments (glomeruli and proximal tubules) of the nephron by the virtues of its antioxidant, hypolipidemic, anti-atherogenic and nephroprotective activities.
Materials and methods
Glutathione, 5,5′-dithiobis-(2-nitrobenzoic acid), thiobarbituric acid (TBA), 2,4-dinitrophenylhydrazine, sulphanilamide (SULF), N-(1-naphthyl) ethylenediamine dihydrochloride (NED), vanadium (III) chloride (VCl3), sodium nitrite, sodium nitrate, p-nitrophenyl-N-acetyl-β-D-glucosaminide, sulfamic acid, 1-chloro-2,4-dinitrobenzene and sulfosalicylic acid were purchased from Sigma-Aldrich Chemicals Pvt. Ltd. (Bangalore, India). Diagnostic kits for the estimation of cholesterol, triglyceride and lipoproteins were purchased from SPAN Diagnostics Ltd. (Surat, India). All chemicals used were of AR grade.
Purification of TRF from PO
TRF was purified from PO in our laboratory as described by Mei et al.Citation13 with minor modifications as mentioned below. The obtained TRF was protected from light and sealed under a nitrogen atmosphere. TRF used in the feeding regimes was isolated from refined edible grade PO by extraction in ethanol. Briefly, 4.0 mL of ethanol was added per gram of PO and stirred for 1 h. After 1 h, the ethanol layer containing the TRF was separated and evaporated at 65 °C under vacuum in a rotary evaporator (BUCHI-R215, Tokyo, Japan) and TRF was recovered. The percent yield of TRF isolated from PO was 5.5 ± 0.37 for 10 separate preparations. The analysis of TRF was determined by high-performance liquid chromatography. It was found to contain 24 mg% α-TOC, 28 mg% α-tocotrienol, 35 mg% γ-tocotrienol and 13 mg% δ-tocotrienol.
Experimental design for the induction of atherosclerosis and hyperlipidemia in animals
Male wistar rats (30), aged between 8 and 12 weeks and weighing 100–120 g, were used in the study. They were kept in the Central Animal House of Jamia Hamdard (Hamdard University) in colony cages at an ambient temperature of 25 ± 2 °C and relative humidity of 45–55% with 12-h light/dark cycles. They had free access to standard rodent pelleted diet (Hindustan Lever Ltd., Bombay, India) and water ad libitum. All procedures using animals were reviewed and approved by the Institutional Animal Ethical Committee that is fully accredited by the Committee for Purpose of Control and Supervision on Experiments on Animals (CPCSEA, Chennai, India).
For the induction of atherosclerosis and hyperlipidemia, 18 rats were fed an atherogenic diet containing 1.25% cholesterol, 0.5% cholic acid, 21% beef tallow (42.6 % calories from fat), 17.3% protein and 46.9% carbohydrate, mixed thoroughly with the powdered chow, making the formulation of standard atherogenic diet (TD.02028; Harlan Teklad, Madison, WI). The atherogenic diet was given for 14 weeks to induce atherosclerosis. Six control rats were given normal rat chow and six drug control animals treated with TRF (100 mg/kg, bw; orally; once daily for six weeks). At the end of 14 weeks, six normal control, six drug control and six atherogenic rats were anaesthetized and sacrificed to measure the zero time point parameters. After the induction of hyperlipidemia, atherogenic diet was withdrawn from rest of the 12 rats. Experiments were performed on two independent groups of six rats each. The first group was taken as disease control, animals in which were left untreated and were given normal rat chow for six weeks, while the second group was treated with 100 mg TRF/kg, bw; orally once daily for six weeks and were also fed normal rat chow. Atherosclerosis and renal functions were evaluated after six weeks of TRF treatment.
Sample collection and tissue preparation
Twenty-four hours before the sacrifice, the rats were housed individually in metabolic cages and allowed water ad libitum. The urine samples were collected over a period of 24 h and maintained under mineral oil to avoid evaporation. Post collection, the urinary volume was measured, and the rats were sacrificed by using sodium pentobarbital anesthesia (60 mg/kg bw, intraperitoneal). Blood samples were obtained by cardiac puncture and serum was separated from cells by centrifugation for 5 min at 4000 rpm. The animals were perfused transcardially through ascending aorta with normal saline. The hearts were removed for freezing section preparation. Frozen sections were then stained with Oil-Red-O and hematoxylin. Briefly, frozen sections of heart (4–6 mm) fixed on glass slides in 10% formalin were washed in running tap water for 1–10 min. Then they were treated with propylene glycol, two changes of 5-min each, followed by staining in Oil-red-O solution for 7 min, again kept in 85% propylene glycol for 3 min. It was then rinsed with distilled water followed by staining in hematoxylin for 1 min. Finally, washed in water and mounted with aqueous mountant. The aortae were rapidly removed and were homogenized with a Polytron homogenizer (Kinematica A.G., Bohemia, NY), in ice-cold phosphate buffer (pH 7.4). The homogenate was centrifuged at 800 × g for 5 min at 4 °C to separate the nuclear debris and was used for estimation of thiobarbituric acid reactive substances (TBARS). The supernatant was further centrifuged at 10,000 × g for 20 min at 4 °C to get the post-mitochondrial supernatant (PMS), which was used for various biochemical assays. The perfusion was continued using 300 mL of 4% paraformaldehyde in 0.1 M phosphate buffer (pH 7.4). The kidneys were then immediately removed and preserved in 10% buffered formalin solution for histopathological examinations.
Assessment of dyslipidemia and atherosclerosis
Serum lipid profile
The level of triglycerides, total cholesterol, HDL-C, LDL-C and triglycerides in rat serum were estimated using commercial assay kits from SPAN Diagnostics Ltd., while, VLDL-C was calculated using the formula given by Friedewald et al.Citation14 The estimations were performed according to the instructions of the manufacturers and the data were expressed in the appropriate units.
Atherogenic index
Atherogenic index (AI) was calculated by using the following formula:Citation15
Evaluation of renal functions
GFR was estimated through the creatinine clearance. Proteinuria is a sensitive indicator of renal damage at the glomerulus or tubular epithelium; therefore, it was relevant to measure its presence. Creatinine was measured in urine and serum by the Jaffe reaction, using commercial assay kits from SPAN Diagnostics Ltd. The estimation was done according to the instructions of manufacturers and expressed as mg/dL. The creatinine clearance was calculated by the standard equations as previously described by Arreola-Mendoza et al.Citation16
Lipid peroxidation (LPO) and LDL oxidation measurement
LPO was measured in the aortal homogenate, based on the formation of TBARS, by the method of Utley et al.Citation17 with some modification. The homogenate (0.25 mL) was pipetted into 15 mm× 100 mm test tubes and incubated at 37 °C in a metabolic shaker for 1 h. An equal volume of homogenate was pipetted into a centrifuge tube, placed at 0 °C and marked at 0 h incubation. After 1 h of incubation, 0.5 mL of 5% (w/v) chilled trichloroacetic acid, followed by 0.5 mL of 0.67% TBA (w/v) was added to each test tube and centrifuged at 1000 × g for 15 min. Thereafter, the supernatant was transferred to fresh test tubes and placed in a boiling water bath for 10 min. The appearance of pink color was noted, and its absorbance was measured at 535 nm in a spectrophotometer (Shimadzu-1601, Kyoto, Japan). The TBARS content was then calculated by using a molar extinction coefficient of 1.56 × 105 M−1 cm−1 and expressed as nmol of TBARS formed min−1 mg−1 of protein. LDL oxidation in plasma was induced by using ascorbate–Fe2+ system. The LDL oxidation products (conjugated dienes) formed were measured and expressed as nmol/min/mL.
Serum and urinary nitric oxide assay
The assay described by Miranda et al.Citation18 was used to reduce nitrate to nitrite by vanadium (III) chloride (VCl3), followed by spectrophotometric analysis of nitrite and nitrate, using Griess reagent. Alternatively, a modification of the method was used. Briefly, VCl3 (200 mg) was dissolved in 1 M HCl (25 mL), and the blue solution stored in dark at 4 °C for less than two weeks. After dissolving NED (0.1%, w/v) in H2O and SULF (2%, w/v) in 5% H3PO4 (v/v), both solutions were stored for several months at 4 °C in the dark. A nitrate standard solution (100 µl) was serially diluted (generally from 100 to 3.125 mM) in duplicate in a 96-well, flat-bottomed, polystyrene microtiter plate.
Nitrite assay
After loading the plate with diluted serum and urine samples (100 µl) in triplicate, the Griess reagent, SULF (50 µl) and NED (50 µl), premixed immediately prior to application to the plate, were added to each well. The absorbance at 540 nm was measured using a plate reader following incubation of 5 min.
Nitrate assay
After loading the plate with diluted serum and urine samples (150 µl) in triplicate, the 150 µl (0.0675 M sulfamic acid 2.5% HCl) was added. The mixture was stirred, and, after 5 min, 300 µl of the Griess reagent followed by 150 µl of reducing agent (VCl3 in 1 M HCl). The absorbance at 540 nm was measured using a plate reader following incubation (usually 30–45 min and 25 °C). Linear regression of mean values for each sample minus blank values was utilized to determine total nitric oxide (NO) concentration according to the calibration curves constructed for standard samples. The values were expressed as nmol/min.
Total protein estimation
Total proteins were evaluated as described by BradfordCitation19 using bovine serum albumin (BSA) as a standard. The PMS of aortal tissues and urine samples were used for the protein estimation. The excretion rates of urinary proteins (Uprot × V) were expressed as µg/min.
Histological examinations
For histological examinations, kidneys from different groups were stained with hematoxylin and eosin (H and E) and Jones periodic acid-Schiff (PAS) for bright-field microscopic evaluation as described by Barrera et al.Citation20 Briefly, thin slices of kidney tissue with cortex and medulla were dehydrated and embedded in paraffin. At least four cross-sections of 4-μm thickness were taken from each kidney and stained with H and E. Following two changes of xylene washes of 2 min, each tissue section was mounted with DPX mountant. The slides were then observed for histopathological changes. A quantitative histological damage was quantified, and microphotographs were taken using an Olympus BX50 microscope system (Olympus, Tokyo, Japan). The image analysis method was used for determination of glomerular area. Digital images of randomly selected glomeruli in H- and E-stained sections were taken. The circumference of each glomerulus was outlined by using the polygonal tracing tool of Image-Pro Plus (Media Cybernetics Inc., Rockville, MD). Glomerular area was computed utilizing the spatial measurement tool calibrated to a stage micrometer.
Statistical analysis
Results are expressed as mean ± standard deviation. The statistical significance of differences between the experimental groups was calculated by ANOVA followed by Tukey–Kramer tests. Analyses were performed using the statistical software Graph Pad InStat v 3 (San Diego, CA). Results were considered significant at p < 0.05.
Results
Dyslipidemia, AI, LDL oxidation and NO assay
Feeding of atherogenic diet for 14 weeks resulted in the development of dyslipidemia, elevation in AI, LPO and LDL oxidation in experimental rats (). There was a significant increase in the levels of plasma triglyceride (2.7-fold). The increase in total serum cholesterol was mainly due to an 8-fold increase in LDL-C levels with a large (39%) decrease in HDL-C (). While we did not observe any remarkable difference in the level of serum VLDL-C of rats fed atherogenic and normal diet, the marker of atherosclerosis, the AI was found to be drastically increased (by 81%) in the animals fed with atherogenic diet as compared to normal diet ().
Table 1. Effect of atherogenic diet and treatment with TRF on the levels of plasma lipids in rats.
Atherogenic diet resulted in a significant increase of 66% in the production of lipid peroxides (TBARS) compared to normal fed rats. Besides this, there was a 55% increase in the oxidation of LDL, which was determined by the formation of conjugated dienes (). The serum NO level was also increased by 44% in atherogenic group as compared to the rats fed normal diet ().
Table 2. Effect of atherogenic diet and treatment with TRF on lipid peroxidation and LDL oxidation in rats.
Table 3. Effect of atherogenic diet and treatment with TRF on the levels of serum nitric oxide in rats.
It was found that atherosclerosis was developed in rats fed with atherogenic diet. In the freezing sections of heart stained with Oil-Red-O and hematoxylin, the endothelium of coronary arteries was found to be bulged with infiltration of lipids, and the plaque/Lumen ratio was larger in rats fed atherogenic diet than in normal rats (, Panels A and B).
TRF supplementation improves dyslipidemia and reduces AI, LPO, LDL-oxidation and serum NO induced by atherogenic diet
The results summarized in show that the treatment of atherogenic rats with TRF significantly decreased the levels of triglycerides by 64%; total serum cholesterol 32%; LDL-C 39%; VLDL-C 48%, while the level of HDL-C was increased by 73%. In addition, the severity of AI decreased by 86%.
The treatment with TRF also resulted in a significant reduction in the magnitude of LPO (by 61%) as well as in the levels of conjugated dienes (by 46%) showing an equivalent prevention of LDL oxidation (). The levels of serum NO was also found to be decreased by 72% (). As evident from (Panel C), the treatment with TRF reduced the severity of atherosclerosis significantly while improving the plaque/Lumen ratio of coronary artery.
Evaluation of renal function
Glomerular filtration rate
Serum creatinine increased sharply (50%) after 14 weeks of feeding with atherogenic diet, and it remained elevated for next four weeks after the withdrawal of atherogenic diet. Treatment with TRF significantly decreased (by 46%) the elevated level of serum creatinine ().
Figure 2. (A) Effect of TRF on serum creatinine in experimental atherogenic rats. Values are mean ± SD. *p < 0.01 versus control; **p < 0.01 versus atherogenic diet (AD) and (B) effect of TRF on creatinine clearance in experimental atherogenic rats. Values are mean ± SD. *p < 0.01 versus control; **p < 0.01 versus atherogenic diet (AD).
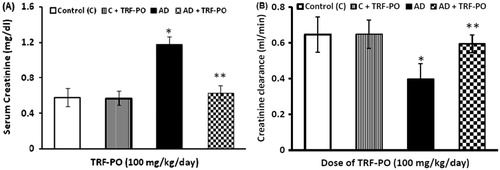
Creatinine clearance was decreased steeply (38%) in the group of rats fed with atherogenic diet for 14 weeks and did not return to the normal level even after the discontinuation of feeding with atherogenic diet. Treatment with TRF had improved the creatinine clearance by 33% and ameliorated the alterations in GFR, indicating the protective effect of TRF against the glomerular dysfunctions caused by atherogenic factors (). Proteinuria, a sensitive indicator of renal damage, can be derived from glomerular or tubular damage. We observed that atherogenic diet elicited proteinuria that was resulted from 14 weeks of feeding with an atherogenic and persisted for another period of four weeks after the withdrawal of atherogenic diet and did not return to control value. The amount of total urinary protein excreted within a period 24 h increased by 53% in the group with highest AI as compared to control animals. Treatment with TRF had significantly (54%) decreased the urinary proteins excretion caused by atherogenic diet ().
Urinary NO
It was found that urinary excretion of increased significantly (25%) in rats fed atherogenic diet as compared to the control group. In TRF supplemented rats the urinary excretion of NO decreased significantly by 23% as compared to the untreated rats ().
Histopathological findings
The histological examination of the H/E and PAS-stained control kidney showed normal architecture of renal tubular epithelial cells and glomeruli (). Significant structural abnormalities were seen in the glomerulus of atherosclerotic rats. The percentage of sclerosed glomeruli was significantly higher, glomeruli showed expanded mesangium and widening of matrix in the group of rats with high level of AI than in all other groups. There was no measurable difference in glomerular area between groups (). The severity of the above-mentioned abnormalities were significantly decreased with treatment of TRF as compared to the untreated group, indicating an effective protection offered by the TRF against the nephritic damage manifested by the atherogenic factors ().
Figure 5. Histology of representative glomeruli at 40X magnification from control rats (A), rats given atherogenic diet (B) and TRF-treated rats (C). Jones PAS-stained glomeruli show expanded mesangium, widening of matrix in atherogenic experimental groups relative to control. The treatment with TRF reduced the widening of matrix and mesangial expansion as compared to untreated rats.
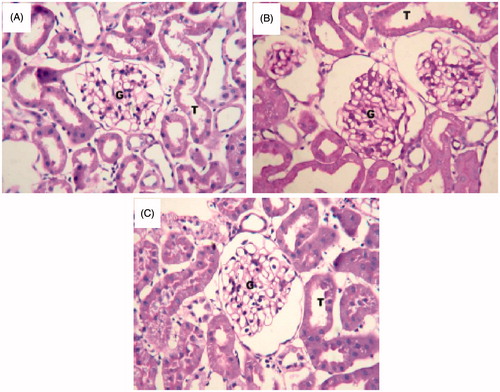
Discussion
The major new findings of this work are dyslipidemia induced by the long-term feeding with atherogenic diet promoted the development of nephropathy and renal dysfunction in rats. Treatment with the TRF from PO had significantly ameliorated the severity of dyslipidemia and improved the renal function disrupted by atherogenic factors. In this study, we investigated the antiatherogenic, nephroprotective and antioxidant effects of TRF from PO, against lipid induced nephropathy in hyperlipidemic rats. Our data clearly show that feeding of atherogenic diet for 14 weeks resulted in dyslipidemia with elevated level of serum triglycerides, total cholesterol and renal dysfunction. Treatment with TRF decreased the plasma triglycerides and total cholesterol. The decrease in total cholesterol was mainly due to reduction in LDL concentration.
These findings were consistent with the previous results reported in animals.Citation21 The rats fed with the TRF have improved the serum HDL-C. In contrast to other lipoproteins, increasing levels of HDL independently reduce CV risk in humans. One important protective mechanism may be HDL’s ability to remove cholesterol from atherosclerotic plaques and cells (reverse cholesterol transport), a process mediated by ApoA-I, the predominant apoprotein in HDL.
The oxidative modification of lipids, particularly oxidation of LDL, has been reported as one of the possible mechanisms leading to CV disease. The antiatherogenic action of antioxidants is commonly linked to the inhibition of lipoprotein oxidation. Currently, LPO is the most valuable maker of oxidative products. In this study, the TRF decreased aortic LPO significantly. This indicated that supplementation of TRF decreased oxidative stress.
Direct evidence for a causative link between in vivo inhibition of lipid oxidation and atherosclerosis is scarce. The data from our study clearly indicate that the hyperlipidemic rats also showed an increase in the levels of lipid peroxides and conjugated dienes, and these data were consistent with the earlier published results.Citation22 Our study demonstrates that, in addition to lowering cholesterol, TRF also helps in inhibiting LPO and oxidation of lipoproteins in hyperlipidemic rats. The antioxidant activity of tocotrienols is rooted in its ability to donate phenolic hydrogens (electrons) to lipid radicals.
In this study, we tried to elucidate that if dyslipidemia facilitate the induction of renal dysfunction. We have shown that rats with dyslipidemia develop glomerular dysfunction, proteinuria and structural changes in kidney. In agreement with our findings, Danda et al.Citation23 reported that high fat diet alone causes significant kidney damage in rats. Our findings suggest that the degree of proteinuria, elevated level of serum creatinine and decrease in the creatinine clearance, which is an ultimate indicator of the GFR, may be adequate markers of renal insufficiency induced by dyslipidemia and atherogenic factors. A proposed mechanism for lipid-induced glomerular injury is the increase in glomerular macrophages with release of a number of chemical mediators and oxidized low-density lipoprotein (LDL)-dependent oxidative stress.Citation24 Hyperfiltration and glomerular hypertension may also play an important role in hypercholesterolemia mediated glomerular injury.Citation25 The treatment with the TRF from PO has reduced the severity of dyslipidemia and the detrimental effect of dyslipidemia on renal function, which might be the outcome of decrease in the formation of oxidized LDL.
Along with the presence of significant glomerular lesions, there was proteinuria evident in the animals fed a high fat diet. The nephrotoxicity of high fat diet does not appear to be specifically related to the particular formulation of the diet used as other investigators have also shown similar kidney pathology with different formulations of high fat diet.Citation24 In this study, the renal dysfunction manifested by the atherogenic factors has relevance with earlier studies. Chronic kidney disease (CKD) patients frequently have a number of additional risk factors associated with CV disease, including proteinuria, electrolyte imbalances, inflammation, increased oxidative stress and altered NO levels.Citation26
Urinary protein loss may increase serum lipoprotein levels. Alternatively, hyperlipidemia may contribute to the progression of CKD by a mechanism similar to atherogenesis.Citation27 Immunostaining of renal biopsies from patients with glomerular disease demonstrated the accumulation of lipoproteins in the glomerular and mesangial cells as well as within the mesangial matrix.Citation28,Citation29
Mesangial cells bind both LDL and oxidized LDL-C (ox-LDL). Hyperlipidemia increases the production of mesangial matrix and drive recruitment of inflammatory cells into the matrix, thus leading to the progression of CKD. High levels of LDL has been shown to increase the expression of fibronectin mRNA and protein, leading to an increase in the mesangial matrix and cell number in cultured mesangial cells.Citation30,Citation31 LDL stimulates the expression of monocyte chemoattractant protein-1 mRNA, leading to increased amounts of secreted monocyte chemotactic activity.Citation31 When bound to the extracellular matrix, ox-LDL has been shown to be cytotoxic.Citation30,Citation32 Both LDL and ox-LDL induce the expression of IL-6 and NF-κB, two factors that are important in the inflammatory and proliferative response of mesangial cells. The transcription factor NF-κB has been linked to inflammatory events associated with glomerulonephritis.Citation33 The nephroprotective action of TRF has been supported by the fact that it reduces the LDL oxidation and mesangial cell expansion or proliferation in the kidney.
These results suggest that TRF supplementation has significant health benefits through the modulation of physiological functions that include various atherogenic lipid profiles and antioxidants in hypercholesterolemia. Therefore, TRF may be a good source of nutrition and may provide good health benefits in hyperlipidemia and related complications.
Acknowledgments
Jamia Hamdard, New Delhi, India, is acknowledged for providing the necessary research facility to carry out this work.
Declaration of interest
The authors declare that there is no conflict of interest.
M.R.K. wishes to acknowledge the College of Pharmacy, King Saud University, Riyadh, KSA, for support during the preparation of manuscript.
References
- Agarwal A, Balla J, Balla G, Croatt AJ, Vercellotti GM, Nath KA. Renal tubular epithelial cells mimic endothelial cells upon exposure to oxidized LDL. Am J Physiol. 1996;271:F814–F823
- Stulak JM, Lerman A, Porcel MR, et al. Renal vascular function in hypercholesterolemia is preserved by chronic antioxidant supplementation. J Am Soc Nephrol. 2001;12:1882–1891
- Chade AR, Rodriguez-Porcel M, Grande JP, et al. Mechanisms of renal structural alterations in combined hypercholesterolemia and renal artery stenosis. Arterioscler Thromb Vasc Biol. 2003;23:1295–1301
- Carr AC, Zhu BZ, Frei B. Potential antiatherogenic mechanisms of ascorbate (vitamin C) and alpha-tocopherol (vitamin E). Circ Res. 2000;87:349–354
- Lee HS, Jeong, JY, Kim BC, Kim YS, Zhang YZ, Chung HK. Dietary antioxidant inhibits lipoprotein oxidation and renal injury in experimental focal segmental glomerulosclerosis. Kidney Int. 1997;51:1151–1159
- Eddy AA. Interstitial fibrosis in hypercholesterolemic rats: Role of oxidation, matrix synthesis, and proteolytic cascades. Kidney Int. 1998;53:1182–1189
- Kasiske BL, O’Donnel MP, Cleary MP, Keane WF. Treatment of hyperlipidemia reduces glomerular injury in obese Zucker rats. Kidney Int. 1988;33:667–672
- Sen CK, Khanna S, Roy S. Tocotrienol: The natural vitamin E to defend the nervous system? Ann NY Acad Sci. 2004;1031:127–142
- Sen CK, Khanna S, Roy S. Tocotrienols: Vitamin E beyond tocopherols. Life Sci. 2006;78:2088–2098
- Khan MR, Siddiqui S, Parveen K, Javed S, Diwakar S, Siddiqui WA. Nephroprotective action of tocotrienol-rich fraction (TRF) from palm oil against potassium dichromate (K2Cr2O7)-induced acute renal injury in rats. Chem Biol Interact. 2010;186:228–238
- Siddiqui S, Khan MR, Siddiqui WA. Comparative hypoglycemic and nephroprotective effects of tocotrienol rich fraction (TRF) from palm oil and rice bran oil against hyperglycemia induced nephropathy in type 1 diabetic rats. Chem Biol Interact. 2010;188:651–658
- Husain K, Francois RA, Hutchinson SZ, et al. Vitamin E delta-tocotrienol levels in tumor and pancreatic tissue of mice after oral administration. Pharmacology. 2009;83:157–163
- Mei HN, Yuen MC, Cheng HC, Hashim MA. Separation of vitamin E (tocopherol, tocotrienol and tocomonoenol) in palm oil. Lipids. 2004;39:1031–1035
- Friedewald WT, Levy RI, Fredrickson DS. Estimation of the concentration of low-density lipoprotein cholesterol in plasma, without use of the preparative ultracentrifuge. Clin Chem. 1972;18(6):499–502
- Deepa PR, Varalakshmi P. Protective effects of certoparin sodium, a low molecular weight heparin derivative, in experimental atherosclerosis. Clin Chim Acta. 2004;339(1–2):105–115
- Arreola-Mendoza L, Reyes JL, Melendez E, et al. Alpha-tocopherol protects against the renal damage caused by potassium dichromate. Toxicology. 2006;218:237–246
- Utley HC, Bernheim F, Hachslein P. Effect of sulfhydryl reagent on peroxidation in microsome. Arch Biochem Biophys. 1967;260:521–531
- Miranda KM, Espey MG, Wink DA. A rapid, simple spectrophotometric method for simultaneous detection of nitrate and nitrite. Nitric Oxide. 2001;5:62–71
- Bradford MM. Rapid and sensitive method for the quantitation of microgram quantities of protein utilizing the principle of protein-dye binding. Anal Biochem. 1976;72:248–254
- Barrera D, Maldonado PD, Medina-Campos ON, Hernandez-Pando R, Ibarra-Rubio ME, Pedraza-Chaverri J. HO-1 induction attenuates renal damage and oxidative stress induced by K2Cr2O7. Free Radic Biol Med. 2003;34:1390–1398
- Iqbal J, Minhajuddin M, Beg ZH. Suppression of 7, 12-dimethylbenz [alpha] anthracene-induced carcinogenesis and hypercholesterolaemia in rats by tocotrienol-rich fraction isolated from rice bran oil. Eur J Can Prev. 2003;12:447–453
- Tanaka M, Nakaya S, Kumai T, Watanabe M, Matsumoto N, Kobayashi S. Impaired testicular function in rats with dietinduced hypercholesterolemia and/or streptozotocin-induced diabetes mellitus. Endocrine Res. 2001;27:109–117
- Danda RS, Habiba NM, Rincon-Choles H, et al. Kidney involvement in a nongenetic rat model of type 2 diabetes. Kidney Int. 2005;68:2562–2571
- Domingues JH, Tang N, Xu W. Studies of renal injury III: Lipid-induced nephropathy in type II diabetes. Kidney Int. 2000;57:92–104
- Anderson S, King AJ, Brenner BM. Hyperlipidemia and glomerular sclerosis. An alternative viewpoint. Am J Med. 1989;87:34N–38N
- Uhlig K, Levey AS, Sarnak MJ. Traditional cardiac risk factors in individuals with chronic kidney disease. Semin Dial. 2003;16:118–127
- Campese VM, Bianchi S, Bigazzi R. Association between hyperlipidemia and microalbuminuria in essential hypertension. Kidney Int. 1999;71:S10–S13
- Takemura T, Yoshioka K, Aya N, et al. Apolipoproteins and lipoprotein receptors in glomeruli in human kidney diseases. Kidney Int. 1993;43:918–927
- Magil AB. Interstitial foam cells and oxidized lipoprotein in human glomerular disease. Mod Pathol. 1999;12:33–40
- Coritsidis G, Rifici V, Gupta S, et al. Preferential binding of oxidized LDL to rat glomeruli in vivo and cultured mesangial cells in vitro. Kidney Int. 1991;39:858–866
- Rovin BH, Tan LC. LDL stimulates mesangial fibronectin production and chemoattractant expression. Kidney Int. 1993;43:218–225
- Gupta S, Rifici V, Crowley S, Brownlee M, Shan Z, Schlondorff D. Interactions of LDL and modified LDL with mesangial cells and matrix. Kidney Int. 1992;141:1161–1169
- Athyros VG, Mikhailidis DP, Papageorgiou AA, et al. Effect of statins versus untreated dyslipidemia on renal function in patients with coronary heart disease: A subgroup analysis of the GREek Atorvastatin and Coronary-heart-disease Evaluation (GREACE) study. J Clin Pathol. 2004;57:728–734