Abstract
Context: Acetaminophen (APAP) is an analgesic and antipyretic agent commonly known agent to cause hepatic and renal toxicity at a higher dose. Naringin, a bioflavonoid possesses multiple pharmacological properties such as antioxidant, anti-inflammatory, analgesic and anti-hyperlipidemic activity. Objective: To evaluate the effect of naringin against the APAP-induced hepatic and renal toxicity. Materials and methods: Male Wistar albino rats (180–220 g) were divided into various groups, and toxicity was induced by APAP (700 mg/kg, p.o., 14 days). Naringin (20, 40 and 80 mg/kg, p.o.) or Silymarin (25 mg/kg) was administered to rats 2 h before APAP oral administration. Various biochemical, molecular and histopathological parameter were accessed in hepatic and renal tissue. Results: Naringin pretreatment significantly decreased (p < 0.05) serum creatinine, blood urea nitrogen, bilirubin, aspartate transaminase, alanine transaminase, lactate dehydrogenase, low-density lipoprotein, very low-density lipoprotein, cholesterol and triglycerides as compared with APAP control rats. Decreased level of serum albumin, uric acid, and high-density lipoprotein were also significantly restored (p < 0.05) by naringin pretreatment. It also significantly restores (p < 0.05) the altered level of superoxide dismutase, reduced glutathione, malondialdehyde and nitric oxide in hepatic and renal tissue. Moreover, altered mRNA expression of hepatic farnesoid X receptor and renal injury molecule-1 (KIM-1) were significantly restored (p < 0.05) by naringin treatment. Naringin treatment also reduced histological alteration induced by APAP in the liver and kidney. Conclusion: Naringin exerts its hepato- and nephroprotective effect via modulation of oxido-nitrosative stress, FXR and KIM-1 mRNA expression.
Introduction
Acetaminophen (N-acetyl-p-aminophenol also known as APAP and Paracetamol), is a widely prescribed as well as over the counter analgesic and antipyretic drugs either as a single compound or combination with other drugs.Citation1–3 APAP is safe at a therapeutic dose, but an acute overdose or extreme chronic administration can cause serious adverse events including hepatic and renal toxicity.Citation4,Citation5 When ingested at a therapeutic dose, APAP is metabolized by a cytochrome P450 and detoxified by glucuronidation as well as sulfation whereas N-acetyl-p-benzoquinone imine (NAPQI) with conjugated glutathione (GSH).Citation6 On the other hand, high dose saturates the detoxication pathways of APAP due to glucuronidation and sulfation insufficiency. Thus, excessive drugs accumulations become accessible for metabolism by cytochrome P450, which leads to GHS depletion. Exhausted GSH levels allow NAPQI free to bind with other targeted cellular proteins which aggravate cellular oxidative stress and indulge in the cellular necrosis process.Citation7,Citation8
Reactive oxygen species (ROS) are strongly associated with oxidative stress.Citation9,Citation10 The equilibrium between ROS generation and the non-enzymatic antioxidant (GSH), superoxide dismutase (SOD), catalase (CAT) notion of being important for the APAP-induced toxicity.Citation5,Citation11
Farnesoid X receptor (FXR) or NR1H4 is a nuclear receptor transcript or factor play a vital role in the regulation of bile acids, cholesterol, glucose metabolism, and lipoproteins. It has been reported that FXR activation could be beneficial in the APAP-induced hepatic toxicity.Citation12 Moreover, several other antioxidants such as liver fatty acid binding protein (L-FABP), with its methionine and cysteine amino acids moiety defense the cells against the oxidative stress by quenching free radicals.Citation13,Citation14 It has been demonstrated that recombinant rat L-FABP can be effective cellular antioxidant against hydrophilic or lipophilic free radical generator.Citation15 Naval renal prognostic biomarker, i.e., Kidney Injury Molecule (KIM-1) belongs to trans-membrane globular protein plays a central role in the phagocytic clearance of apoptotic and necrotic cells in renal injury.Citation16,Citation17
Pharmacotherapy for the management of APAP-induced hepatic and renal toxicity is multivariate as many interactive pathways are involved in their pathophysiology. It has been shown that administration of gadolinium chloride or dextran sulfate played a vital role in the macrophages inactivation whereas aminoguanidine, a nitric oxide (NO) synthase inhibitor also utilized for the amelioration of APAP-induced toxicity.Citation18,Citation19 Treatments with zinc sulfate and lobenzarit are other treatment options for APAP-induced toxicity that improved hepatic GSH levels.Citation20 Intravenous administration of N-acetylcysteine is also an early treatment option for APAP poisoning.Citation21
In Ayurveda, plenty of medicinal plants have been recommended as a treatment option for the renal and hepatic diseases.Citation22 Moreover, >600 commercial preparations with hepatoprotective potential are available all over the world which contains ∼100 medicinal plants from Ayurveda.Citation23 But, due to lack of scientific validation of efficacy and safety, these medicinal plants are often ignored by many and considered to be an alternative to conventional medicine. Therefore, their systematic scientific validation in terms of biological assays, animal models, clinical trials, chemical standardization as well as documentation is today’s need of hours.Citation24,Citation25 The medicinal plant based drugs have the added advantage of being simple, effective and offering a broad spectrum of activity with an emphasis on the preventive actions of drugs. Because of these factors, the demand for plant-based medicines (photomedicine and phytopharmaceutical) is increasing worldwide.Citation26
Bioflavonoids considered as a potent bioactive moiety against free radical and oxidative stress.Citation27–30 Naringin is a polyphenolic bioflavonoid compound derived from citrus fruits and related grapefruits.Citation31 Oral naringin intake gets converted into naringenin, an absorbable form through α-rhamnosidase and β-glucosidase enzymes.Citation32 Naringin possesses multiple biological and pharmacological properties such as lipid alleviating, anti-apoptotic, anti-diabetic, neuroprotective, anti-inflammatory, wound healing, metal chelating, anti-microbial, anti-mutagenic, anti-cancer, free radical trapping, and antioxidant properties.Citation27,Citation30,Citation33–36 Moreover, our laboratory has recently demonstrated that naringin may exert its free radical scavenging and antioxidative effect in sodium arsenite-induced renal and hepatic toxicity.Citation37 To best of our knowledge, no study has been carried out on the effect of naringin on APAP-induced hepatic and renal toxicity. We, therefore, evaluated the protective effect of naringin on APAP-induced renal and hepatotoxicity via examination of FXR, L-FABP, KIM-1, and other antioxidants as well as serum prognostic markers in Wistar albino rats.
Materials and methods
Animals
Adult male Wistar rats (180–220 g) were obtained from the National Institute of Biosciences, Pune, India. They were housed in cages in a facility maintained at 24 ± 1 °C, with a relative humidity of 45–55% and 12:12 h dark/light cycle. The animals had free access to standard pellet chow (Pranav Agro Industries Ltd., Sangli, India) and filtered water throughout the experimental period. All experiments were carried out between 09:00 and 17:00 h. The experimental protocol was approved (CPCSEA/67/2012) by the Institutional Animal Ethics Committee (IAEC) of Poona College of Pharmacy, Pune, India, and performed in accordance with the guidelines of Committee for Control and Supervision of Experimentation on Animals (CPCSEA), Government of India. Animals were transferred to testing laboratory 1 h before the experiment for adaptation.
Chemicals
Acetaminophen and silymarin were obtained as gift samples from Symed Pharmaceutical Pvt. Ltd, Hyderabad, India. Naringin (≥ 95% by HPLC) was purchased from Sigma Chemical Co. (St Louis, MO). 1,1′,3,3′-Tetraethoxypropane, crystalline beef liver CAT, reduced GSH, and 5,5′-dithiobis (2-nitrobenzoic acid) were purchased from SD Fine Chemicals, Mumbai, India. Sulfanilamides, naphthylamine diamine HCl, and phosphoric acid were obtained from Loba Chemi Pvt. Ltd., Mumbai, India.
Induction of APAP-induced toxicity and drug treatment schedule
The selection of dose for APAP was based on the studies carried out previously.Citation38 Silymarin was administered to rats orally at a dose of 25 mg/kg for 14 days.Citation39 APAP was administered to rats orally at a dose of 700 mg/kg.Citation38 Fasted rats were randomly divided into seven groups of six rats as follows:
Group I: Normal control: rats were administered a single daily dose of distilled water (10 unit), p.o. for 14 days.
Group II: APAP: rats that served as the model control was administered a single daily dose of distilled water (10 mg/kg, p.o.) 2 h before oral administration APAP suspension (700 mg/kg) for 14 days.
Group III: Silymarin (25): rats were administered a single daily dose of silymarin (25 mg/kg, p.o.) in distilled water, 2 h before oral administration APAP suspension (700 mg/kg) for 14 days.
Group IV: Naringin (20 mg/kg) [N (20)]: rats were administered single daily dose of Naringin (20 mg/kg, p.o.) 2 h before oral administration APAP suspension (700 mg/kg) for 14 days.
Group V: Naringin (40 mg/kg) [N (40)]: rats were administered single daily dose of Naringin (40 mg/kg, p.o.) 2 h before oral administration APAP suspension (700 mg/kg) for 14 days.
Group VI: Naringin (80 mg/kg) [N (80)]: rats were administered single daily dose of Naringin (80 mg/kg, p.o.) 2 h before oral administration APAP suspension (700 mg/kg) for 14 days.
Group VII: Per se: rats were administered a single daily dose of Naringin (80 mg/kg, p.o.) for 14 days. However, it did not receive any oral administration APAP suspension.
After 14-day treatment, rats fasted overnight and, after 24 h, they were sequentially anesthetized with anesthetic ether for ∼30–40 s. The blood was withdrawn by the retro-orbital puncture. Each blood sample was collected into separate vials for the determination of serum parameters. After blood collection, the animals were sacrificed by cervical dislocation and then liver as well as kidney were removed. The specimens were divided into two portions: one portion was used for biochemical estimation, and another portion was processed for histopathological examination.
Serum biochemistry
The serum was separated by centrifugation using an Eppendorf cytocentrifuge (model no. 5810, Eppendorf, Hamburg, Germany), maintained at 4 °C and run at a speed of 7000 rpm for 15 min. The levels of serum albumin, alkaline phosphatase (ALP), blood urea nitrogen (BUN), cholesterol, creatinine, direct bilirubin, high-density lipoprotein (HDL), lactate dehydrogenase (LDH), low-density lipoprotein (LDL), aspartate transaminase (AST), alanine transaminase (ALT), triglyceride, total bilirubin, and uric acid were measured by a spectrophotometer (UV-visible spectrophotometer, Jasco V-530, Tokyo, Japan) using commercially available reagent kits according to procedure provided by manufacturer (Accurex Biomedical Pvt. Ltd., Mumbai, India).
Biochemical estimations
Preparation of tissue homogenate
For liver and kidney homogenization, tissue segments were mixed with 0.1 M phosphate buffer and homogenized on ice for 60 s at 10 000 rpm in a homogenizer (Remi Equipment Pvt. Ltd., Remi Motors Ltd., Mumbai, India). Supernatant of tissue homogenates was employed to estimate SOD, reduced GSH, lipid peroxidation [malondialdehyde (MDA) content], and NO content.
Determination of total protein, SOD, GSH, MDA, and NO
The level of total protein, SOD, GSH, MDA, and NO in liver and kidney homogenate was determined according to earlier reported methods.Citation40–45
Reverse transcriptase-polymerase chain reaction (RT-PCR) analysis
Total RNA was extracted from liver and kidney tissues according to earlier reported methods.Citation46,Citation47 The polymerase chain reaction mixture was amplified in a DNA thermal cycler (Eppendorf India Ltd, Chennai) by using gene specific primers. The primer sequences for FXR, KIM-1, and β-actin were selected according to the previously reported methodCitation17 (Supplementary file). PCR products were run on 1% agarose gels, stained with ethidium bromide. The expression of all the genes was assessed by generating densitometry data for band intensities in different sets of experiments and was generated by analyzing the gel images in the Image J program (Version 1.33, Wayne Rasband, National Institutes of Health (NIH), Bethesda, MD). The band intensities were compared with constitutively expressed β-actin which served as a control for sample loading and integrity. The intensity of mRNAs was standardized against that of the β-actin mRNA from each sample and the results are expressed as PCR-product/β-actin mRNA ratio.
Histopathological examination
Liver and kidney tissues were stored in 10% formalin for 24 h. The specimen was dehydrated and placed in xylene for 1 h (3 times) and later in ethyl alcohol (70%, 90% and 100%) for 2 h. The infiltration and impregnation were carried out by treating with paraffin wax twice, each time for 1 h. Tissue specimens were cut into sections of 3–5 mm thickness and were stained with hematoxylin and eosin (H&E). The specimen was mounted on the slide by use of distrain phthalate xylene as a mounting medium. Sections were examined under a light microscope for the inspection of the histopathology features of specimen and infiltration of cells. The various changes in histological features were graded as Grade 0 (not present or very slight), Grade 1 (mild), Grade 2 (moderate), and Grade 3 (severe) as described earlier.Citation37,Citation48
Statistical analysis
Data were expressed as mean ± SEM. Data analysis was performed using software (v 5.0, Graph Pad, San Diego, CA). Data of biochemical parameters were analyzed using one-way analysis of variance (ANOVA) and followed by Tukey’s multiple range tests for each parameter separately. A value of p < 0.05 was considered to be statistically significant.
Results
Effect of naringin treatment on APAP-induced alteration in body weight and relative organ weight in rats
There was significant (p < 0.05) decrease in body weight of APAP rats as compared to normal rats. Whereas relative organ weights of liver and spleen were significantly (p < 0.05) increased in APAP rats as compared with normal rats. Treatment with naringin (40 and 80 mg/kg, p.o.) showed a significant increase in body weight as compared to APAP rats whereas, relative liver weight, as well as relative spleen weight, were significantly (p < 0.05) decreased as compared to APAP rats. Administration of silymarin (25 mg/kg, p.o.) for 14 days significantly (p < 0.05) increased body weight as compared to APAP rats whereas relative liver weight and spleen weight were significantly (p < 0.05) decreased as compared to APAP rats. There was no significant difference in the body weight and relative organ weight in normal as well as per se treated group ().
Table 1. Effect of naringin treatment on body weight, relative liver weight, relative spleen weight, albumin, blood urea nitrogen, serum creatinine and uric acid in APAP-induced toxicity in rats.
Effect of naringin treatment on APAP-induced alteration in serum albumin, blood urea nitrogen, serum creatinine and serum uric acid in rats
The level of serum albumin and serum uric acid were significantly (p < 0.05) decreased in APAP rats as compared to normal rats whereas BUN and serum creatinine level were significantly (p < 0.05) increased in the APAP rats as compared to normal rats. Chronic administration of naringin (40 and 80 mg/kg, p.o.) significantly increased serum albumin (p < 0.05) and serum uric acid (p < 0.05) level whereas the level of BUN and serum creatinine were significantly (p < 0.05) decreased by naringin treatment (40 and 80 mg/kg, p.o.) as compared to APAP control rats. When compared with APAP rats, the serum albumin and serum uric acid levels were significantly (p < 0.05) increased in silymarin (25 mg/kg, p.o.) treated rats whereas the levels of BUN, and serum creatinine were significantly (p < 0.05) decreased. However, serum albumin, BUN, serum creatinine and serum uric did not differ significantly in normal as well as per se treated group ().
Effect of naringin treatment on APAP-induced alteration in alkaline phosphatase, total bilirubin, direct bilirubin, AST and ALT in rats
Chronic administration of APAP caused significant (p < 0.05) increase in the level of alkaline phosphatase, total bilirubin, direct bilirubin, AST and ALT in APAP rats as compared to normal rats. When compared with APAP rats, there was significant (p < 0.05) decrease in the level of alkaline phosphatase, total bilirubin, direct bilirubin, AST and ALT after chronic naringin treatment (40 and 80 mg/kg, p.o). Silymarin (25 mg/kg, p.o.) administration significantly (p < 0.05) reduced these elevated levels of alkaline phosphatase, total bilirubin, direct bilirubin, AST and ALT when compared with APAP rats ().
Table 2. Effect of naringin treatment on alkaline phosphatase, total bilirubin, direct bilirubin, AST and ALT in APAP-induced toxicity in rats.
Effect of naringin treatment on APAP-induced alteration in serum cholesterol, HDL, LDL, LDH and triglyceride in rats
There were significant (p < 0.05) increase in the level of serum cholesterol, LDL, LDH and triglyceride in APAP rats as compared to normal rats. Chronic administration of APAP significantly (p < 0.05) decreased the level of HDL in APAP rats as compared to normal rats. The level of serum HDL was significantly (p < 0.05) increased by the chronic treatment with naringin (40 and 80 mg/kg, p.o.) when compared with APAP rats. Moreover, administration of naringin (40 and 80 mg/kg, p.o.) significantly (p < 0.05) prevented APAP caused an increase in the level of serum cholesterol, LDL, LDH and triglyceride as compared to APAP rats. Silymarin (25 mg/kg, p.o.) significantly (p < 0.05) decreased the elevated levels of serum cholesterol, LDL, LDH and triglyceride as compared to APAP rats. However, administration of silymarin (25 mg/kg, p.o.) did not produce any significant change in the level of HDL as compared to APAP rats. The levels of serum cholesterol, HDL, LDL, LDH and triglyceride after APAP administration did not differ significantly in per se group as compared to normal group ().
Table 3. Effect of naringin treatment on cholesterol, HDL, LDL, LDH and triglyceride in APAP-induced toxicity in rats.
Effect of naringin treatment on APAP-induced alteration in hepatic as well as renal SOD and GSH in rats
Chronic administration of APAP for 14 days resulted in significant (p < 0.05) decrease in the level of SOD and GSH in hepatic as well as renal tissue in APAP rats as compared to normal rats. Treatment with naringin (40 and 80 mg/kg, p.o.) showed significant elevation (p < 0.05) in the level of hepatic SOD and renal SOD as well as hepatic GSH and renal GSH as compared to APAP rats. Silymarin (25 mg/kg, p.o.) showed significant (p < 0.05) increase in level of hepatic SOD, renal SOD and renal GSH as compared to APAP rats but, it failed to produce any significant increase in the level of hepatic GSH as compared to APAP rats. The level of SOD and GSH in hepatic as well as renal tissue did not significantly differ in per se group as compared to normal group ().
Figure 1. Effect of naringin treatment on hepatic and renal SOD (A), GSH (B), MDA (C) and NO (D) in APAP-induced toxicity in rats. Data are expressed as mean ± SEM (n = 6) and analyzed by one-way ANOVA followed by Tukey’s multiple range test for each parameter separately. *p < 0.05 as compared to APAP group, #p < 0.05 as compared to normal group and $p < 0.05 as compared to one another group. APAP: acetaminophen; N (20): naringin (20 mg/kg) treated; N (40): naringin (40 mg/kg) treated; N (80): naringin (80 mg/kg) treated; SOD: superoxide dismutase; GSH: glutathione; MDA: malondialdehyde; NO: nitric oxide.
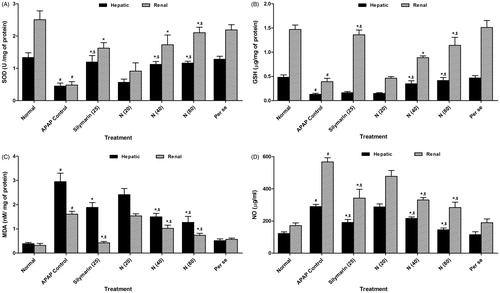
Effect of naringin treatment on APAP-induced alteration in hepatic as well as renal MDA and NO in rats
There was significant increase (p < 0.05) in the levels of hepatic MDA and NO as well as renal MDA and NO in APAP rats as compared to normal rats. Treatment with naringin (40 and 80 mg/kg, p.o.) showed a significant decrease (p < 0.05) in hepatic and renal MDA as well as hepatic and renal NO levels as compared to APAP rats. When compared with APAP rats, silymarin (25 mg/kg, p.o.) treated rats showed a significant decrease (p < 0.05) in the level of hepatic as well as renal MDA and NO. However, per se treated group did not show any significant alterations in hepatic as well as renal MDA and NO as compared to normal rats ().
Effect of naringin treatment on APAP-induced alteration in hepatic FXR and renal KIM-1 mRNA expression in rats
There was significant decrease (p < 0.05) hepatic FXR mRNA expression whereas renal KIM-1 mRNA expression was increased (p < 0.05) in APAP rats as compared to normal rats. Treatment with naringin (40 and 80 mg/kg, p.o.) showed significant amelioration (p < 0.05) in the alterations induced in hepatic FXR and renal KIM-1 mRNA expression as compared to APAP rats. When compared with APAP rats, silymarin (25 mg/kg, p.o.) treated rats showed a significant increase (p < 0.05) in hepatic FXR mRNA expression whereas there was significant decrease (p < 0.05) in renal KIM-1 mRNA expression. However, per se treated group did not show any significant alterations in hepatic FXR and renal KIM-1 mRNA expression as compared to normal rats (.
Figure 2. Effect of naringin treatment on liver FXR mRNA expression (A), renal KIM-1 mRNA expression (B) in APAP-induced toxicity in rats. Data are expressed as mean ± SEM (n = 6) and analyzed by one-way ANOVA followed by Tukey’s multiple range test for each parameter separately. *p < 0.05 as compared to APAP group, #p < 0.05 as compared to normal group and $p < 0.05 as compared to one another group. APAP: acetaminophen; FXR: farnesoid X receptor; KIM-1: kidney injury molecule-1.
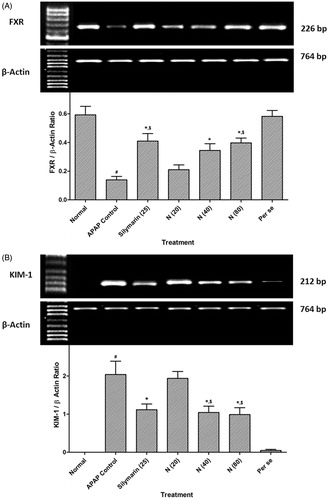
Effect of naringin treatment on APAP-induced pathological alteration in rat liver and kidney
In the histopathological studies, normal control, as well as per se animals, showed normal central vein in liver parenchymal cells and without any signs of inflammation as well as necrosis in cells (, respectively). However, there was evidence of congestion (grade 1). The histopathological examination of liver from APAP administered rats was showed inflammatory cells (grade 4) and shrunken hepatocytes with chromatin condensation. Administration of APAP caused hepatocellular injury reflected by the presence of diffuse cytoplasmic vacuolation (grade 3), centrilobular necrosis (grade 4), vascular congestion (grade 3) and nuclear pyknosis (grade 3) of the hepatocytes. Macrovesicular fatty changes (grade 2) were also evident in liver histological observation of APAP rats (). However, silymarin (25 mg/kg) showed moderate histopathological changes in liver marked by sinusoidal congestion (grade 2), cytoplasmic vacuolation (grade 1) and the presence of only a few inflammatory cells (grade 1) along with few fatty globules (grade 1) (). In the naringin (40 mg/kg, p.o.) treated rats, the histology of liver showed a mild degree of vacuolization (grade 1), necrosis (grade 1) and congestion (grade 1) around central vein (). Moderate inflammatory cells with few plasma cells (grade 2) were present in naringin (80 mg/kg, p.o.) treated rats () ().
Figure 3. Effect of naringin treatment on APAP-induced pathological alteration in rat liver. Photomicrograph of sections of liver of normal (A), APAP controls (B), silymarin (25 mg/kg) treated (C), naringin (40 mg/kg) treated (D), naringin (80 mg/kg) treated (E) and per se treated (F) rats. Inflammatory infiltration (black arrow), edema (yellow arrow), congestion (green arrow) and necrosis (red arrow). H&E staining at 40× and 100 × (inset).
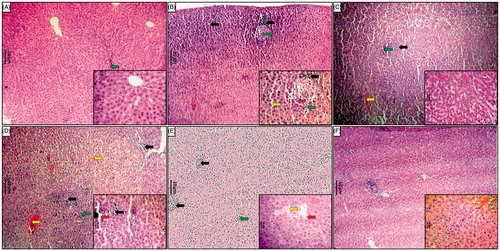
Table 4. Effect of naringin treatment on APAP-induced pathological alteration in rat hepatic and renal histology.
showed the normal architecture of kidney from normal as well per se group. Chronic administration of APAP caused renal damage evident by glomerular structural disruption (grade 4) and partial endothelial rupture in the capsule. APAP animals showed the presence of intraluminal cell debris, karyorrhexis and pyknosis (grade 2) indicating cell death (). Treatment with silymarin (25 mg/kg) as well as naringin (80 mg/kg, p.o.) showed a moderate number of inflammatory (grade 1) and apoptotic cells (). Naringin (40 mg/kg, p.o.) treated rats showed mild architectural damage with few intraluminal cell debris (grade 2) and infiltration of inflammatory cells (grade 2) as compared to APAP () ().
Figure 4. Effect of naringin treatment on APAP-induced pathological alteration in rat kidney. Photomicrograph of sections of kidney of normal (A), APAP controls (B), silymarin (25 mg/kg) treated (C), naringin (40 mg/kg) treated (D), naringin (80 mg/kg) treated (E) and per se treated (F) rats. Glomerular hypertrophy (blue arrow), inflammatory infiltration (black arrow), edema (yellow arrow), congestion (green arrow) and necrosis (red arrow). H&E staining at 40× and 100× (inset).
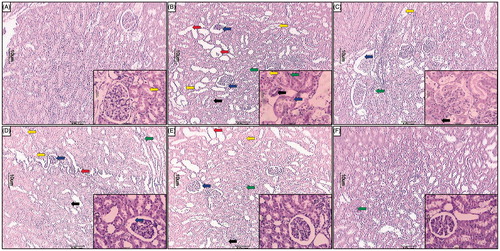
Discussion
Clinically, it has been found that high dose of APAP tightly associated with hepatic and renal calamity.Citation49,Citation50 Naringin has been reported to possess potent antioxidant, anti-hypercholesterolemic, free radical scavenging, metal chelating and anticancer properties.Citation51,Citation52 The aim of present study was to investigate the role of naringin against APAP-induced hepatic toxicity and renal toxicity. We observed that the APAP administration caused hepatic and renal toxicity by disturbing its antioxidant status, lipid profiles and histomorphological architecture.
Antioxidant such as SOD enzyme and GSH, a non-enzymatic antioxidant are the first line defense system that confines the toxicity allied with the free radicals.Citation53–56 Antioxidants and ROS balance might be important for deletion of oxidative stress in intracellular organelles.Citation57–59 Nonetheless, in the APAP-induced toxicity, ROS generation enormously deter this balance with an enhanced demand on the antioxidant protection system. Moreover, Lipid peroxidation associated with the increased free radicals production and/or decreased antioxidant defense system activities.Citation60–62 Several lines of studies suggested that free radical scavenging enzymes, i.e., SOD and GSH decreased and MDA, a lipid peroxidation end product increased when administered with APAP.Citation11 In line with previous studiesCitation63,Citation64 the present study also found significantly decreased SOD and GSH levels as well as increased MDA level during APAP treatment in the hepatic and renal tissue. Naringin treatment significantly enhanced tissue SOD and GSH as well as decreased MDA levels, demonstrating that naringin could maintain the abnormal antioxidant and ROS generation, which in turn modulate the membrane integrity against the APAP-induced cellular injury.
NO with O2− form peroxynitrite anion, high production exerts toxic effect against the antioxidant defense mechanism cause cellular disruption through apoptosis and necrosis.Citation65–68 The present study demonstrated that higher dose of APAP resulted in significantly increased hepatic and renal NO levels as compared to normal rats. It in agreement with the number of previous studies which showed that generation of peroxynitrite free radical due to increase in NO levels might be responsible for the oxidative stress in APAP-induced hepatic and renal toxicity.Citation69–71 In addition, earlier reports suggested that enhanced NO levels also attributed with GSH depletion.Citation72–74 APAP-induced oxidative stress significantly decreased by naringin treatment via attenuation of increased hepatic and renal NO levels.
ALT and AST are important endogenous diagnostic biomarkers for hepatic toxicity. These two important enzymes found in high concentration in the cytoplasm and during hepatic insult leaks into the blood stream in conformity with the extent of hepatotoxicity. An increased concentration of AST and ALT in serum is an indicator of cellular leakage and loss of functional integrity of cell membrane in liver.Citation75 In the current study, elevated ALT and AST levels in serum are the results of APAP-induced liver impairment. In our histopathological examination, we found fat accumulation and centrilobular necrosis of liver tissue of APAP rats. It has been also documented that APAP-induced hepatotoxicity caused ALT and AST overproduction due to hepatic parenchymal injury and increased alkaline phosphatase (ALP) synthesis.Citation23,Citation38 An earlier report also suggested that bile canaliculi cell lining caused ALP discharge in response to cholestasis and increased biliary pressure. Furthermore, serum bilirubin including total as well as direct bilirubin considered as a prophetic marker for the investigation of hepatic abnormalities. Liver parenchymal cells consumed bilirubin from the blood and in the presence of glucuronyl transferase enzymes conjugates with glucuronic acid and then excreted into bile. Hyperbilirubinemia is an important consequence of excessive heme destruction and biliary tract obstruction. Previous studies revealed that hepatic parenchymal cells mishap are the main reason for the increased total and direct serum bilirubin.
LDH enzymes generally occur in the cytoplasmic cells and ooze out into the blood during apoptotic and necrotic cell death.Citation30,Citation76 Serum LDH considered as a prognostic marker for the investigation of hepatic injury.Citation77 On the other hand, serum creatinine, uric acid, BUN also play very important role in the diagnosis of renal impairment.Citation78 In the present study, APAP administration resulted in significantly increased LDH, creatinine, and BUN while, serum uric acid level was significantly decreased. These findings were significantly corroborated with the previous finding.Citation38 In the current study, APAP administration significantly increases the serum total cholesterol, LDL, and triglycerides as well as decreases the HDL level. This result comes in agreement with the result of the previous investigator.Citation64 In the past, it has been mentioned that APAP causes impairment in lipoprotein and cholesterol metabolism.Citation79 Naringin treatment showed remarkable attenuation of altered serum lipid profile level which is in line with the previous investigation.Citation80
Farnesoid X receptor also known as NR1H4, a ligand-activated transcriptor factor belonging to a category of nuclear receptor super-family. FXR greatly expressed in the liver, kidney, intestine and adrenal glandsCitation81 and involved in the expression of various proteins and biosynthetic enzymes which are important for the physiological maintenance of cholesterol and bile acid homeostasis.Citation82 It has been reported that FXR activation drop off the levels of liver bile acids, turn on Foxm1b and other genes responsible for the progression of cell cycle and stimulate liver regeneration.Citation83 Previously; it has been suggested that FXR agonist treatment significantly protects the liver through FXR activation from APAP-induced toxicity in mice model.Citation12 In addition, hepatic steatosis and hypertriglyceridemia developed in FXR-deficient mice reflect the key role of FXR in the regulation of hepatic lipid metabolism.Citation84 Similarly, in the present study, we also found that APAP-induced hepatic toxicity demur the expression of FXR, which was in accordance with the previous finding.Citation12 Naringin treatment notably increases the FXR expression in APAP-induced hepatic toxicity as compared to APAP control group.
Liver fatty acid binding protein (L-FABP), an endogenous antioxidant occurs inside the cytoplasm and nucleus of hepatocytes as well as also expressed in various tissues, such as kidney, intestine and heart.Citation85 L-FABP bind with polyunsaturated fatty acids and long chain fatty acid peroxidation products, control the accessibility of fatty acids to oxidative pathways and thus monitor the ROS release.Citation86,Citation87 It has been demonstrated that L-FABP could act as an antioxidant through its free radical scavenging property.Citation88 In addition, the previous report also explained that L-FABP considered as a cytoprotective agent against oxidative stress in APAP-induced toxicity model. It has been well reported that APAP-induced toxicity may be associated with ROS generation.Citation18 In our animal study, results suggest that APAP ingestion causes antioxidant depletion might be responsible for the decreased L-FABP due to excess generation of ROS. Till now, best of our knowledge this is the first animal study to explore the role of L-FABP in APAP-induced toxicity. Further studies on the role of L-FABP in APAP-induced toxicity are required. Treatment with naringin significantly increased the L-FABP, suggesting that this effect might contribute to its protective effect against APAP-induced oxidative stress.
Ischemic or toxic insult, a series of events including loss of cytoskeleton integrity, apoptotic and necrotic cell casualty, cells proliferation and dedifferentiation undergoes in renal proximal tubule cells. KIM-1 with its unique feature, such as dedifferentiation of regenerating cells is one of the striking pathological manifestations for the kidney injury.Citation89–91 Recently, in a mice model it has been shown that APAP-induced renal toxicity increases KIM-1 expression.Citation11 Similarly, in our study, we also observed significantly increased KIM-1 expression in APAP-induced renal toxicity. The exact mechanism behind the enhanced KIM-1 expression in APAP-induced renal toxicity is still not elucidated, but from our histopathological investigation, we can conclude that elevated KIM-1 expression in the renal tissue could be due to oxidative stress followed by necrotic cell death. Whereas, administration of naringin appreciably ameliorated the APAP-induced increases in renal KIM-1expression.
Conclusion
In conclusion, chronic APAP ingestion caused oxidative impairment in the hepatic and renal tissue of the experimental animals, and that could be averted by the treatment of naringin. Protective role of naringin could be due to its antioxidant, membrane stabilizing as well as anti-hyperlipidemic actions exerted through modulation of FXR and KIM-1 mRNA expression. In addition, results also suggest that naringin might be beneficial for the finding of therapeutic potential against APAP-induced hepatic and renal toxicity.
Acknowledgments
The authors acknowledge Dr. S. S. Kadam, Vice Chancellor, Bharati Vidyapeeth University and Dr. K. R. Mahadik, Principal, Poona College of Pharmacy for keen interest and providing the necessary facilities to carry out the study.
Disclosure statement
There is no conflict of interest between any of the authors.
References
- Gum SI, Cho MK. Recent updates on acetaminophen hepatotoxicity: The role of nrf2 in hepatoprotection. Toxicol Res. 2013;29:165–172.
- Smilkstein MJ, Douglas DR, Daya MR. Acetaminophen poisoning and liver function. N Engl J Med. 1994;331:1310–1311. author reply 1311-1312.
- Whitcomb Dc, Block Gd. Association of acetaminophen hepatotoxicity with fasting and ethanol use. JAMA. 1994;272:1845–1850.
- Jaeschke H, McGill MR, Ramachandran A. Oxidant stress, mitochondria, and cell death mechanisms in drug-induced liver injury: Lessons learned from acetaminophen hepatotoxicity. Drug Metab Rev. 2012;44:88–106.
- McGill MR, Williams CD, Xie Y, Ramachandran A, Jaeschke H. Acetaminophen-induced liver injury in rats and mice: Comparison of protein adducts, mitochondrial dysfunction, and oxidative stress in the mechanism of toxicity. Toxicol Appl Pharmacol. 2012;264:387–394.
- Nelson SD. Metabolic activation and drug toxicity. J Med Chem. 1982;25:753–765.
- Jollow DJ, Mitchell JR, Potter WZ, Davis DC, Gillette JR, Brodie BB. Acetaminophen-induced hepatic necrosis. II. Role of covalent binding in vivo. J Pharmacol Exp Ther. 1973;187:195–202.
- Shon YH, Nam KS. Protective effect of moutan cortex extract on acetaminophen-induced cytotoxicity in human Chang liver cells. Biol Pharm Bull. 2002;25:1427–1431.
- Adil M, Visnagri A, Kumar VS, Kandhare AD, Ghosh P, Bodhankar SL. Protective effect of naringin on sodium arsenite induced testicular toxicity via modulation of biochernical perturbations in experimental rats. Pharmacologia. 2014;5:222–234.
- Ghule AE, Kandhare AD, Jadhav SS, Zanwar AA, Bodhankar SL. Omega-3-fatty acid adds to the protective effect of flax lignan concentrate in pressure overload-induced myocardial hypertrophy in rats via modulation of oxidative stress and apoptosis. Int Immunopharmacol. 2015;28:751–763.
- Naguib YM, Azmy RM, Samaka RM, Salem MF. Pleurotus ostreatus opposes mitochondrial dysfunction and oxidative stress in acetaminophen-induced hepato-renal injury. BMC Complement Altern Med. 2014;14:494.
- Lee FY, de Aguiar Vallim TQ, Chong HK, et al. Activation of the farnesoid X receptor provides protection against acetaminophen-induced hepatic toxicity. Mol Endocrinol. 2010;24:1626–1636.
- Bassuk JA, Tsichlis PN, Sorof S. Liver fatty acid binding protein is the mitosis-associated polypeptide target of a carcinogen in rat hepatocytes. Proc Natl Acad Sci USA. 1987;84:7547–7551.
- Kandhare AD, Bodhankar SL, Mohan V, Thakurdesai PA. Prophylactic efficacy and possible mechanisms of oligosaccharides based standardized fenugreek seed extract on high-fat diet-induced insulin resistance in C57BL/6 mice. J Appl Pharm Sci. 2015;5:035–045.
- Chen A, Tang Y, Davis V, et al. Liver fatty acid binding protein (L-Fabp) modulates murine stellate cell activation and diet-induced nonalcoholic fatty liver disease. Hepatology. 2013;57:2202–2212.
- Ichimura T, Asseldonk EJ, Humphreys BD, Gunaratnam L, Duffield JS, Bonventre JV. Kidney injury molecule-1 is a phosphatidylserine receptor that confers a phagocytic phenotype on epithelial cells. J Clin Invest. 2008;118:1657–1668.
- Visnagri A, Kandhare AD, Bodhankar SL. Renoprotective effect of berberine via intonation on apoptosis and mitochondrial-dependent pathway in renal ischemia reperfusion-induced mutilation. Ren Fail. 2015;37:482–493.
- Michael SL, Pumford NR, Mayeux PR, Niesman MR, Hinson JA. Pretreatment of mice with macrophage inactivators decreases acetaminophen hepatotoxicity and the formation of reactive oxygen and nitrogen species. Hepatology. 1999;30:186–195.
- Gardner CR, Heck DE, Yang CS, et al. Role of nitric oxide in acetaminophen-induced hepatotoxicity in the rat. Hepatology. 1998;27:748–754.
- Armesto J, Frutos N, Gonzalez R, Pascual C. In vitro activation of hepatic glutathione reductase from mice by lobenzarit disodium. Agents Actions. 1993;39:69–71.
- Prescott LF. The third Lilly Prize Lecture. University of London, January, 1979. The nephrotoxicity and hepatotoxicity of antipyretic analgesics. Br J Clin Pharmacol. 1979;7:453–462.
- Subramoniam A, Pushpangadan P. Development of phytomedicines for liver disease. Indian J Pharmacol. 1999;31:166–175.
- Ahmed B, Alam T, Khan SA. Hepatoprotective activity of Luffa echinata fruits. J Ethnopharmacol. 2001;76:187–189.
- Yuan R, Lin Y. Traditional Chinese medicine: An approach to scientific proof and clinical validation. Pharmacol Ther. 2000;86:191–198.
- Kandhare AD, Raygude KS, Ghosh P, Gosavi TP, Bodhankar SL. Patentability of animal models: India and the Globe. Int J Pharm Biol Arch. 2011;2:1024–1032.
- Dole S, Kandhare AD, Ghosh P, Gosavi TP, Bodhankar SL. The health outcome after homeopathic treatment in cases of BPH and LUTS: A prospective clinical study. J Pharm Biomed Sci. 2012;22:1–7.
- Kandhare AD, Alam J, Patil MV, Sinha A, Bodhankar SL. Wound healing potential of naringin ointment formulation via regulating the expression of inflammatory, apoptotic and growth mediators in experimental rats. Pharm Biol. 2016;54419–432.
- Visnagri A, Kandhare AD, Chakravarty S, Ghosh P, Bodhankar SL. Hesperidin, a flavanoglycone attenuates experimental diabetic neuropathy via modulation of cellular and biochemical marker to improve nerve functions. Pharm Biol. 2014;52:814–828.
- Sarkar A, Sengupta A, Mukhrjee A, et al. Antiulcer potential of morin in acetic acid-induced gastric ulcer via modulation of endogenous biomarkers in laboratory animals. Pharmacologia. 2015;6:273–281.
- Kumar VS, Rajmane AR, Adil M, Kandhare AD, Ghosh P, Bodhankar SL. Naringin ameliorates acetic acid induced colitis through modulation of endogenous oxido-nitrosative balance and DNA damage in rats. J Biomed Res. 2014;28:132–145.
- Jagetia GC, Reddy TK. The grapefruit flavanone naringin protects against the radiation-induced genomic instability in the mice bone marrow: A micronucleus study. Mutat Res. 2002;519:37–48.
- Kim DH, Jung EA, Sohng IS, Han JA, Kim TH, Han MJ. Intestinal bacterial metabolism of flavonoids and its relation to some biological activities. Arch Pharm Res. 1998;21:17–23.
- Jeon SM, Park YB, Choi MS. Antihypercholesterolemic property of naringin alters plasma and tissue lipids, cholesterol-regulating enzymes, fecal sterol and tissue morphology in rabbits. Clin Nutr. 2004;23:1025–1034.
- Kim HJ, Song JY, Park HJ, Park HK, Yun DH, Chung JH. Naringin protects against rotenone-induced apoptosis in human neuroblastoma SH-SY5Y cells. Korean J Physiol Pharmacol. 2009;13:281–285.
- Kandhare AD, Ghosh P, Bodhankar SL. Naringin, a flavanone glycoside, promotes angiogenesis and inhibits endothelial apoptosis through modulation of inflammatory and growth factor expression in diabetic foot ulcer in rats. Chem Biol Interact. 2014;219:101–112.
- Kandhare AD, Raygude KS, Ghosh P, Ghule AE, Bodhankar SL. Neuroprotective effect of naringin by modulation of endogenous biomarkers in streptozotocin induced painful diabetic neuropathy. Fitoterapia. 2012;83:650–659.
- Adil M, Kandhare AD, Visnagri A, Bodhankar SL. Naringin ameliorates sodium arsenite-induced renal and hepatic toxicity in rats: Decisive role of KIM-1, Caspase-3, TGF-β, and TNF-α. Ren Fail. 2015;37:1396–1407.
- Ahmad ST, Arjumand W, Nafees S, et al. Hesperidin alleviates acetaminophen induced toxicity in Wistar rats by abrogation of oxidative stress, apoptosis and inflammation. Toxicol Lett. 2012;208:149–161.
- Hegde K, Joshi AB. Hepatoprotective and antioxidant effect of Carissa spinarum root extract against CCl4 and paracetamol-induced hepatic damage in rats. Bangladesh J Pharmacol. 2010;5:73–76.
- Gosavi TP, Kandhare AD, Ghosh P, Bodhankar SL. Anticonvulsant activity of Argentum metallicum, a homeopathic preparation. Der Pharmacia Lettre. 2012;4:626–637.
- Kandhare AD, Raygude KS, Ghosh P, Bodhankar SL. The ameliorative effect of fisetin, a bioflavonoid, on ethanol-induced and pylorus ligation-induced gastric ulcer in rats. Int J Green Pharm. 2011;5:236.
- Kandhare AD, Bodhankar SL, Singh V, Mohan V, Thakurdesai PA. Anti-asthmatic effects of type-A procyanidine polyphenols from cinnamon bark in ovalbumin-induced airway hyperresponsiveness in laboratory animals. Biomed Aging Pathol. 2013;3:23–30.
- Kandhare AD, Ghosh P, Ghule AE, Bodhankar SL. Elucidation of molecular mechanism involved in neuroprotective effect of Coenzyme Q10 in alcohol-induced neuropathic pain. Fundam Clin Pharmacol. 2013;27:603–622.
- Aswar UM, Kandhare AD, Mohan V, Thakurdesai PA. Anti-allergic effect of intranasal administration of type-A procyanidin polyphenols based standardized extract of cinnamon bark in ovalbumin sensitized BALB/c mice. Phytother Res. 2015;29:423–433.
- Badole SL, Chaudhari SM, Jangam GB, Kandhare AD, Bodhankar SL. Cardioprotective activity of pongamia pinnata in streptozotocin-nicotinamide induced diabetic rats. Biomed Res Int. 2015;2015:403291.
- Kandhare AD, Ghosh P, Bodhankar SL. Morin Attenuates Airway Hyperresponsiveness of Allergic Asthma Via Down Regulation of Immune-Inflammatory Biomarkers. Paper presented at: In Proceedings of the 15th International Congress of Immunology (ICI), Milan, Italy, August 22–27, 2013.
- Devkar S, Kandhare A, Zanwar A, et al. Hepatoprotective effect of withanolide rich fraction in acetaminophen intoxicated rat: Decisive role of TNF-α, IL-1β, COX-II, and iNOS. Pharm Biol. 2016. [Epub ahead of print]. doi: 10.3109/13880209.2016.1157193.
- Kandhare AD, Bodhankar SL, Mohan V, Thakurdesai PA. Acute and repeated doses (28 days) oral toxicity study of glycosides based standardized fenugreek seed extract in laboratory mice. Regul Toxicol Pharmacol. 2015;72:323–334.
- Ghosh A, Sil PC. Anti-oxidative effect of a protein from Cajanus indicus L against acetaminophen-induced hepato-nephro toxicity. J Biochem Mol Biol. 2007;40:1039–1049.
- Nelson SD. Molecular mechanisms of the hepatotoxicity caused by acetaminophen. Paper presented at: Semin Liver Dis, 1990.
- Kroyer G. [The antioxidant activity of citrus fruit peels]. Z Ernahrungswiss. 1986;25:63–69.
- Anandhi R, Annadurai T, Anitha TS, et al. Antihypercholesterolemic and antioxidative effects of an extract of the oyster mushroom, Pleurotus ostreatus, and its major constituent, chrysin, in Triton WR-1339-induced hypercholesterolemic rats. J Physiol Biochem. 2013;69:313–323.
- Kamble H, Kandhare AD, Bodhankar SL, Mohan V, Thakurdesai PA. Effect of low molecular weight galactomannans from fenugreek seeds on animal models of diabetes mellitus. Biomed Aging Pathol. 2013;3:145–151.
- Goswami S, Kandhare A, Zanwar AA, et al. Oral l-glutamine administration attenuated cutaneous wound healing in Wistar rats. Int Wound J. 2016;13:116–124.
- Raygude KS, Kandhare AD, Ghosh P, Ghule AE, Bodhankar SL. Evaluation of ameliorative effect of quercetin in experimental model of alcoholic neuropathy in rats. Inflammopharmacology. 2012;20:331–341.
- Saraswathi KY, Muthal AP, Kandhare A, Rojatkar S, Bodhankar SL. Study of methanolic extract of artemisia pallens wall on endurance of laboratory animals. Pharmacologia. 2014;5:298–309.
- Kandhare AD, Bodhankar SL, Mohan V, Thakurdesai PA. Effect of glycosides based standardized fenugreek seed extract in bleomycin-induced pulmonary fibrosis in rats: Decisive role of Bax, Nrf2, NF-κB, Muc5ac, TNF-α and IL-1β . Chem Biol Interact. 2015;237:151–165.
- Kandhare AD, Ghosh P, Ghule AE, Zambare GN, Bodhankar SL. Protective effect of Phyllanthus amarus by modulation of endogenous biomarkers and DNA damage in acetic acid induced ulcerative colitis: Role of phyllanthin and hypophyllanthin. Apollo Med. 2013;10:87–97.
- Patil A, Guru A, Mukhrjee A, et al. Elucidation of gastro-protective activity of Morin in pylorus ligation induced gastric ulcer via modulation of oxidative stress. Der Pharmacia Lettre. 2015;7:131–139.
- Zhou B, Wu LJ, Li LH, et al. Silibinin protects against isoproterenol-induced rat cardiac myocyte injury through mitochondrial pathway after up-regulation of SIRT1. J Pharmacol Sci. 2006;102:387–395.
- Kandhare AD, Raygude KS, Ghosh P, et al. Effect of hydroalcoholic extract of Hibiscus rosa sinensis Linn. leaves in experimental colitis in rats. Asian Pac J Trop Biomed. 2012;2:337–344.
- Visnagri A, Kandhare AD, Shiva Kumar V, et al. Elucidation of ameliorative effect of Co-enzyme Q10 in streptozotocin-induced diabetic neuropathic perturbation by modulation of electrophysiological, biochemical and behavioral markers. Biomed Aging Pathol. 2012;2:157–172.
- Olaleye MT, Rocha BT. Acetaminophen-induced liver damage in mice: Effects of some medicinal plants on the oxidative defense system. Exp Toxicol Pathol. 2008;59:319–327.
- Honmore V, Kandhare A, Zanwar AA, Rojatkar S, Bodhankar S, Natu A. Artemisia pallens alleviates acetaminophen induced toxicity via modulation of endogenous biomarkers. Pharm Biol. 2015;53:571–581.
- Denicola A, Radi R. Peroxynitrite and drug-dependent toxicity. Toxicology. 2005;208:273–288.
- Kandhare AD, Shivakumar V, Rajmane A, Ghosh P, Bodhankar SL. Evaluation of the neuroprotective effect of chrysin via modulation of endogenous biomarkers in a rat model of spinal cord injury. J Nat Med. 2014;68:586–603.
- Ketkar S, Rathore A, Kandhare AD, et al. Alleviating exercise-induced muscular stress using neat and processed bee pollen: Oxidative markers, mitochondrial enzymes, and myostatin expression in rats. Integr Med Res. 2015;4:147–160.
- Raygude KS, Kandhare AD, Ghosh P, Bodhankar SL. Anticonvulsant effect of fisetin by modulation of endogenous biomarkers. Biomed Prev Nutr. 2012;2:215–222.
- Ghosh J, Das J, Manna P, Sil PC. Acetaminophen induced renal injury via oxidative stress and TNF-alpha production: Therapeutic potential of arjunolic acid. Toxicology. 2010;268:8–18.
- Gardner CR, Laskin JD, Dambach DM, et al. Reduced hepatotoxicity of acetaminophen in mice lacking inducible nitric oxide synthase: Potential role of tumor necrosis factor-alpha and interleukin-10. Toxicol Appl Pharmacol. 2002;184:27–36.
- Knight TR, Kurtz A, Bajt ML, Hinson JA, Jaeschke H. Vascular and hepatocellular peroxynitrite formation during acetaminophen toxicity: Role of mitochondrial oxidant stress. Toxicol Sci. 2001;62:212–220.
- Abdel-Zaher AO, Abdel-Rahman MM, Hafez MM, Omran FM. Role of nitric oxide and reduced glutathione in the protective effects of aminoguanidine, gadolinium chloride and oleanolic acid against acetaminophen-induced hepatic and renal damage. Toxicology. 2007;234:124–134.
- Kandhare AD, Raygude KS, Ghosh P, Ghule AE, Bodhankar SL. Therapeutic role of curcumin in prevention of biochemical and behavioral aberration induced by alcoholic neuropathy in laboratory animals. Neurosci Lett. 2012;511:18–22.
- Kandhare AD, Raygude KS, Shiva Kumar V, et al. Ameliorative effects quercetin against impaired motor nerve function, inflammatory mediators and apoptosis in neonatal streptozotocin-induced diabetic neuropathy in rats. Biomed Aging Pathol. 2012;2:173–186.
- Drotman RB, Lawhorn GT. Serum enzymes as indicators of chemically induced liver damage. Drug Chem Toxicol. 1978;1:163–171.
- Kandhare AD, Kumar VS, Adil M, Rajmane AR, Ghosh P, Bodhankar SL. Investigation of gastro protective activity of Xanthium strumarium L. by modulation of cellular and biochemical marker. Orient Pharm Exp Med. 2012;12:287–299.
- Kim KA, Lee WK, Kim JK, et al. Mechanism of refractory ceramic fiber- and rock wool-induced cytotoxicity in alveolar macrophages. Int Arch Occup Environ Health. 2001;74:9–15.
- Visnagri A, Kandhare AD, Ghosh P, Bodhankar SL. Endothelin receptor blocker bosentan inhibits hypertensive cardiac fibrosis in pressure overload-induced cardiac hypertrophy in rats. Cardiovasc Endocrinol. 2013;2:85–97.
- Kobashigawa JA, Kasiske BL. Hyperlipidemia in solid organ transplantation. Transplantation. 1997;63:331–338.
- Raghavendran HR, Sathivel A, Devaki T. Effect of Sargassum polycystum (Phaeophyceae)-sulphated polysaccharide extract against acetaminophen-induced hyperlipidemia during toxic hepatitis in experimental rats. Mol Cell Biochem. 2005;276:89–96.
- Forman BM, Goode E, Chen J, et al. Identification of a nuclear receptor that is activated by farnesol metabolites. Cell. 1995;81:687–693.
- Fiorucci S, Rizzo G, Donini A, Distrutti E, Santucci L. Targeting farnesoid X receptor for liver and metabolic disorders. Trends Mol Med. 2007;13:298–309.
- Chen WD, Wang YD, Meng Z, Zhang L, Huang W. Nuclear bile acid receptor FXR in the hepatic regeneration. Biochim Biophys Acta. 2011;1812:888–892.
- Wagner M, Zollner G, Trauner M. Nuclear receptors in liver disease. Hepatology. 2011;53:1023–1034.
- Yan J, Gong Y, She YM, Wang G, Roberts MS, Burczynski FJ. Molecular mechanism of recombinant liver fatty acid binding protein’s antioxidant activity. J Lipid Res. 2009;50:2445–2454.
- Raza H, Pongubala JR, Sorof S. Specific high affinity binding of lipoxygenase metabolites of arachidonic acid by liver fatty acid binding protein. Biochem Biophys Res Commun. 1989;161:448–455.
- Ek BA, Cistola DP, Hamilton JA, Kaduce TL, Spector AA. Fatty acid binding proteins reduce 15-lipoxygenase-induced oxygenation of linoleic acid and arachidonic acid. Biochim Biophys Acta. 1997;1346:75–85.
- Wang G, Gong Y, Anderson J, et al. Antioxidative function of L-FABP in L-FABP stably transfected Chang liver cells. Hepatology. 2005;42:871–879.
- Thadhani R, Pascual M, Bonventre JV. Acute renal failure. N Engl J Med. 1996;334:1448–1460.
- Bonventre JV. Dedifferentiation and proliferation of surviving epithelial cells in acute renal failure. J Am Soc Nephrol. 2003;14(Suppl 1):S55–S61.
- Kandhare AD, Patil MV, Bodhankar SL. L-Arginine attenuates the ethylene glycol induced urolithiasis in ininephrectomized hypertensive rats: Role of KIM-1, NGAL, and NOs. Ren Fail. 2015;37:709–721.