Abstract
Chronic exposure of a naturally occurring metal arsenic leads to renal and hepatic diseases. Naringin, a flavanone glycoside, possesses anti-inflammatory and anti-oxidant potential. The aim of this investigation was to evaluate the protective effect of naringin against arsenic-induced renal and hepatic toxicity in rats. Renal and hepatic toxicity was induced in rats by sodium arsenite (5 mg/kg, p.o.). Rats were treated orally with either vehicle or naringin (20, 40, and 80 mg/kg) or Coenzyme Q10 (10 mg/kg) for 28 days. Various biochemical, histological, and molecular biomarkers were assessed in kidney and liver. Treatment with naringin (40 and 80 mg/kg) significantly and dose-dependently restored (p < 0.01 and p < 0.001) altered levels of kidney (serum creatinine, urine creatinine, BUN, uric acid, and creatinine clearance) and liver function test (AST and ALT) induced by sodium arsenite. Elevated levels of oxido-nitrosative stress in renal and hepatic tissue was significantly and dose-dependently decreased (p < 0.01 and p < 0.001) by naringin (40 and 80 mg/kg) treatment. It significantly and dose-dependently down-regulated (p < 0.01 and p < 0.001) renal KIM-1, Caspase-3, TGF-β, and TNF-α mRNA expression. Histopathological alteration induced in kidney and liver by sodium arsenite was reduced by naringin (40 and 80 mg/kg) treatment. In conclusion, naringin treatment ameliorates arsenic-induced renal and hepatic damage in rats due its antioxidant and anti-inflammatory properties via down-regulation of elevated oxido-nitrosative stress, KIM-1, Caspase-3, TGF-β, and TNF-α levels.
Introduction
Inorganic arsenic as trivalent arsenite (As3+) or pentavalent arsenite (As5+) is a potent naturally occurring toxic agent possesses multiple toxic effects on the animal as well as on human health standpoints. Drinking water is the main source through which arsenic exposure occurs in most of the developing country like India, China, Mexico, and Bangladesh.Citation1 Arsenic compounds have high value in an agricultural application such as fungicides, insecticides, herbicides, algaecides, and wood preservatives.Citation1 Arsenic indulge in various enzymatic reactions, affects probably whole organ system in human and other animals.Citation2 Due to inorganic arsenic toxicity concerns with various other form of internal cancers international agency for research on cancer classify arsenic as a group 1 human carcinogen.Citation3 In addition, arsenic exposure possesses non-cancerous effects like kidney diseases, liver diseases, lung disease, hypertension, diabetes, anaemia, cardiovascular diseases, and neurological disorder.Citation4,Citation5 Investigation at the cellular and molecular levels reveals that arsenic exposure begets ROS via cellular oxidation–reduction reaction that ultimately causes oxidative stress.Citation6 ROS in associated with cellular biomolecule responsible for lipid peroxidation, damage protein, enzyme as well as DNA in cells, hinder mitosis, and aggravate apoptosis.Citation7
It has been reported that liver and kidney are ascribed as highly prone organs to deleterious manifestation by toxins.Citation8–11 Arsenic accumulation and excretion occur in kidney, long term exposure of arsenic havoc the pathology of kidney which cause acute renal failure characterized by nephritis, nephritic syndrome, and nephrosis.Citation8,Citation12 A study reveals that metabolic conversion of arsenic into methylated products occurs in the liver.Citation13 Previously, it has been claimed that arsenic causes oxidative stress responsible for the apoptosis, increased cell proliferation, genomic flexibility, and deviant estrogen signaling in association with liver toxicity.Citation14 Oral arsenic exposure leads changes in hepatocyte mitochondria, i.e., condensation of mitochondrial matrix and distortion of mitochondrial transmembrane potential.Citation15 It is also well acknowledged that prolong arsenic exposure cause lung cancer.Citation16 Evidence from the several study show that arsenic-exposed subjects also undergoes nervous system impairment such as depression, mood swing, impaired facial recognition, convulsion, seizure, headache, and decreased locomotor activity.Citation16 Arsenic exposure plays a pivotal role in the pathogenesis of cardiac tissue that contributes cardiac arrhythmias, myocardial injury, and cardiomyopathy.Citation17 The molecular mechanism through which arsenic intoxication on male reproductive organs still not illuminated. Although some evidence reveals that oxidative stress responsible for the inflammation with the production of pro-inflammatory cytokines involve in arsenic-induced organ damage.Citation18
Naringin (4′,5,7-trihydroxyflavone 7-rhamnoglucoside) is a bioflavonoid predominantly present in grapefruits and related citrus species.Citation19 After oral ingestion of naringin, enzymes like α-rhamnosidase, and β-glucosidase hydrolyzed it into the absorbable form of naringenin.Citation20 Naringin plays a significant role as metal-chelating, antioxidant, anti-apoptotic, and free radical scavenging properties.Citation21 Various evidence support that naringin possess numerous pharmacological and therapeutic action, antimutagenic, anti-inflammatory, anticancer, antihypertensive, neuroprotective, wound healing, antihyperlipidemic, and anticancer potential.Citation22–29 Metabolized product of naringin, i.e., naringenine has a promising nephroprotective and hepatoprotective actions.Citation30,Citation31 Naringin crosses the blood–brain barrier thus it imparts ameliorative effect on central nervous system diseases.Citation32–34 A previous study revealed that naringin exhibited potential cardioprotective effect via modulation of membrane stabilizing and antioxidant properties.Citation35 A previous study carried in out our laboratory showed that naringin possesses protective action against sodium arsenite-induced testicular toxicity via inhibition of TNF-α and IL-1β.Citation36 Hence, the aim of this study was to investigate the protective effect of naringin against arsenic-induced renal and hepatic toxicity via assessment of biochemical, histological, and molecular biomarkers.
Materials and methods
Animals
Adult male Sprague–Dawley rats (200–250 g) were purchased from the National Institute of Biosciences, Pune (India). They were maintained at 24 ± 1 °C, with a relative humidity of 45–55% and 12:12 h dark/Light cycle. The animals had free access to standard pellet chow (Pranav Agro-industries Ltd., Sangli, India) and water throughout the experimental protocol. All experiments were carried out between 09:00 and 17:00 h. The experimental protocol was approved by the Institutional Animal Ethics Committee (IAEC) of Poona College of Pharmacy, Pune and performed in accordance with the guidelines of Committee for Control and Supervision of Experimentation on Animals (CPCSEA), Government of India on animal experimentation.
Drugs and chemicals
Naringin was purchased from Sigma Chemical Co. (St. Louis, MO). Coenzyme Q10 was supplied by Medicines Pvt. Ltd. (Mumbai, India) whereas sodium arsenite was purchase from Otto Chemicals, India. 1,1′,3,3′-Tetraethoxypropane, crystalline beef liver catalase, and 5,5′-dithiobis (2-nitrobenzoic acid) were purchased from S.D. Fine Chemicals, Mumbai, India. Sulphanilamides, naphthylamine diamine HCl, and phosphoric acid were obtained from Loba Chemie Pvt. Ltd., Mumbai, India. Total RNA Extraction kit and One-step RT-PCR kit was purchased from MP Biomedicals India Private Limited, India.
Experimental design
Rats were randomly divided into following groups (n = 6) as follows:
Group I: Normal [N]: Received double distilled water (5 mL/kg, p.o.) for 28 days.
Group II: Arsenic + Coenzyme Q10 (10 mg/kg) [As + CoQ10 (10)]: Received sodium arsenite (10 mg/kg, p.o.) and treated with Coenzyme Q10 (10 mg/kg, p.o.) for 28 days.
Group III: Arsenic Control [As]: Received sodium arsenite (5 mg/kg, p.o.) and treated with distilled water (5 g/kg, p.o for 28 days) for 28 days.
Group IV: Arsenic + Naringin (20 mg/kg) [As + N (20)]: Received sodium arsenite (5 mg/ kg, p.o.) and treated with naringin (20 mg/kg, p.o.) for 28 days.
Group V: Arsenic + Naringin (40 mg/kg) [As + N (40)]: Received sodium arsenite (5 mg/ kg, p.o.) and treated with naringin (40 mg/kg, p.o.) for 28 days.
Group VI: Arsenic + Naringin (80 mg/kg) [As + N (80)]: Received sodium arsenite (5 mg/ kg, p.o.) and treated with naringin (80 mg/kg, p.o.) for 28 days.
Serum and urinary biochemistry
The serum was separated by centrifugation using Eppendorf Cryocentrifuge (model No. 5810, Germany), maintained at 4 °C and run at a speed of 7000 rpm for 15 min. Serum creatinine, urine creatinine, BUN, Uric acid, ALT, and AST were measured by spectrophotometer (UV–Visible spectrophotometer, Jasco V-530, Japan) using reagent kits according to procedure provided by manufacturer (Accurex Biomedical Pvt. Ltd., Mumbai, India).
Biochemical estimation
Tissue homogenate preparation
All animals were sacrificed at the end of the study, i.e., on the 29th day, kidney and liver were immediately isolated. Tissue homogenates were prepared with 0.1 M Tris-HCl buffer (pH 7.4) and supernatant of homogenates was employed to estimate superoxide dismutase (SOD), reduced glutathione (GSH), lipid peroxidation (MDA content), nitric oxide (NO content), and Na–K–ATPase as described previously.Citation38–43
Determination of KIM-1, Caspase-3, TGF-β, and TNF-α by reverse transcriptase PCR in kidney
RNA isolation
The levels of mRNA were analyzed in kidney tissue using a reverse transcription (RT)-PCR as described previously.Citation44–47 Single-stranded cDNA was synthesized from 5 µg of total cellular RNA using reverse transcriptase kit (MP Biomedicals India Private Limited, India) as described previously.Citation44 The primer sequence for kidney injury molecule-1 (KIM-1), Caspase-3, transforming growth factor-beta (TGF-β), tumor necrosis factor-alpha (TNF-α), and β-actin are presented in . Amplification of β-actin served as a control for sample loading and integrity. PCR products were detected by electrophoresis on a 1.5% agarose gel containing ethidium bromide. The size of amplicons was confirmed using a 100-bp ladder as a standard size marker. The amplicons were visualized, and images were captured using a gel documentation system (Alpha Innotech Inc., San Leandro, CA). Expression of all the genes was assessed by generating densitometry data for band intensities in different sets of experiments and was generated by analyzing the gel images on the Image J software, Version 1.33 (Wayne Rasband, NIH, Bethesda, MD) semi-quantitatively. The band intensities were compared with constitutively expressed β-actin. The intensity of mRNAs was standardized against that of the β-actin mRNA from each sample, and the results were expressed as PCR-product/β-actin mRNA ratio.
Table 1. Primer sequences for KIM-1, Caspase-3, TGF-β, TNF-α and β-actin.
Histological evaluation of kidney and liver
At the end of experiment, kidney and liver were isolated and fixed for histopathological evaluation with 4% buffered paraformaldehyde solution and embedded in paraffin. Three to four-micrometer thick paraffin sections were dewaxed and brought to the water through graded ethanol. Sections of kidney and liver were stained with hematoxylin–eosin stain (H & E), then dehydrated through graded ethanol, cleared in xylene and mounted with Distyrene Plasticizer Xylene. H & E stained sections were graded to verify the morphological assessment of tissue damage. For staining of renal fibers, the Yuccafine Masson’s trichrome staining kit (Yucca Diagnostics, India) was used. The sections stained with Masson’s trichrome stain were assessed for the presence of interstitial fibrosis, and hematoxylin and eosin-stained sections were graded for the presence of medullary congestion, tubular cell necrosis and dilation, cytoplasmic vacuolization, nuclear pyknosis, and cytoplasmic eosinophilia. Photomicrographs were captured at 40× and 100× magnification.
Statistical analysis
Data was expressed as mean ± standard error mean (SEM). Data analysis was performed using Graph Pad Prism version 5.0 software (Graph Pad, San Diego, CA). Data of biochemical parameters was analyzed by one-way analysis of variance (ANOVA), and Dunnett’s tests were applied for post hoc analysis. A value of p < 0.05 was considered to be statistically significant.
Results
Effect of naringin on sodium arsenite-induced alteration in body weight and relative organs weight in rats
There was significant decreased (p < 0.001) in body weight, relative weights of kidney and liver of arsenic control rats as compared to normal rats. However, there was significant and dose-dependent increase (p < 0.05 and p < 0.01) in body weight, relative weights of kidney and liver in naringin (40 and 80 mg/kg, resp.) treated rats as compared to arsenic control rats. Whereas, treatment with Coenzyme Q10 (10 mg/kg) also showed a significant increase (p < 0.001) in the body weight, the relative weight of kidney and liver as compared to arsenic-treated rats ().
Table 2. Effect of naringin on sodium arsenite-induced alteration in body weight, kidney weight and liver weight.
Effect of naringin on sodium arsenite-induced alteration in renal biomarkers in rats
Oral administration of sodium arsenite resulted in significant increased (p < 0.001) in serum creatinine, BUN, uric acid as compared to normal rats, whereas sodium arsenite treated rats showed a significant decrease (p < 0.001) in urine creatinine and creatinine clearance as compared to normal rats. However, treatment with naringin (40 and 80 mg/kg) showed significantly and dose-dependently decreased (p < 0.01 and p < 0.001, respectively) serum creatinine, BUN, uric acid as well as significant and dose-dependent increased (p < 0.01 and p < 0.001, respectively) in urine creatinine and creatinine clearance as compared to arsenic-treated group. Furthermore, treatment with Coenzyme Q10 (10 mg/kg) showed significant increased (p < 0.001) in serum creatinine, BUN, uric acid as well as significant decreased (p < 0.001) in urine creatinine and creatinine clearance as compared to normal rats ().
Table 3. Effect of naringin on sodium arsenite-induced alteration in kidney and liver function test.
Effect of naringin on sodium arsenite-induced alteration in hepatic biomarkers in rats
The level of serum ALT and AST were increased significantly (p < 0.001) in sodium arsenite control rats as compared to normal rats. Treatment with naringin (40 and 80 mg/kg) showed significant and dose-dependent (p < 0.01 and p < 0.001, respectively) decreased in serum ALT and AST as compared to sodium arsenite control rats, whereas Coenzyme Q10 (10 mg/kg) treated rats also showed significantly decreased (p < 0.001) serum ALT and AST as compared to sodium arsenite control rats ().
Effect of naringin on sodium arsenite-induced alteration in renal oxidative stress biomarkers in rats
There was significantly decreased (p < 0.001) in renal SOD and GSH in sodium arsenite control rats as compared to normal rats. However, treatment with naringin (40 and 80 mg/kg) showed significantly and dose-dependently increased (p < 0.01 and p < 0.001, respectively) renal SOD and GSH level as compared to sodium arsenite control rats. In addition, renal MDA level was significantly increased (p < 0.001) in sodium arsenite treated rats as compared to normal rats, whereas treatment with naringin (40 and 80 mg/kg) showed significantly and dose-dependently decreased (p < 0.01 and p < 0.001, respectively) in renal MDA level as compared with sodium arsenite treated rats. Furthermore, Coenzyme Q10 (10 mg/kg) treated rats show significant increased (p < 0.001) in renal SOD and GSH as well as significant decreased (p < 0.001) in renal MDA contents as compared with sodium arsenite control rats ().
Table 4. Effect of naringin on sodium arsenite-induced alteration in oxido-nitrosative stress and membrane-bound enzyme.
Effect of naringin on sodium arsenite-induced alteration in hepatic oxidative stress biomarkers in rats
The level of hepatic SOD and GSH were significantly decreased (p < 0.001) in sodium arsenite control rats as compared to normal rats, whereas treatment with naringin (40 and 80 mg/kg) show significant and dose-dependent increased (p < 0.01 and p < 0.001, respectively) in hepatic SOD and GSH level as compared to sodium arsenite treated rats. However, hepatic MDA level was significantly decreased (p < 0.001) in sodium arsenite treated rats as compared to normal rats. Treatment with Coenzyme Q10 (10 mg/kg) showed significant increased (p < 0.001) in hepatic SOD and GSH as well as significantly decreased (p < 0.001) the hepatic MDA level as compared with sodium arsenite treated rats ().
Affect of naringin on sodium arsenite-induced alteration in renal and hepatic NO levels in rats
There was significant increased (p < 0.001) in renal and hepatic NO level in sodium arsenite control rats as compared to normal rats. However, treatment with naringin (40 and 80 mg/kg) showed significant and dose-dependent decreased (p < 0.01 and p < 0.001, respectively) in renal and hepatic NO level as compared to sodium arsenite control rats. Furthermore, Coenzyme Q10 (10 mg/kg) treated rats showed significant decreased (p < 0.001) in renal and hepatic NO level as compared with sodium arsenite control rats ().
Effect of naringin on sodium arsenite-induced alteration in renal Na–K-ATPase levels in rats
Membrane bound Na–K-ATPase level was significantly decreased (p < 0.001) in sodium arsenite control rats as compared to normal rats. However, naringin (40 and 80 mg/kg) treated rats showed noteworthy and dose-dependent increase (p < 0.05 and p < 0.01, respectively) in Na–K-ATPase levels as compared to sodium arsenite treated rats, whereas treatment with Coenzyme Q10 (10 mg/kg) showed significant increased (p < 0.001) in Na–K-ATPase levels as compared to sodium arsenite treated rats ().
Effect of naringin on sodium arsenite-induced alteration in renal mRNA expression of KIM-1, Caspase-3, TGF-β, and TNF-α in rats
There was significant up-regulation (p < 0.001) in renal KIM-1 mRNA expression in sodium arsenite control rats as compared to normal rats. Treatment with naringin (40 and 80 mg/kg) significant and dose-dependently down-regulated (p < 0.05 and p < 0.01, respectively) renal KIM-1 mRNA expression as compared to sodium arsenite control rats. When compared with sodium arsenite control rats, the up-regulated mRNA expression of Caspase-3, TGF-β, and TNF-α was significantly and dose-dependently down-regulated (p < 0.01 and p < 0.001, respectively) by naringin (40 and 80 mg/kg) treatment, whereas treatment with Coenzyme Q10 (10 mg/kg) show significant down-regulated (p < 0.001) renal KIM-1, Caspase-3, TGF-β, and TNF-α mRNA expression as compared to sodium arsenite control rats ().
Figure 1. Effect of naringin on sodium arsenite-induced alteration in renal mRNA expression of KIM-1, Caspase-3, TGF-β and TNF-α in rats (A), quantitative representation of mRNA expression of KIM-1 (B), Caspase-3 (C), TGF-β (D) and TNF-α (E). Results are represented as mean ± SEM, (n = 4) Data was analyzed by One-way ANOVA followed by post hoc Dunnett’s tests. *p < 0.05, **p < 0.01 and ***p < 0.001 as compared with the Arsenic Control group. ###p < 0.001 as compared to normal group. N: Normal group; As: Arsenic Control group; CoQ10 (10): Coenzyme Q10 (10 mg/kg, p.o.) treated group; N (20): naringin (20 mg/kg, p.o.) treated group; N (40): naringin (40 mg/kg, p.o.) treated group and N (80): naringin (80 mg/kg, p.o.) treated group.
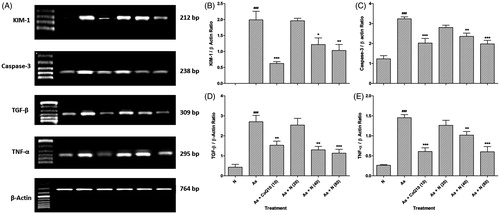
Effect of naringin on sodium arsenite-induced pathological alteration in rat kidney
depicted the normal architecture of kidney tissue from normal rats with devoid of any changes like congestion, necrosis, and inflammatory infiltration with the presence of intact glomerulus basement membrane and tubules. Sodium arsenite caused massive renal damage reflected by the presence of intrinsic lesions within the glomeruli and epithelium. It also showed the presence of edema and inflammatory infiltration (). The thickness of glomerulus basement membrane was increased. Naringin (20 mg/kg) treatment showed the presence of moderate edema and inflammatory infiltration (). However, the thickness of glomerulus basement membrane was remains increased as that of sodium arsenite control rats. Naringin (40 and 80 mg/kg) treatment showed the reduction in the renal damage reflected by decreased inflammatory infiltration with the restoration of the glomerulus basement membrane (, respectively). Renal tissue from Coenzyme Q10 (10 mg/kg) treated rats showed mild number of inflammatory cells with reduced necrosis, edema, and thickens of glomerulus basement membrane was intact ().
Figure 2. Effect of naringin on sodium arsenite-induced alteration in kidney histology of rats. Photomicrograph of sections of kidney from normal group (A); Arsenic Control group (B); Coenzyme Q10 (10 mg/kg, p.o.) treated group (C); Naringin (20 mg/kg, p.o.) treated group (D); Naringin (40 mg/kg, p.o.) treated group (E) and Naringin (80 mg/kg, p.o.) treated group (F). H & E staining at 100× and respective inset at 400×.
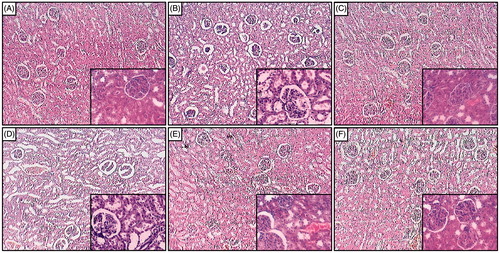
Light microscopic evaluation of the renal tissue from the sodium arsenite control rats after Masson’s trichrome staining showed severe interstitial fibrosis (). Renal tissue from normal rats did not show the presence of any interstitial fibrosis (). Treatment with naringin (20 mg/kg) showed the presence of moderate interstitial fibrosis () whereas naringin (40 and 80 mg/kg) and Coenzyme Q10 (10 mg/kg) treatment decreased interstitial fibrosis (, respectively).
Figure 3. Effect of naringin on sodium arsenite-induced alteration in kidney histology of rats. Photomicrograph of sections of kidney from normal group (A); Arsenic Control group (B); Coenzyme Q10 (10 mg/kg, p.o.) treated group (C); Naringin (20 mg/kg, p.o.) treated group (D); Naringin (40 mg/kg, p.o.) treated group (E) and Naringin (80 mg/kg, p.o.) treated group (F). MT staining at 100×.
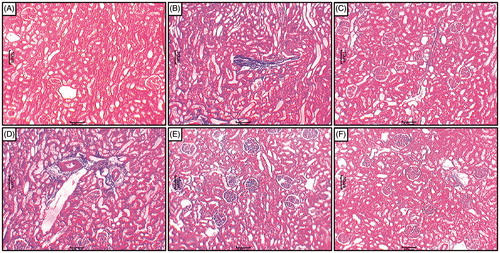
Effect of naringin on sodium arsenite-induced pathological alteration in rat liver
Liver tissue from normal rats showed the normal architecture of the hepatic tissue. The central vein in liver parenchymal cells was normal without any signs of inflammation, necrosis, congestion, and vacuolization (). Administration of sodium arsenite caused inflammatory infiltration, centrilobular necrosis, shrunken hepatocytes, chromatin condensation, cytoplasmic vacuolation, and vascular congestion in the hepatocytes of sodium arsenite control rats (). However, administration of Coenzyme Q10 (10 mg/kg) showed moderate histopathological changes in liver reflected by sinusoidal congestion, cytoplasmic vacuolation, and the presence of only a few inflammatory cells (). In naringin (40 and 80 mg/kg) treated rats, the histology of liver showed mild degree of vacuolization, moderate inflammatory cells, necrosis, and congestion around central vein (, respectively). However, moderate inflammatory infiltration, centrilobular necrosis, cytoplasmic vacuolation, and vascular congestion were present in the histological structure of hepatocytes from naringin (20 mg/kg) treated rats ().
Figure 4. Effect of naringin on sodium arsenite-induced alteration in liver histology of rats. Photomicrograph of sections of liver from normal group (A); Arsenic Control group (B); Coenzyme Q10 (10 mg/kg, p.o.) treated group (C); Naringin (20 mg/kg, p.o.) treated group (D); Naringin (40 mg/kg, p.o.) treated group (E) and Naringin (80 mg/kg, p.o.) treated group (F). H & E staining at 100×.
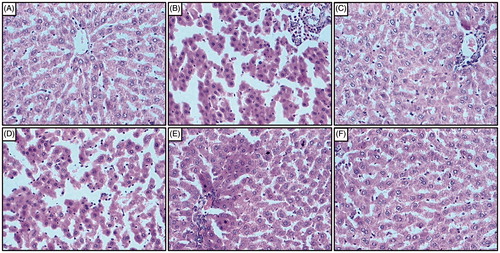
Discussion
Arsenic is ubiquitously present in the environment considered as a one of the heavy metal and human carcinogen.Citation7 Arsenic has wide spectrum of application in the era of agriculture wood preservatives, herbicides, insecticides, fungicides, algaecides as well in the manufacturing of semiconductors, dyestuffs, glass, cigarette, smelters, and as metal alloys additive.Citation48 The mechanism behind the arsenic toxicity is resembled its chemical forms and oxidative state. Arsenic responsible for the generation of reactive oxygen species (ROS) (intracellular peroxide, hydrogen peroxide, superoxide anion radicals, and hydroxyl free radicals), reactive nitrogen species (RNS), which provoke proinflammatory cytokines, profibrogenic cytokines, and assault mitochondrial respiratory chain which create obstacle in the antioxidant defence system further lead cellular DNA and protein marred.Citation49,Citation50 Arsenic after ingestion promptly absorbed by the gastrointestinal tract and circulated in the blood to the kidney, liver, and spleen in other word almost affect all organs.Citation51
Chronic arsenic exposure causes various disease and abnormalities in the several organs like kidney and liver.Citation5 Abatement in body weight is an important signal for the impairment of the animal health status. Nandi et al. (2005) and Kaltreider et al. (2001) revealed that arsenic diminish the calibre of glucocorticoids and its receptor.Citation52,Citation53 Glucocorticoid hormones involve in glucose regulation, lipid, carbohydrates, and protein metabolism. Inhibitory effect of sodium arsenite on the body weight and relative weight of kidney and liver resembles with the finding of previous researchers.Citation54,Citation55 Rahman et al. (1998) explain that long time arsenic exposures upshot inception of diabetes mellitus that elicit the cause of weight loss of rats.Citation56 Indeed, arsenic-induced nephrotoxicity in experimental animals is well-known events.Citation57,Citation58 Arsenic exposure cause renal ruination which impinge tubular and glomerular deterioration with a simultaneous decrease in lysosomal latency as an early beacon of renal mishap.Citation59 It has been revealed that arsenic is an important cause of chronic renal disease.Citation60 Alteration in kidney function due to arsenic toxicity examined by measuring serum and urinary markers of the kidney. Creatine is picked up by skeletal muscles and converted to creatine phosphate by creatine kinase. Both creatine and creatine phosphate are converted non-enzymatically and irreversibly to creatinine. Urinary and serum creatinine are used to evaluate the creatinine clearance that emulate the glomerular filtration rate that is a salient feature of renal function.Citation61 Arsenic damage the cells release creatine phosphokinase that is responsible for the conversion of phosphocreatine into creatine.Citation62 The liver produces urea in the urea cycle as a waste product of the digestion of protein. Blood urea nitrogen (BUN) is a waste product of protein catabolism, terminated by the kidneys as urea, and an indication of renal health. Uric acid is the final product of purine metabolism, and it is formed from guanine and hypoxanthine via xanthine in reactions catalyzed by guanase and xanthine oxidase. The toxic effect of arsenic alters the guanase and xanthine oxidase that elevate the serum uric acid level.Citation62 Previous finding expose that arsenic-treated rat show significant decreased in urinary creatinine and creatinine clearance and increased in the serum BUN and uric acid which in line with our investigation.Citation63
Glutathione (GSH) is a tripeptide non-enzymatic antioxidant that has a beneficiary role in the cell protection against arsenic-induced reactive oxygen species.Citation64 However, various mechanism have been postulated for the impairment of the GSH level in arsenic-induced toxicity; for conversion of As (v) to As (iii) arsenic act as an electron donor,Citation65 sulfhydryl group oxidation impeder.Citation66 In our study, present finding show that GSH level declined in the kidney and liver of arsenic-treated group compared to normal rats indicating oxidative stress resemble with the agreement of previous finding.Citation67,Citation68 However, treatment with naringin noticeably elevate the GSH level in kidney and liver that is in line with the previous investigation.Citation69
Oxidative stress is an important feature of cell apoptosis and necrosis.Citation70–73 It is well known that reactive oxygen species is generated during the process of cell death and an enhanced level of ROS occur due to the production of antioxidant and detoxifying enzymes catalase, or superoxide dismutase has a tutelary role.Citation74–77 SOD abets the dismutation of superoxide to H2O2, which is cleaved by catalase.Citation78–80 It has been revealed that the reaction of molecular oxygen with dimethylarsine (a metabolite of dimethyl arsenic acid) produce free radical species,Citation81–83 Significantly decrease in the activity of SOD content may be due to the overproduction of superoxide during arsenic metabolism.Citation84 In this investigation, arsenic treated group clearly reflects significant reduction of SOD content in kidney and liver due to oxidative stress which is in accordance with the previous study,Citation36,Citation84,Citation85 whereas treatment with naringin remarkably soar the SOD level in aforementioned organs.Citation86
Arsenic activate the release of iron from ferritin through the activation of heme oxygenase the rate-limiting enzyme in heme degradation.Citation87 Free iron is assumed as a potent enhancer of ROS formation, as exemplified by the reduction of H2O2 with the generation of highly aggressive hydroxyl radical.Citation88–92 Hydroxyl radicals penetrate membrane barriers and enter in the cell nucleus where it react mutagenically with DNA and play a noxious role.Citation93–96 Reactive oxygen species can lead lipid peroxidation and havoc the membrane lipid bilayer arrangement that result in deactivation of membrane-bound receptors and enzyme and promote tissue permeability.Citation91,Citation97–99 Evidence from the previous outcome show that arsenic exposure cause elevation of MDA level which is an end product of lipid peroxidation arises through the ROS generation lead oxidative stress in kidney and liver that is correlation with this investigation.Citation52 This study revealed that treatment with naringin refurbishes the MDA level in kidney and liver that is in agreement with the previous paradigm.Citation100
Nitric oxide (NO) interact with molecular oxygen and water generate stable oxidized product, i.e., nitrite and nitrate.Citation101 NO play a vital role as a transcellular messenger in the various physiological processes. NADPH-dependent enzymes known as NO synthases (NOS) responsible for the production of NO from the terminal guanido nitrogen atom of l-arginine.Citation102 NO retain an unpaired electron. Thus, it acts as a free radical. On arsenic exposure NO promptly react with O2− produce a noxious product, peroxynitrite anion that oxidized sulfhydryl groups and thioethers further cause DNA damage.Citation103 Basically, three mechanism participate in DNA damage via NO i.e. N-nitroso compound formation which cause DNA alkylation, interfering with primary amino group of DNA, which leads deamination and finally formation of ROS like peroxynitrite and hydroxyl radicals.Citation104 NO or ROS or may be both are culprit indulge in arsenic-induced DNA strand cleavage, it is up to cell type examined. Mukherjee et al. (2006) aforementioned that rat treated with arsenic show a significant increase in NO production.Citation105 Based on the evidence of this study, it is hypothesized that increase production of NO in kidney and liver due to generation of ROS, i.e., peroxynitrite which result oxidative stress concord with the finding of previous arsenic-induced toxicity model.Citation85 However, treatment with naringin reflects down-regulation of NO level in kidney and liver.
Estimation of membrane-associated enzymes activities such as ATPases are an important hallmark of alteration in membranes under pathological states.Citation106 Membrane bound ATPases comprises sulfhydryl group associated with enzymes and lipid dependent.Citation107 It is previously claimed that sodium–potassium adenosine triphosphatase (Na/K ATPase) responsible for the energy-dependent eviction of sodium ions (Na+) and uptake of potassium ions (K+) play a crucial role in the maintenance of ionic homeostasis.Citation108 Arsenic exposure causes oxidative stress that create hurdles in the antioxidant defence system wreck membrane lipids and debilitate Na/K ATPase functioning that could be important in water/ion transport alteration. Evidence from the previous study revealed that cellular transport incapacitate due to enervated ATP metabolism after arsenic or 4-hydroxy-2-nonenal (a lipid peroxidation product) exposure.Citation109 It is clearly depicted from the various experiment that arsenic-treated rat show downregulation of Na/K ATPase activities in kidney due to increased in lipid peroxidation by free radicals which is consort with the our investigation.Citation85
Heavy metals like arsenic, cadmium, lead, and mercury disturb the antioxidant defence system and depletion of thiol status cause ROS generation which ultimately show the deleterious effect on cellular enzymes.Citation110 Arsenic exposure is an important cause of nephrotoxicity is kindred with oxidative stress, proximal tubular menace and necrosis of proximal tubular epithelial cell in kidney of rats.Citation111 Kidney injury molecule-1 (KIM-1) is a transmembrane glycoprotein which is normally undetectable in normal kidney thoroughly express in dedifferentiated proximal tubule cells after toxic insult or ischemic acute kidney injury in animal models.Citation112,Citation113 It is previously reported that KIM-1 is a promising tissue and urinary biomarker for kidney injury induced by various nephrotoxicants. Tissue and urinary expression of KIM-1 were elucidated by numerous nephrotoxic doses of cadmium, mercury, chromium, cisplatin, gentamicin, and folic acid.Citation114,Citation115 In this study, we evaluate the possible role of KIM-1 in association with arsenic-induced nephrotoxicity has not been reported previously. Expression of KIM-1was increased in the control rats, whereas treatment with naringin reduced these increased level of renal KIM-1 mRNA expression as compared to control rats.
Arsenic intoxication leads generation of ROS, which cause production of apoptosis and modification of cells.Citation116 Arsenic compounds such as Arsenic trioxide (As2O3) combine with adenine nucleotide translocator in the mitochondria or directly act on the mitochondria responsible for opening of mitochondrial permeability transition pore and thus release cytochrome c (Cyt c),Citation117 decline mitochondrial membrane potential, activation of caspases, i.e., caspase-3 and caspase-9.Citation118,Citation119 Caspase-3 is an important apoptotic marker imparts in cytoskeletal deterioration, nuclear decrease as well as cells alteration in coordination with apoptosis.Citation120 It has been previously reported that arsenic-treated rats show activation of renal casapase-3 expression via enhanced apoptosis and cytoskeletal disintegrity which is concurrent with the finding of our investigation.Citation85,Citation121 Naringin treated group show a remarkably decrement in Caspase-3 level in arsenic administered rats as compared to control rats.
Transforming growth factor-β (TGF-β) is a protein involve in various cellular processes including differentiation, growth, immunosuppression, angiogenesis, cancerization as well as extracellular matrix formation. TGF-β1 is isoforms of TGF-β apprehend the cell cycle in G1 phase that evoke restrain of cell proliferation and promote apoptosis. TGF-β1 is a vigorous profibrogenic cytokine and increased in TGF-β1 levels due to oxidative stress cause activation of profibrotic signaling pathways.Citation122 Previously it is reported that arsenic lead ROS generation which aggravates pro-inflammatory and profibrogenic cytokines in renal and hepatic tissue.Citation50 In normal tissue, non-parenchymal cells (fat-storing cell, kupffers cell, and endothelial cell) source of TGF-β1 production.Citation123 From the evidence of previous study, it is cleared that sodium arsenite treated group show significant upregulation of renal TGF-β which is in line with our investigation.Citation124 This study shows noteworthy down-regulation of renal TGF-β on treatment with naringin.
Tumor necrosis factor-alpha (TNF-α) is an important pro-inflammatory chemokines and cytokines that play a key role in adaptive and innate immunity. Due to its pro-inflammatory characteristic it involves in recruitment and activation of inflammatory cells at the injury sites.Citation125 It is well described that ROS is produced by cytokines and elevated level of ROS by the metabolism of arsenic may be responsible for the activation of TNF-α synthesis.Citation126 It has been already documented that ROS generation is the consequences of arsenic exposure that leads to tissue fibrosis.Citation127 TNF-α play a foremost role in the actuation of HSCs (hepatic stellate cells), predominant cells impart in the creation of collagen type-I in the liver and involve in remodelling of the extracellular matrix and histological hepatic fibrosis.Citation128 In kidney, glomerular mesangial cells are the prime genesis of TNF-α.Citation129 TNF-α abate glomerular blood flow and GFR by activating mesangial cells to beget vasodilatory and vasoconstrictive mediators.Citation130 Ramanathan et al. (2005) previously claimed that on arsenic administration there was a conspicuous upswing in the extent of renal TNF-α which is in accordance with findings of this investigation.Citation131 However, arsenic-exposed rat treated with naringin show a significant reduction in renal TNF-α level.
In conclusion, the results of this investigation shows that naringin treatment ameliorates arsenic-induced renal and hepatic damage in rats, which could be due its antioxidant and anti-inflammatory properties via down-regulation of elevated oxido-nitrosative stress, KIM-1, Caspase-3, TGF-β, and TNF-α levels.
Acknowledgments
The authors acknowledge Dr. S. S. Kadam, Vice Chancellor, Bharati Vidyapeeth Deemed University and Dr. K. R. Mahadik, Principal, Poona College of Pharmacy for their keen interest and providing the necessary facilities to carry out the study.
Declaration of interest
We are also thankful to the All India Council of Technical and Education (AICTE), India for financial support by awarding GPAT Scholarship to one of the authors Mr. Mohammad Adil for the research work.
The authors report no conflicts of interest. The authors alone are responsible for the content and writing of this article.
References
- Singh KP, DuMond JW. Genetic and epigenetic changes induced by chronic low dose exposure to arsenic of mouse testicular Leydig cells. Int J Oncol. 2007;30:253–260
- Mazumder DG. Effect of chronic intake of arsenic-contaminated water on liver. Toxicol Appl Pharmacol. 2005;206:169–175
- Humans IWGotEoCRt, Cancer IAfRo. IARC Monographs on the Evaluation of Carcinogenic Risks to Humans. Geneva: World Health Organization; 2004
- Szymanska-Chabowska A, Antonowicz-Juchniewicz J, Andrzejak R. Some aspects of arsenic toxicity and carcinogenicity in living organism with special regard to its influence on cardiovascular system, blood and bone marrow. Int J Occup Med Environ Health. 2002;15:101–116
- Chowdhury UK, Rahman MM, Mandal B, et al. Groundwater arsenic contamination and human suffering in West Bengal, India and Bangladesh. Environ Sci. 2001;8:393–415
- Liu SX, Athar M, Lippai I, Waldren C, Hei TK. Induction of oxyradicals by arsenic: Implication for mechanism of genotoxicity. Proc Natl Acad Sci USA. 2001;98:1643–1648
- Lin A, Zhang X, Zhu YG, Zhao FJ. Arsenate-induced toxicity: Effects on antioxidative enzymes and DNA damage in Vicia faba. Environ Toxicol Chem. 2008;27:413–419
- Nandi D, Patra R, Swarup D. Oxidative stress indices and plasma biochemical parameters during oral exposure to arsenic in rats. Food Chem Toxicol. 2006;44:1579–1584
- Honmore V, Kandhare A, Zanwar AA, Rojatkar S, Bodhankar S, Natu A. Artemisia pallens alleviates acetaminophen induced toxicity via modulation of endogenous biomarkers. Pharm Biol. 2015;53:571–581
- Kandhare A, Patil MV, Bodhankar S. L-arginine attenuates the ethylene glycol induced urolithiasis in uninephrectomized hypertensive rats: Role of KIM-1, NGAL and NOs. Ren Fail. 2015;37:709–721
- Visnagri A, Kandhare AD, Bodhankar SL. Renoprotective effect of berberine via intonation on apoptosis and mitochondrial-dependent pathway in renal ischemia reperfusion-induced mutilation. Ren Fail. 2015;37:482–493
- Tsai S-M, Wang T-N, Ko Y-C. Mortality for certain diseases in areas with high levels of arsenic in drinking water. Arch Environ Health Int J 1999;54:186–193
- Hughes MF, Kenyon EM, Edwards BC, Mitchell CT, Del Razo LM, Thomas DJ. Accumulation and metabolism of arsenic in mice after repeated oral administration of arsenate. Toxicol Appl Pharmacol. 2003;191:202–210
- Islam K, Haque A, Karim R, et al. Dose-response relationship between arsenic exposure and the serum enzymes for liver function tests in the individuals exposed to arsenic: A cross sectional study in Bangladesh. Environ Health, 2011;10:64
- Fowler BA, Woods JS, Schiller CM. Ultrastructural and biochemical effects of prolonged oral arsenic exposure on liver mitochondria of rats. Environ Health Perspect. 1977;19:197
- Hubaux R, Becker-Santos DD, Enfield K, et al. Molecular features in arsenic-induced lung tumors. Mol Cancer. 2013;12:20
- Manna P, Sinha M, Sil PC. Arsenic-induced oxidative myocardial injury: Protective role of arjunolic acid. Arch Toxicol. 2008;82:137–149
- Singh N, Kumar D, Lal K, Raisuddin S, Sahu AP. Adverse health effects due to arsenic exposure: Modification by dietary supplementation of jaggery in mice. Toxicol Appl Pharmacol. 2010;242:247–255
- Tripoli E, La Guardia M, Giammanco S, Di Majo D, Giammanco M. Citrus flavonoids: Molecular structure, biological activity and nutritional properties: A review. Food Chem. 2007;104:466–479
- Kim D-H, Jung E-A, Sohng I-S, Han J-A, Kim T-H, Han MJ. Intestinal bacterial metabolism of flavonoids and its relation to some biological activities. Arch Pharm Res. 1998;21:17–23
- Chen C, Chen C, Wu M, Kuo T. Cancer potential in liver, lung, bladder and kidney due to ingested inorganic arsenic in drinking water. Br J Cancer. 1992;66:888
- Gopinath K, Prakash D, Sudhandiran G. Neuroprotective effect of naringin, a dietary flavonoid against 3-nitropropionic acid-induced neuronal apoptosis. Neurochem Int. 2011;59:1066–1073
- Zbarsky V, Datla KP, Parkar S, Rai DK, Aruoma OI, Dexter DT. Neuroprotective properties of the natural phenolic antioxidants curcumin and naringenin but not quercetin and fisetin in a 6-OHDA model of Parkinson's disease. Free Radic Res. 2005;39:1119–1125
- Jeon S-M, Park YB, Choi M-S. Antihypercholesterolemic property of naringin alters plasma and tissue lipids, cholesterol-regulating enzymes, fecal sterol and tissue morphology in rabbits. Clin Nutr. 2004;23:1025–1034
- So FV, Guthrie N, Chambers AF, Moussa M, Carroll KK. Inhibition of human breast cancer cell proliferation and delay of mammary tumorigenesis by flavonoids and citrus juices. Nutr Cancer. 1996;26:167–81
- Kandhare AD, Alam J, Patil MVK, Sinha A, Bodhankar SL. Wound healing potential of naringin ointment formulation via regulating the expression of inflammatory, apoptotic and growth mediators in experimental rats. Pharm Biol. 2015;1–14. doi:10.3109/13880209.2015.1038755
- Kandhare AD, Ghosh P, Bodhankar SL. Naringin, a flavanone glycoside, promotes angiogenesis and inhibits endothelial apoptosis through modulation of inflammatory and growth factor expression in diabetic foot ulcer in rats. Chem Biol Interact. 2014;219C:101–112
- Kandhare AD, Raygude KS, Ghosh P, Ghule AE, Bodhankar SL. Neuroprotective effect of naringin by modulation of endogenous biomarkers in streptozotocin induced painful diabetic neuropathy. Fitoterapia. 2012;83:650–659
- Kumar VS, Rajmane AR, Adil M, Kandhare AD, Ghosh P, Bodhankar SL. Naringin ameliorates acetic acid induced colitis through modulation of endogenous oxido-nitrosative balance and DNA damage in rats. J Biomed Res. 2014;28:132–145
- Lee M-H, Yoon S, Moon J-O. The flavonoid naringenin inhibits dimethylnitrosamine-induced liver damage in rats. Biol Pharm Bull. 2004;27:72–6
- Badary OA, Abdel-Maksoud S, Ahmed WA, Owieda GH. Naringenin attenuates cisplatin nephrotoxicity in rats. Life Sci. 2005;76:2125–2135
- Butterfield DA, Howard B, Yatin S, et al. Elevated oxidative stress in models of normal brain aging and Alzheimer's disease. Life Sci. 1999;65:1883–1892
- Gaur V, Aggarwal A, Kumar A. Protective effect of naringin against ischemic reperfusion cerebral injury: Possible neurobehavioral, biochemical and cellular alterations in rat brain. Eur J Pharmacol. 2009;616:147–154
- Golechha M, Chaudhry U, Bhatia J, Saluja D, Arya DS. Naringin protects against kainic acid-induced status epilepticus in rats: Evidence for an antioxidant, anti-inflammatory and neuroprotective intervention. Biol Pharm Bull. 2011;34:360–365
- Rajadurai M, Prince PSM. Preventive effect of naringin on isoproterenol-induced cardiotoxicity in Wistar rats: An in vivo and in vitro study. Toxicology. 2007;232:216–225
- Adil M, Visnagri A, Kumar VS, Kandhare AD, Ghosh P. Protective effect of naringin on sodium arsenite induced testicular toxicity via modulation of biochemical perturbations in experimental rats. Pharmacologia. 2014;5:222–234
- El-Demerdash FM, Yousef MI, Radwan FM. Ameliorating effect of curcumin on sodium arsenite-induced oxidative damage and lipid peroxidation in different rat organs. Food Chem Toxicol. 2009;47:249–254
- Visnagri A, Kandhare AD, Shiva Kumar V, et al. Elucidation of ameliorative effect of Co-enzyme Q10 in streptozotocin-induced diabetic neuropathic perturbation by modulation of electrophysiological, biochemical and behavioral markers. Biomed Aging Pathol. 2012;2:157–172
- Kandhare AD, Ghosh P, Ghule AE, Bodhankar SL. Elucidation of molecular mechanism involved in neuroprotective effect of Coenzyme Q10 in alcohol induced neuropathic pain. Fundam Clin Pharmacol. 2013;27:603–622
- Badole SL, Chaudhari SM, Jangam GB, Kandhare AD, Bodhankar SL. Cardioprotective activity of Pongamia pinnata in streptozotocin-nicotinamide induced diabetic rats. BioMed Res Int. 2015;2015:403291
- Gosavi TP, Ghosh P, Kandhare AD, et al. Therapeutic effect of H. pylori nosode, a homeopathic preparation in healing of chronic H. pylori infected ulcers in laboratory animals. Asian Pacif J Trop Dis. 2012;2:S603–S611
- Gosavi TP, Kandhare AD, Ghosh P, Bodhankar SL. Anticonvulsant activity of Argentum metallicum, a homeopathic preparation. Pharm Lett. 2012;4:626–637
- Goswami S, Kandhare A, Zanwar AA, et al. Oral l-glutamine administration attenuated cutaneous wound healing in Wistar rats. Int Wound J 2014. doi:10.1111/iwj.12246
- Kandhare AD, Shivakumar V, Rajmane A, Ghosh P, Bodhankar SL. Evaluation of the neuroprotective effect of chrysin via modulation of endogenous biomarkers in a rat model of spinal cord injury. J Nat Med. 2014;68:586–603
- Visnagri A, Kandhare AD, Chakravarty S, Ghosh P, Bodhankar SL. Hesperidin, a flavanoglycone attenuates experimental diabetic neuropathy via modulation of cellular and biochemical marker to improve nerve functions. Pharm Biol. 2014;52:814–828
- Kandhare AD, Bodhankar SL, Mohan V, Thakurdesai PA. Prophylactic efficacy and possible mechanisms of oligosaccharides based standardized fenugreek seed extract on high-fat diet-induced insulin resistance in C57BL/6 mice. J Appl Pharm Sci. 2015;5:035–045
- Ketkar S, Rathore A, Kandhare A, et al. Alleviating exercise induced muscular stress using neat and processed bee pollen: Oxidative markers, mitochondrial enzymes and myostatin expression in rats. Integr Med Res 2015. doi:10.1016/j.imr.2015.02.003
- Bhadauria S, Flora S. Response of arsenic-induced oxidative stress, DNA damage, and metal imbalance to combined administration of DMSA and monoisoamyl-DMSA during chronic arsenic poisoning in rats. Cell Biol Toxicol. 2007;23:91–104
- Kitchin KT, Ahmad S. Oxidative stress as a possible mode of action for arsenic carcinogenesis. Toxicol Lett. 2003;137:3–13
- Brunati AM, Pagano MA, Bindoli A, Rigobello MP. Thiol redox systems and protein kinases in hepatic stellate cell regulatory processes. Free Radic Res. 2010;44:363–378
- Yousef MI, El-Demerdash FM, Radwan FM. Sodium arsenite induced biochemical perturbations in rats: Ameliorating effect of curcumin. Food Chem Toxicol. 2008;46:3506–3511
- Nandi D, Patra R, Swarup D. Effect of cysteine, methionine, ascorbic acid and thiamine on arsenic-induced oxidative stress and biochemical alterations in rats. Toxicology. 2005;211:26–35
- Kaltreider RC, Davis AM, Lariviere JP, Hamilton JW. Arsenic alters the function of the glucocorticoid receptor as a transcription factor. Environ Health Perspect 2001;109:245
- Bashir S, Sharma Y, Irshad M, Gupta SD, Dogra T. Arsenic-induced cell death in liver and brain of experimental rats. Basic Clin Pharm Toxicol. 2006;98:38–43
- Jana K, Jana S, Samanta PK. Effects of chronic exposure to sodium arsenite on hypothalamo-pituitary-testicular activities in adult rats: Possible an estrogenic mode of action. Reprod Biol Endocrinol. 2006;4:1–13
- Rahman M, Tondel M, Ahmad SA, Axelson O. Diabetes mellitus associated with arsenic exposure in Bangladesh. Am J Epidemiol. 1998;148:198–203
- Kimura A, Ishida Y, Hayashi T, et al. Interferon-γ plays protective roles in sodium arsenite-induced renal injury by up-regulating intrarenal multidrug resistance-associated protein 1 expression. Am J Pathol. 2006;169:1118–1128
- Ayala-Fierro F, Baldwin AL, Wilson LM, Valeski JE, Carter DE. Structural alterations in the rat kidney after acute arsine exposure. Lab Invest. 2000;80:87–97
- Dalal P, Gangopadhyay A, Roychowdhury A. Identification of indicators of arsenic induced nephrotoxicity in humans. Internet J Toxicol 2008;5
- Madden EF, Fowler BA. Mechanisms of nephrotoxicity from metal combinations: A review. Drug Chem Toxicol. 2000;23:1–12
- Gross JL, De Azevedo MJ, Silveiro SP, Canani LH, Caramori ML, Zelmanovitz T. Diabetic nephropathy: Diagnosis, prevention, and treatment. Diabetes Care. 2005;28:164–176
- Saxena PN, Anand S, Saxena N, Bajaj P. Effect of arsenic trioxide on renal functions and its modulation by Curcuma aromatica leaf extract in albino rat. J Environ Biol. 2009;30:527–531
- Zhang J, Pan X, Li N, et al. Grape seed extract attenuates arsenic-induced nephrotoxicity in rats. Exp Ther Med 2014;7:260–266
- Masella R, Di Benedetto R, Varì R, Filesi C, Giovannini C. Novel mechanisms of natural antioxidant compounds in biological systems: Involvement of glutathione and glutathione-related enzymes. J Nutr Biochem. 2005;16:577–586
- Zakharyan RA, Aposhian HV. Enzymatic reduction of arsenic compounds in mammalian systems: The rate-limiting enzyme of rabbit liver arsenic biotransformation is MMAV reductase. Chem Res Toxicol. 1999;12:1278–1283
- Afzal M, Afzal A, Jones A, Armstrong D. A rapid method for the quantification of GSH and GSSG in biological samples. Methods Mol Biol. 2002;186:117–122
- Muthumani M. Tetrahydrocurcumin potentially attenuates arsenic induced oxidative hepatic dysfunction in rats. J Clin Toxicol. 2013;3:1000168
- Hemalatha P, Reddy AG, Reddy YR, Shivakumar P. A study on arsenic-induced toxicity in rats. J Chem Pharm Res 2012;4:4459--4461
- Singh D, Chopra K. The effect of naringin, a bioflavonoid on ischemia-reperfusion induced renal injury in rats. Pharmacol Res. 2004;50:187–193
- Fiers W, Beyaert R, Declercq W, Vandenabeele P. More than one way to die: Apoptosis, necrosis and reactive oxygen damage. Oncogene. 1999;18:7719–7730
- Aswar UM, Kandhare AD, Mohan V, Thakurdesai PA. Anti-allergic effect of intranasal administration of type-A procyanidin polyphenols based standardized extract of cinnamon bark in ovalbumin sensitized BALB/c mice. Phytother Res. 2014;29:423--433
- Kamble H, Kandhare AD, Bodhankar S, Mohan V, Thakurdesai P. Effect of low molecular weight galactomannans from fenugreek seeds on animal models of diabetes mellitus. Biomed Aging Pathol. 2013;3:145–151
- Kandhare AD, Bodhankar SL, Mohan V, Thakurdesai PA. Acute and repeated doses (28 days) oral toxicity study of glycosides based standardized fenugreek seed extract in laboratory mice. Regul Toxicol Pharmacol. 2015;72:323–334
- Kandhare A, Raygude K, Ghosh P, Bodhankar S. The ameliorative effect of fisetin, a bioflavonoid, on ethanol-induced and pylorus ligation-induced gastric ulcer in rats. Int J Green Pharm. 2011;5:236–243
- Kandhare AD, Bodhankar SL, Singh V, Mohan V, Thakurdesai PA. Anti-asthmatic effects of type-A procyanidine polyphenols from cinnamon bark in ovalbumin-induced airway hyperresponsiveness in laboratory animals. Biomed Aging Pathol. 2013;3:23–30
- Sarkar A, Sengupta A, Mukhrjee A, et al. Antiulcer potential of morin in acetic acid-induced gastric ulcer via modulation of endogenous biomarkers in laboratory animals. Pharmacologia. 2015;6:273–281
- Visnagri A, Kandhare AD, Ghosh P, Bodhankar SL. Endothelin receptor blocker bosentan inhibits hypertensive cardiac fibrosis in pressure overload-induced cardiac hypertrophy in rats. Cardiovasc Endocrinol. 2013;2:85–97
- Usoh I, Akpan E, Etim E, Farombi E. Antioxidant actions of dried flower extracts of Hibiscus sabdariffa L. on sodium arsenite-induced oxidative stress in rats. Pak J Nutr. 2005;4:135–141
- Raygude KS, Kandhare AD, Ghosh P, Ghule AE, Bodhankar SL. Evaluation of ameliorative effect of quercetin in experimental model of alcoholic neuropathy in rats. Inflammopharmacology. 2012;20:331–341
- Saraswathi KY, Muthal A, Kandhare A, Rojatkar S, Bodhankar S. Study of methanolic extract of Artemisia pallens wall on endurance of laboratory animals. Pharmacologia. 2014;5:298–309
- Yamanaka K, Okada S. Induction of lung-specific DNA damage by metabolically methylated arsenics via the production of free radicals. Environ Health Perspect. 1994;102:37
- Kandhare AD, Ghosh P, Ghule AE, Zambare GN, Bodhankar SL. Protective effect of Phyllanthus amarus by modulation of endogenous biomarkers and DNA damage in acetic acid induced ulcerative colitis: Role of phyllanthin and hypophyllanthin. Apollo Med. 2013;10:87–97
- Kandhare AD, Kumar VS, Adil M, Rajmane AR, Ghosh P, Bodhankar SL. Investigation of gastro protective activity of Xanthium strumarium L. by modulation of cellular and biochemical marker. Orient Pharm Exp Med. 2012;12:287–299
- Gupta R, Flora S. Effect of Centella asiatica on arsenic induced oxidative stress and metal distribution in rats. J Appl Toxicol. 2006;26:213–222
- Prabu SM, Muthumani M. Silibinin ameliorates arsenic induced nephrotoxicity by abrogation of oxidative stress, inflammation and apoptosis in rats. Mol Biol Rep. 2012;39:11201–11216
- Chen Y, Nie Y-c, Luo Y-l, et al. Protective effects of naringin against paraquat-induced acute lung injury and pulmonary fibrosis in mice. Food Chem Toxicol. 2013;58:133–140
- Ahmad S, Kitchin KT, Cullen WR. Arsenic species that cause release of iron from ferritin and generation of activated oxygen. Arch Biochem Biophys. 2000;382:195–202
- Farber JL, Kyle ME, Coleman JB. Mechanisms of cell injury by activated oxygen species. Lab Invest. 1990;62:670
- Kandhare AD, Raygude KS, Shiva Kumar V, et al. Ameliorative effects quercetin against impaired motor nerve function, inflammatory mediators and apoptosis in neonatal streptozotocin-induced diabetic neuropathy in rats. Biomed Aging Pathol. 2012;2:173–186
- Patil M, Kandhare A, Bhise S. Pharmacological evaluation of ameliorative effect of aqueous extract of Cucumis sativus L. fruit formulation on wound healing in Wistar rats. Chron Young Sci. 2011;2:207–213
- Patil M, Kandhare A, Bhise S. Anti-inflammatory effect of Daucus carota root on experimental colitis in rats. Int J Pharm Pharm Sci. 2012;4:337–343
- Raygude KS, Kandhare AD, Ghosh P, Bodhankar SL. Anticonvulsant effect of fisetin by modulation of endogenous biomarkers. Biomed Prev Nutr. 2012;2:215–222
- Robertson RP, Harmon J, Tran PO, Tanaka Y, Takahashi H. Glucose toxicity in β-cells: Type 2 diabetes, good radicals gone bad, and the glutathione connection. Diabetes. 2003;52:581–587
- Kandhare AD, Raygude KS, Ghosh P, et al. Effect of hydroalcoholic extract of Hibiscus rosa sinensis Linn. leaves in experimental colitis in rats. Asian Pac J Trop Biomed. 2012;5:337–344
- Patil MVK, Kandhare AD, Bhise SD. Anti-arthritic and anti-inflammatory activity of Xanthium srtumarium L. ethanolic extract in Freund's complete adjuvant induced arthritis. Biomed Aging Pathol 2012;2:6–15
- Patil MVK, Kandhare AD, Bhise SD. Effect of aqueous extract of Cucumis sativus Linn. fruit in ulcerative colitis in laboratory animals. Asian Pac J Trop Biomed. 2012;2:S962–S969
- Girotti AW. Mechanisms of lipid peroxidation. J Free Radic Biol Med. 1985;1:87–95
- Kandhare AD, Raygude KS, Ghosh P, Ghule AE, Bodhankar SL. Therapeutic role of curcumin in prevention of biochemical and behavioral aberration induced by alcoholic neuropathy in laboratory animals. Neurosci Lett. 2012;511:18–22
- Patil MVK, Kandhare AD, Bhise SD. Pharmacological evaluation of ethanolic extract of Daucus carota Linn root formulated cream on wound healing using excision and incision wound model. Asian Pac J Trop Biomed 2012;2:S646–S655
- Mahmoud M, Hamdan D, Wink M, El-Shazly A. Naringin and rutin prevent D-Galactosamine-induced hepatic injury in rats via attenuation of the inflammatory cascade and oxidative stress. Eur Sci J. 2013;9:141--155
- Ignarro LJ, Fukuto JM, Griscavage JM, Rogers NE, Byrns RE. Oxidation of nitric oxide in aqueous solution to nitrite but not nitrate: Comparison with enzymatically formed nitric oxide from L-arginine. Proc Natl Acad Sci USA. 1993;90:8103–8107
- Lala PK, Chakraborty C. Role of nitric oxide in carcinogenesis and tumour progression. Lancet Oncol. 2001;2:149–156
- Beckman JS, Beckman TW, Chen J, Marshall PA, Freeman BA. Apparent hydroxyl radical production by peroxynitrite: Implications for endothelial injury from nitric oxide and superoxide. Proc Natl Acad Sci USA. 1990;87:1620–1624
- Leaf CD, Wishnok JS, Tannenbaum SR. Endogenous incorporation of nitric oxide from L-arginine into N-nitrosomorpholine sitmulated by Escherichia coli lipopolysaccharide in the rat. Carcinogenesis. 1991;12:537–539
- Mukherjee S, Das D, Mukherjee M, Das AS, Mitra C. Synergistic effect of folic acid and vitamin B 12 in ameliorating arsenic-induced oxidative damage in pancreatic tissue of rat. J Nutr Biochem. 2006;17:319–327
- Kempaiah RK, Srinivasan K. Beneficial influence of dietary curcumin, capsaicin and garlic on erythrocyte integrity in high-fat fed rats. J Nutr Biochem. 2006;17:471–478
- Ithayarasi AP, Devi C. Effect of a-tocopherol on lipid peroxidation in isoproterenol induced myocardial infarction in rats. Indian J Physiol Pharmacol. 1997;41:369–376
- Park S, Jung Y. Combined actions of Na+/K+-ATPase, NCX1 and glutamate dependent NMDA receptors in ischemic rat brain penumbra. Anat Cell Biol. 2010;43:201–210
- Miyake H, Kadoya A, Ohyashiki T. Increase in molecular rigidity of the protein conformation of brain Na+-K+-ATPase by modification with 4-hydroxy-2-nonenal. Biol Pharm Bull. 2003;26:1652–1656
- Ercal N, Gurer-Orhan H, Aykin-Burns N. Toxic metals and oxidative stress part I: Mechanisms involved in metal-induced oxidative damage. Curr Top Med Chem. 2001;1:529–539
- Li Y, Ling M, Xu Y, et al. The repressive effect of NF-κB on p53 by Mot-2 is involved in human keratinocyte transformation induced by low levels of arsenite. Toxicol Sci. 2010;116:174–182
- Han WK, Bailly V, Abichandani R, Thadhani R, Bonventre JV. Kidney Injury Molecule-1 (KIM-1): A novel biomarker for human renal proximal tubule injury. Kidney Int. 2002;62:237–244
- Kandhare AD, Raygude KS, Ghosh P, Gosavi TP, Bodhankar SL. Patentability of Animal Models: India and the Globe. Int J Pharm Biol Arch. 2011;2:1024–1032
- Prozialeck W, Vaidya V, Liu J, et al. Kidney injury molecule-1 is an early biomarker of cadmium nephrotoxicity. Kidney Int. 2007;72:985–993
- Vaidya VS, Ramirez V, Ichimura T, Bobadilla NA, Bonventre JV. Urinary kidney injury molecule-1: A sensitive quantitative biomarker for early detection of kidney tubular injury. Am J Physiol Ren Physiol. 2006;290:F517–F529
- Maeda H, Hori S, Nishitoh H, et al. Tumor growth inhibition by arsenic trioxide (As2O3) in the orthotopic metastasis model of androgen-independent prostate cancer. Cancer Res. 2001;61:5432–5440
- Belzacq A-S, El Hamel C, Vieira H, et al. Adenine nucleotide translocator mediates the mitochondrial membrane permeabilization induced by lonidamine, arsenite and CD437. Oncogene. 2001;20:7579–7587
- Banerjee N, Banerjee M, Ganguly S, et al. Arsenic-induced mitochondrial instability leading to programmed cell death in the exposed individuals. Toxicology. 2008;246:101–111
- Li D, Morimoto K, Takeshita T, Lu Y. Formamidopyrimidine-DNA glycosylase enhances arsenic-induced DNA strand breaks in PHA-stimulated and unstimulated human lymphocytes. Environ Health Perspect 2001;109:523
- Bratton SB, Cohen GM. Apoptotic death sensor: An organelle's alter ego? Trends Pharmacol Sci. 2001;22:306–315
- Majumdar S, Karmakar S, Maiti A, et al. Arsenic-induced hepatic mitochondrial toxicity in rats and its amelioration by dietary phosphate. Environ Toxicol Pharmacol. 2011;31:107–118
- Samarakoon R, Overstreet JM, Higgins SP, Higgins PJ. TGF-β1→ SMAD/p53/USF2→ PAI-1 transcriptional axis in ureteral obstruction-induced renal fibrosis. Cell Tissue Res. 2012;347:117–128
- Bissell DM, Roulot D, George J. Transforming growth factor β and the liver. Hepatology. 2001;34:859–867
- Ghosh D, Ghosh S, Sarkar S, et al. Quercetin in vesicular delivery systems: Evaluation in combating arsenic-induced acute liver toxicity associated gene expression in rat model. Chem Biol Interact. 2010;186:61–71
- Ernandez T, Mayadas T. Immunoregulatory role of TNFα in inflammatory kidney diseases. Kidney Int. 2009;76:262–276
- Schreck R, Rieber P, Baeuerle PA. Reactive oxygen intermediates as apparently widely used messengers in the activation of the NF-kappa B transcription factor and HIV-1. EMBO J. 1991;10:2247
- Mazumder DG. Chronic arsenic toxicity & human health. Indian J Med Res. 2008;128:436–447
- Friedman SL. Mechanisms of hepatic fibrogenesis. Gastroenterology. 2008;134:1655–1669
- Fouqueray B, Philippe C, Herbelin A, Perez J, Ardaillou R, Baud L. Cytokine formation within rat glomeruli during experimental endotoxemia. J Am Soc Nephrol. 1993;3:1783–1791
- Donnahoo KK, Shames BD, Harken AH, Meldrum DR. Review article: The role of tumor necrosis factor in renal ischemia-reperfusion injury. J Urol. 1999;162:196–203
- Ramanathan K, Anusuyadevi M, Shila S, Panneerselvam C. Ascorbic acid and α-tocopherol as potent modulators of apoptosis on arsenic induced toxicity in rats. Toxicol Lett. 2005;156:297–306