Abstract
Objective: To investigate whether L-carnitine (LC) inhibits eryptosis induced by uremic serum and the related mechanism. Methods: One percent erythrocyte suspension was cultured by three kinds of mediums in vitro, which was included in the control group (Group C, phosphate buffered saline [PBS]), the uremic serum group (Group U, 30% uremic serum + 70% PBS) and the LC group (Group L, 30% uremic serum + 70% PBS + 200 umol/L LC), respectively. Erythrocytes were collected at 24 and 48 h, respectively. Phosphatidylserine (PS) was estimated from Annexin-V-binding and reactive oxygen species (ROS) by flow cytometry, glutathione (GSH) was estimated from Enzyme linked immunosorbent assays (ELISA) by Microplate reader. Results: Eryptosis in Group C increased as the incubating time extended (3.43 ± 0.37 at 24 h, 4.21 ± 0.44 at 48 h). Eryptosis increased in Group U compared with Group C (6.5 1 ± 0.71 at 24 h, p < 0.01; 8.55 ± 0.76 at 48 h, p < 0.01), while decreased in Group L compared with Group U (5.80 ± 0.69 at 24 h, p < 0.05; 7.87 ± 0.76 at 48 h, p < 0.05). Meanwhile, ROS of erythrocytes increased in Group U compared with Group C (33.12 ± 1.61 versus 14.83 ± 2.22 at 24 h, p < 0.01; 42.06 ± 1.81 versus 20.94 ± 1.78 at 48 h, p < 0.01), and GSH decreased in Group U compared with Group C (25.66 ± 0.32 versus 31.27 ± 0.38 at 24 h, p < 0.01; 8.53 ± 0.59 versus 17.29 ± 0.54 at 48 h, p < 0.01). ROS of erythrocytes decreased in Group L compared with Group C (26.29 ± 1.69 at 24 h, p < 0.01; 36.21 ± 2.00 at 48 h, p < 0.01). GSH increased in Group L compared with Group U (27.54 ± 0.60 at 24 h, p < 0.01; 15.18 ± 0.42 at 48 h, p < 0.01). Conclusions: LC inhibits eryptosis induced by uremic serum, which possibly relates to oxidative stress in part.
Introduction
Anemia is one of the most frequent complications of kidney failure and a common cause of morbidity in dialysis patients. The multifactorial pathogenesis of renal anemia is well known. The main etiologies are inadequate production of erythropoietin as well as absolute or relative iron deficiency, and shortened life span of the erythrocyte. Renal anemia can be successfully treated by the use of erythropoietin and iron in most hemodialysis patients. However, a significant number of patients remain hypo-responsive to these kinds of therapy, which is called refractory anemia with hemoglobin less than target levels. Several studies have shown that L-carnitine (LC) supplementation has a beneficial effect on renal anemia,Citation1–3 but the underlying mechanisms have not yet been completely elucidated.
LC is a cofactor required for transporting of long-chain fatty acids into the mitochondrial matrix, where they undergo oxidation for cellular energy production and removal of fat metabolism by-products and toxic compounds from the cells. LC has potential function of anti-inflammation, anti-apoptosis and anti-oxidative, which is often used in the treatment of heart failure and secondary LC insufficiency. Previous studies focused on the usefulness of erythrocyte membrane stability or the changed osmotic fragility after LC supplementation.Citation4–7 Further studies are needed to explore the anti-oxidative characteristic of LC, which could inhibit eryptosis in patients with uremia.
Based on these observations, the aim of the present study was to examine the effect of exogenous LC supplementation on (i) the expression of phosphatidylserine (PS) on membranes of erythrocytes; (ii) the variation of reactive oxygen species (ROS) (the marker of oxidative products) and glutathione (GSH) (the marker of anti-oxidant products) of erythrocytes in vitro. Our study will observe the protective effect of LC on erythrocytes in uremic serum in vitro and explore its mechanism, which may provide a new idea and a new way for curing renal anemia in clinic.
Materials and methods
Materials
PBS (phosphate buffered saline), 2,7-dichlorofluorescin diacetate (DCFH-DA) was purchased from Sigma (Beijing China). Glutathione detection kit was purchased from BiyunTian, Shanghai, China. Annexin V-FITC apoptosis detection kit was purchased from Kaiji, Nanjing, China. Sterile operating platform was produced by ESCO Class II. Cell incubator was MCO-18AIC. Flow cytometer was BD FACSCalibur. Microplate reader was ANTO2010. LC injection was purchased from Sigma-Tau Industrie Farmaceutiche (1 g/5 mL).
Study population
Serum were obtained from end-stage renal disease patients on chronic maintenance hemodialysis, presenting at the dialysis center of the Second Hospital of Shandong University, who had been on regular dialysis treatment for more than 1 year. Blood pressure was controlled below 150/90 mmHg. The patients with diabetes mellitus, obvious infection or severe heart failure were excluded. The patients included were eight cases with glomerulonephritis, seven with primary hypertension and five with drug-induced renal injury in the study (male 12 and female eight, aged 42.3 ± 2.1 years). Blood creatinine was 873.34 ± 23.56 umol/L and urea nitrogen was 25.65 ± 12.95 mmol/L in the mixed serum.
The study protocol was approved by the Ethics Committee of the Second Hospital of Shandong University, and all subjects gave informed written consent.
Uremic serum preparation
Blood samples (6 mL for each patient) obtained from HD patients following informed consent were collected into evacuated tubes before the start of the hemodialysis session. After centrifugation for 5 min at 30g in 30 min, serum was carefully removed into Eppendorf tubes and kept in −20 °C for use in 10 days. All the mixed serum was filtrated by 0.22 um filter membrane to sterilization before the test. Uremic serum solution was prepared as required.
Healthy erythrocyte incubation in vitro
Six healthy volunteers with normal hepatic and renal function donated erythrocytes for the study. The average age was 33 ± 2.5 years old. Ten milliliter venous blood was obtained into tubes with ethylenediaminetetraaceticacid (EDTA) anticoagulation. After centrifugation for 15 min at 500g, erythrocytes were carefully removed into another tube and washed three times with PBS. Then erythrocytes were suspended in 100 uL of PBS at 1% hematocrit. The suspension liquid was injected into 12 pore culture plates and incubated in 37 °C, 5% CO2 incubator.
The suspension liquid of erythrocytes was exposed to different mediums. According to mediums, it was divided into three groups.
Group C: The culture medium was PBS.
Group U: The culture medium was 30% uremia serum and 70% PBS.
Group L: The culture medium was 30% uremia serum and 70% PBS and LC (the final concentration was 200 umol/L).
At 24 and 48 h, erythrocytes were collected and centrifuged, then washed by PBS at least two times. All of the erythrocytes were examined in 1 h. In Group L, LC was added and incubated for 2 h in advance. After centrifugation and washed at least two times, erythrocytes were incubated with required concentration with the other groups.
PS expression
Annexin V-FITC is able to label the externalized PS on the outer layer of cell membrane. According to the manufacturer’s instructions, cells were loaded with Annexin V-FITC (10 uL) and propidium iodide (PI, 5 uL) under protection from light. After addition of 400 uL of the binding buffer, the cells were analyzed in a flow cytometer equipped with a 488 nm argon laser (FACSort, Becton–Dickinson). Data were analyzed with the equipped software.
Intracellular ROS
Fluorescent probe Dichlorodihydrofluorescein diacetate (DCFH-DA) was diluted by PBS to final concentration 10 umol/L, then incubated for 20 min in order to be fully combined. DCFH-DA responses to ROS and produces 2′,7′-dichlorodihydrofluorescein (DCF) with fluorescent characteristic. Erythrocytes were washed three times by PBS to wipe off DCFH-DA outside the cells. The cells were analyzed in a flow cytometer equipped with a 488 nm argon laser (FACSort, Becton–Dickinson). Data were analyzed with the equipped software.
Intracellular glutathione (GSH)
Microplate reader examined the intracellular GSH according to the demand.
Three parallel contrast holes were equipped at one time. Since different erythrocyte specimens used in distinct experiments are differently susceptible to the triggers of eryptosis, the same erythrocyte specimens have been used for control and experimental conditions. The same test was repeated six times. The end value was averaged at the same time.
Statistics
All data were dealt with SPSS 12.0. Results were expressed as arithmetic means ± standard error (mean ± SEM). As indicated in the figure legends, statistical analysis was made using ANOVA with LSD as the post-test. Least significant difference with p < 0.05 was considered as the criterion for statistical significance.
Results
1. Apoptosis of healthy erythrocyte incubated in different culture mediums: Eryptosis increased with the incubating time extension (3.43 ± 0.37 at 24 h, 4.21 ± 0.44 at 48 h). After injecting uremic serum, eryptosis increased (6.51 ± 0.71 at 24 h, p < 0.01; 8.55 ± 0.76 at 48 h, p < 0.01) in Group U. When injecting LC and uremia serum simultaneously, eryptosis decreased (5.80 ± 0.69 at 24 h, p < 0.05; 7.87 ± 0.76 at 48 h, p < 0.05) in Group L. The data are shown in , and .
Figure 1. The effect of different mediums on PS, ROS and GSH of healthy erythrocytes. The arithmetic means ± standard error (mean ± SEM, n = 6) of PS, ROS and GSH following incubation for 24 and 48 h in Group C, U and L, respectively.
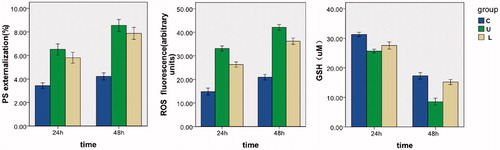
Figure 2. Original histogram of Annexin-V-binding of erythrocytes following exposure for 24 and 48 h to Group C, U and L. Blue area represents the quantity of Annexin-V-binding of erythrocytes. Fig.012 is Annexin-V-binding erythrocytes at 0 h. Fig.006, 014 and 004 are Annexin-V-binding erythrocytes at 24 h in Group C, U and L, respectively. Fig.015, 016 and 024 are Annexin-V-binding erythrocytes at 48 h in Group C, U and L, respectively.
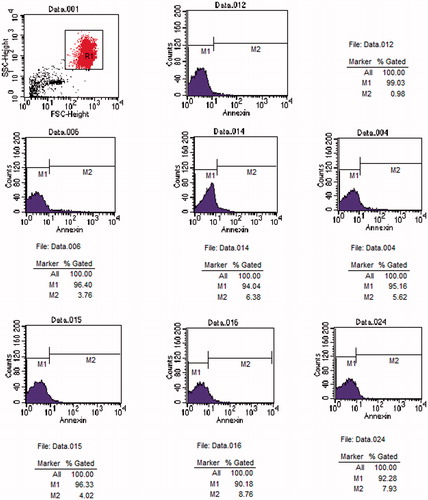
Table 1. The results of PS, ROS, GSH in different group (mean ± SEM).
2. ROS and GSH of healthy erythrocyte incubated in different culture mediums: ROS increased with the incubating time extension (14.83 ± 2.22 at 24 h, 20.94 ± 1.78 at 48 h), GSH decreased (31.27 ± 0.38 at 24 h, 17.29 ± 0.54 at 48 h). After injecting uremic serum, ROS increased (33.12 ± 1.61 at 24 h, p < 0.01; 42.06 ± 1.81 at 48 h, p < 0.01) and GSH decreased (25.66 ± 0.32 at 24 h, p < 0.01; 8.53 ± 0.59 at 48 h, p < 0.01) in Group U. When injecting LC and uremia serum simultaneously, ROS decreased (26.29 ± 1.69 at 24 h, p < 0.01; 36.21 ± 2.00 at 48 h, p < 0.01) and GSH increased (27.54 ± 0.600 at 24 h, p < 0.01; 15.18 ± 0.42 at 48 h, p < 0.01) in Group L. The data are shown in , and .
Figure 3. Original histogram of ROS of erythrocytes following exposure for 24 and 48 h to Group C, U and L. Blue area represents the quantity of ROS. Fig.001 is ROS at 0 h. Fig.003, 014 and 007 are ROS at 24 h in Group C, U and L, respectively. Fig.023, 035 and 048 are ROS at 48 h in Group C, U and L, respectively.
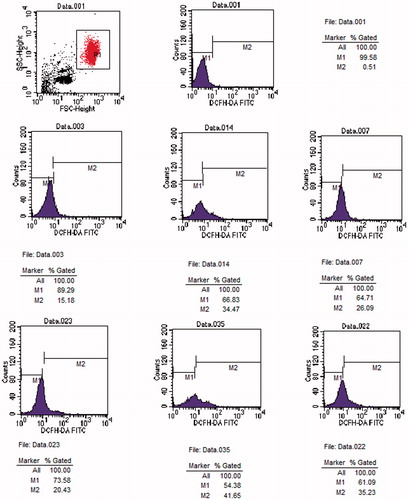
3. The correlation analysis between oxidative stress indexes and eryptosis: The result showed that PS was positively correlated with ROS (r = 0.87 at 24 h, p < 0.01; r = 0.92 at 48 h, p < 0.01). GSH was negatively correlated with PS (24 h r = −0.79 at 24 h, p < 0.01; r = −0.69 at 48 h, p < 0.01), as well as ROS (r = −0.83 at 24 h, p < 0.01; r = −0.80 at 48 h, p < 0.01). The data are shown in .
Table 2. The correlation analysis of PS, ROS and GSH.
Discussion
Anemia occurs frequently in patients with chronic kidney disease (CKD), especially in the end stages, which directly influences the quality of life and decreases the survival rate of patients in maintained renal replacement.Citation8 It is very important for treatment of renal anemia effectively. In this study, we focused our interest on the study of eryptosis triggered by uremic serum and alleviated by LC. Our study was to incubate erythrocytes in uremic serum or LC supplements in vitro, then observe eryptosis, ROS and GSH at 24 and 48 h. We conjectured LC decreases eryptosis by rectifying oxidative imbalance. Such results indicate that oxidative stress may be involved in the protective mechanisms.
It is well known that mature erythrocytes do not contain organelles. So it is unable to synthesize protein or repair itself. It is more prone to eryptosis. Compared with other cells, the life of erythrocyte is limited and vulnerable to the external environment in the process of survival. Much evidence points out different environmental conditions, such as diabetes, sepsis and the Mediterranean anemia, chronic renal failure and other diseases may induce eryptosis.Citation9 In a number of clinical trials, erythrocyte was confirmed that the expectancy was decreased from normal 120 days to about 90 days in uremia.Citation10 It is also one of the important reasons of renal anemia, including the occurrence of eryptosis frequent. It is one of the characteristics of eryptosis that outer layer of erythrocyte membrane expresses PS originally located in the inner layer. The appearance of PS on erythrocytes means the beginning of eryptosis. At the same time, PS activates macrophage recognitionCitation11 and phagocytosis. It also causes adhesionCitation12 of erythrocytes or between erythrocyte and endothelial cell, which can activate coagulation. In other words, PS expression on erythrocytes will stimulate blood clotting and trigger thrombosis. The serum of patients in chronic renal failure can induce eryptosis, and eryptosis is reversibleCitation13 in the process. This suggested that it should be an effective method for the treatment of anemia by inhibiting or reversing expression of PS.
In our study, we chose stable hemodialysis patients and simulated uremia environment in vitro. The results showed that the apoptosis was increased in healthy erythrocytes with the incubation extension, and ROS increased, but GSH decreased. After erythrocytes were cultured in the uremia serum, eryptosis was found increasingly significant. These were consistent with the results of Bonomini et al.,Citation13 which illuminates that internal environment of stable hemodialysis patients may induce eryptosis compared with healthy population. Of course, uremic toxins have been proved to stimulate eryptosis.Citation14 Meanwhile, both the increase of ROS and decrease of GSH showed that oxidative stress might be involved in the mechanism of eryptosis.
As a necessary material for long chain fatty acyl-CoA metabolism, LC also has the potentials of anti-oxidation, anti-apoptosis, anti-inflammatory and other pharmacological characteristics. Previous studies have confirmed that LC has obvious therapeutic effect for renal anemia. It is reported that LC can inhibit cell apoptosisCitation15,Citation16 and extend the life span of erythrocyte in maintained hemodialysis patients.Citation17 Arduini et al.Citation17 used Cr51 marked erythrocyte in his clinical study and found that the life span of erythrocyte were significantly prolonged 8.5 days after intravenous LC supplement for 24 weeks in maintenance hemodialysis patients. We intervened erythrocyte in uremic serum by LC, and found that LC decreased eryptosis. The conclusion of Bonomini et al. was confirmed that the process of eryptosis is reversible. Eryptosis could be reduced by restore to normal living environment or by some drugs. It also explained Arduini et al.’s results that LC inhibits eryptosis by reducing the expression of PS to prolong the life span of erythrocytes.
Many studies have shown that not only the decreased production of erythropoietin and lack of iron, but also the internal environment of oxidative stress products lead to renal anemia in uremia.Citation18 Excessive oxidative stress increases eryptosis, which is one of the causes of uremic anemia.Citation19 Maintenance hemodialysis patients must contact dialysis materials and dialysis fluid for several times a week. These processes result in a large number of ROSCitation20,Citation21 produced, including in the treatment of anemia. These reasons imply that the application of anti-oxidant drugs may contribute to renal anemia. ROS and GSH keep balance in the oxidative stress of erythrocyte and can maintain its normal morphology and function. Our results showed that eryptosis was decreased in Group L than in Group U, and the decreased ROS and increased GSH were found in Group L. These results suggest that the character of anti-oxidative was contributed to the improvement of renal anemia. It indicates that LC is an effective radical scavenger for erythrocyte in uremic serum which protects erythrocytes by alleviating oxidative stress.
Apart from the ability to act as transport of fatty acid across the mitochondrial membrane, it has been reported that LC is beneficial to various diseases due to anti-oxidative stress characteristics, such as drug-induced renal injury (cisplatin,Citation22 carboplatin,Citation23 Adriamycin,Citation24 glycerin,Citation25 gentamicin,Citation26 cyclophosphamide,Citation27 etc.) and multiple organ damage by the contrast agent.Citation28 Our research also manifested that the LC reduced eryptosis by inhibiting oxidative stress. It is necessary to probe the best concentration and effective acting time. Our study provides a new treatment idea for the prevention and treatment of renal anemia, but it still need further research on the clinical application. In conclusion, our study suggests that LC inhibits eryptosis in the uremic model and the effect is at least in part due to anti-oxidative characteristic of LC.
Acknowledgments
We thank research assistant Junli Liu (Department of Central Laboratory, the Second Hospital of Shandong University, Jinan, Shandong Province, China) for her elaborately measure in the trial.
Declaration of interest
The authors declare no conflicts of interest. This work was supported by Natural Science Foundation of Shandong Province (ZR2012L35), China.
References
- Matsumoto Y, Amano I, Hirose S, et al. Effects of L-carnitine supplementation on renal anemia in poor responders to erythropoietin. Blood Purif. 2001;19:24–32
- Wanic-Kossowska M, Kazmierski M, Pawliczak E, et al. Combined therapy with L-carnitine and erythropoietin of anemia in chronic kidney failure patients undergoing hemodialysis. Pol Arch Med Wewn. 2007;117(1–2):14–19
- Kadiroglu AK, Yilmaz ME, Sit D, et al. The evaluation of postdialysis L-carnitine administration and its effect on weekly requiring doses of rHuEPO in hemodialysis patients. Renal Fail. 2005;27:367–372
- Nikolaos S, George A, Telemachos T, et al. Effect of L-carnitine supplementation on red blood cells deformability in hemodialysis patients. Renal Fail. 2000;22:73–80
- Matsumura M, Hatakeyama S, Koni I, et al. Correlation between serum carnitine levels and erythrocyte osmotic fragility in hemodialysis patients. Nephron. 1996;72:574–578
- Arduini A, Rossi M, Mancinelli G, et al. Effect of L-carnitine and acetyl-L-carnitine on the human erythrocyte membrane stability and deformability. Life Sci. 1990;47:2395–2400
- Labonia WD, Morelli Jr OH, Gimenez MI, et al. Effects of L-carnitine on sodium transport in erythrocytes from dialyzed uremic patients. Kidney Int. 1987;32:754–759
- Locatelli F, Pisoni RL, Combe C, et al. Anaemia in hemodialysis patients of five European countries: Association with morbidity and mortality in the Dialysis Outcomes and Practice Patterns Study (DOPPS). Nephrol Dial Transplant. 2004;19:121–132
- Lang F, Lang E, Föller M. Physiology and pathophysiology of eryptosis. Transfus Med Hemother. 2012;39(5):308–314
- Sato Y, Mizuguchi T, Shigenaga S, et al. Shortened red blood cell lifespan is related to the dose of erythropoiesis-stimulating agents requirement in patients on hemodialysis. Ther Apher Dial. 2012;16(6):522–528
- Bonomini M, Sirolli V, Reale M, et al. Involvement of phosphatidylserine exposure in the recognition and phagocytosis of uremic erythrocytes. Am J Kidney Dis. 2001;37:807–814
- Bonomini M, Sirolli V, Gizzi F, et al. Enhanced adherence of human uremic erythrocytes to vascular endothelium: Role of phosphatidylserine exposure. Kidney Int. 2002;62:1358–1363
- Bonomini M, Sirolli V, Settefrati N, et al. Increased erythrocyte phosphatidylserine exposure in chronic renal failure. J Am Soc Nephrol. 1999;10:1982–1990
- Ahmed MS, Langer H, Abed M, et al. The uremic toxin acrolein promotes suicidal erythrocyte death. Kidney Blood Press Res. 2013;37(2–3):158–167
- Kitamura Y, Satoh K, Satoh T, et al. Effect of L-carnitine on erythroid colony formation in mouse bone marrow cells. Nephrol Dial Transplant. 2005;20(5):981–984
- Matsumura M, Hatakeyama S, Koni I, et al. Effect of L-carnitine and palmitoyl-L-carnitine on erythroid colony formation in fetal mouse liver cell culture. Am J Nephrol. 1998;18(5):355–383
- Arduini A, Bonomin M, Elaine J, et al. Effect of L-carnitine administration on erythrocyte survival in hemodialysis patients. Nephrol Dial Transplant. 2006;21(9):2671–2672
- Mircescu G. Oxidative stress: An accomplice to uremic toxicity? J Ren Nutr. 2006;16(3):194–198
- Usberti M, Gerardi G, Bufano G, et al. Effects of erythropoietin and vitamin E-modified membrane on plasma oxidative stress markers and anemia of hemodialyzed patients. Am J Kidney Dis. 2002;40(3):590–599
- Agarwal R, Vasavada N, Sachs NG, et al. Oxidative stress and renal injury with intravenous iron in patients with chronic kidney disease. Kidney Int. 2004;65(6):2279–2289
- Nagababu E, Gulyani S, Earley CJ, et al. Iron-deficiency anemia enhances red blood cell oxidative stress. Free Radic Res. 2008;42(9):824–829
- Yürekli Y, Unak P, Yenisey C, et al. L-carnitine protection against cisplatin nephrotoxicity in rats: Comparison with amifostin using quantitative renal Tc 99m DMSA uptake. Mol Imaging Radionucl Ther. 2011;20(1):1–6
- Arafa HM. Carnitine deficiency aggravates carboplatin nephropathy through deterioration of energy status, oxidant/anti-oxidant balance, and inflammatory endocoids. Toxicology. 2008;254(1–2):51–60
- Boonsanit D, Kanchanapangka S, Buranakarl C. L-carnitine ameliorates doxorubicin-induced nephrotic syndrome in rats. Nephrology (Carlton). 2006;11:313–320
- Ustundag S, Sen S, Yalcin O. L-carnitine ameliorates glycerol-induced myoglobinuric acute renal failure in rats. Ren Fail. 2009;31(2):124–133
- Al-Shabanah OA, Aleisa AM, Al-Yahya AA. Increased urinary losses of carnitine and decreased intramitochondrial coenzyme A in gentamicin-induced acute renal failure in rats. Nephrol Dial Transplant. 2010;25:69–76
- Sayed-Ahmed MM. Progression of cyclophosphamide-induced acute renal metabolic damage in carnitine-depleted rat model. Clin Exp Nephrol. 2010;14(5):418–426
- Boyacioglu M, Turgut H, Akgullu C, et al. The effect of L-carnitine on oxidative stress responses of experimental contrast-induced nephropathy in rats. Vet Med Sci. 2014;76(1):1–8