Abstract
Objective: The aim of this study was to develop a practical and efficient method for isolation and characterization of the enterobacterial microbiota of infant fecal samples. It could be used for reliable comparisons between individual microflora in different neonates, and in the follow-up of the succession in the floral developments in various nutritional conditions. Methods: A combination of traditional plate culture and different enrichment procedures carried out in a portable microbe enrichment unit (PMEU) was studied. The strains were isolated by plate culture both before and after enrichment. The isolated strains were identified by biochemical tests and the phenotypes of the isolates were compared by the PhenePlate™ technique. To obtain a picture of the formation of intestinal flora during the first year two follow-up samples were collected at the ages of 3 and 12 months from eight Finnish infants (one girl and seven boys). Results: Altogether 21 and 46 enterobacterial isolates were detected in samples taken at the ages of 3 and 12 months, respectively; 7/21 (33%) and 24/46 (52%) isolates were not detected by direct plating but only after enrichment. This material was supposed to demonstrate the differences between the different methodological approaches. Conclusion: There were age-dependent changes in the species proportions of different subpopulations of Enterobacteriaceae detected from the infantile fecal microbiota at different times. The statistically significant difference in the number of isolations with and without enrichment step suggests that the use of enrichment is highly recommendable and the PMEU equipment and method have now proven suitable for that purpose, at least for screening and monitoring the infant fecal flora. The method offers more representative, quicker, and relatively practical tools for obtaining an overall picture of the enterobacterial microbiota of the fecal samples and its variations, thus also giving an idea of the microbial communities in the gastrointestinal tract.
Introduction
The maturation of the immune system of the infantile intestine is a sensitive process that may reflect human health and well-being throughout life. The intestinal microbiota, consisting of over a thousand different bacterial species, is in a continuous interaction with the gut mucosa and has an important role in this process. The host–bacterial relationships in the gut are important factors in the host's health, being involved, for example, in the digestion of foods, production of vitamin K (Citation1), and protection of the intestinal mucosa against pathogenic microbes (Citation2). Recently, a model of balanced function of different enterobacterial strains in the regulation of the intestinal pH has been suggested (Citation3). Some commercial strains have been subjected to intense studies over many decades, such as Lactobacillus rhamnosus strain GG (Gorbach, Goldin), which has been shown to shorten and decrease the severity of acute diarrhea in the pediatric population, reduce diarrheal symptoms during antibiotic treatment, prevent traveler's diarrhea, and prevent and treat allergic reactions (Citation4).
The gram-negative facultatively anaerobic bacilli of the family Enterobacteriaceae form a very important part of the human intestinal microbiota. They have the ability to grow both aerobically and anaerobically. Some of the bacteria belonging to this group, such as Salmonella, Shigella, and also some strains of Escherichia coli and Klebsiella, have important pathogenic properties associated, for example, with their different fimbriae and flagellae (Citation5,Citation6). However, most of the Enterobacteriaceae detected in fecal specimens are harmless in normal circumstances. They constitute an important fraction of the normal microbiota in man (Citation7,Citation8). It has been shown that the normal flora protects the host against harmful or pathogenic organisms (Citation9). It is also possible for them to act in a commensal fashion, producing balanced function regulating the conditions in the intestinal environment so that they are more favorable for the entire microbial community and also for the host (Citation3). This dualistic balance is a consequence of some members of the family, such as E. coli, carrying out mixed acid fermentation where acetic, lactic, and succinic acids occur as the main end products, whereas many other strains, e.g. Klebsiella spp., produce mostly 2,3-butadiene and ethanol in a synchronized fashion with the mixed acid fermentation, which helps to maintain the duodenal pH at around 6.0.
It has been postulated that only 10–50% of the fecal bacteria can be studied by traditional cultivation methods (Citation10). During the last decade several alternative methods have been developed for studying the variation of the intestinal microflora. Bacterial cellular fatty acid profiles reflecting relative changes in the overall composition and diversity of the fecal flora over time have been studied by gas-liquid chromatography (Citation10,Citation11). Also, a wide spectrum of molecular methods have been introduced to analyze fecal microbiota, e.g. fluorescent in situ hybridization (FISH) (Citation12,Citation13), denaturing gel electrophoresis (DGGE) (Citation14,Citation15), and quantitative real-time PCR (Citation16,Citation17). These methods are highly effective in obtaining a rapid survey of microbes. However, since living bacteria cannot be isolated by these approaches, it is not possible to perform any further studies concerning the effects of single bacterial strains or their interactions with the host or with each other.
Enrichment of bacterial samples in liquid culture medium is essential in recovering bacteria that have suffered from environmental stress due to dehydration, high temperature, high or low osmotic pressure, prolonged storage, etc., and in the selection of certain bacteria from heterogeneous samples (Citation18). Enrichment is also highly advantageous when very small sample volumes are studied. For these purposes we studied an effective enrichment procedure in combination with plate culture. It is also possible to generate conditions that simulate the real-life situation in the small intestines, for example (Citation3).
Material and methods
Subjects
Fecal samples were collected from eight infants (nos I–VIII) at the ages of 3 and 12 months. These healthy children (one girl and seven boys) were born in Kuopio University Hospital between February 2004 and February 2005. There was no diagnosis or suspicion of allergy in any of the subjects. One child had received two courses of systemic antibiotic therapy while the others had no antibiotic treatments. Three children had gastroenteritis in anamnesis and two had received probiotic products.
Fecal sampling and storage
The samples were collected in plastic containers at home by parents. The samples were immediately frozen at −20°C and delivered in cool bags to the study personnel for storage at −70°C within 2 weeks.
Studies of the intestinal microbiota
The samples were thawed rapidly in a warm water bath and homogenized manually. Then 0.5 g of each sample was mixed 1:9 with phosphate-buffered saline (PBS, pH 7.4). Tenfold serial dilutions of samples were carried out with PBS (pH 7.4). Then samples were plated to BD CHROMagar™ Orientation plates (Becton, Dickinson & Co., Sparks, MD, USA), 100 μl per plate. Two parallel plates were used for each dilution.
The enrichment culture was carried out in a portable microbe enrichment unit (PMEU; Finnoflag Oy, Samplion Oy, Kuopio and Siilinjärvi, Finland) () (Citation19). This is a portable incubator where the enrichment of microbes takes place in specific syringes containing enrichment broth. The PMEU has been designed to enhance cultivation of microbes under aseptic gas flow in adjusted temperatures. It has improved the recovery of enteric bacteria in different samples, as demonstrated by our studies with Campylobacter spp. (Pitkänen et al.,) and Salmonella spp. (Citation20). In this study the gas flow was funneled into 20 ml syringes (ONCE, CODAN Medical ApS, DK-4970 Rødby, Denmark) through a sterile filter (0.2 μm, SY13TF-S, Advanced Micro devices Pvt Ltd, Ambala Cantt, India) and a needle (0.80 mm × 120 mm, Sterican, B. Braun, Melsungen, Germany). The gas was bubbled through the broth, agitating the broth and the bacterial cells.
Figure 1. A portable microbe enrichment unit (PMEU; Finnoflag Oy and Samplion Oy, Kuopio and Siilinjärvi, Finland) (Citation19).
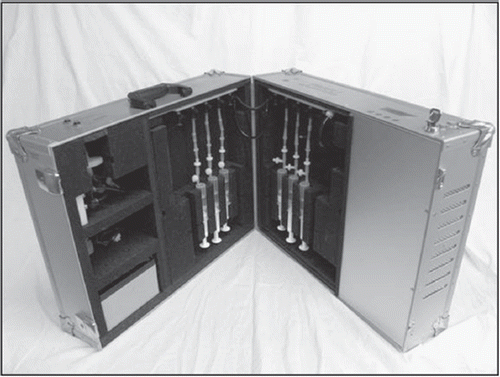
The samples were enriched in MacConkey broth (Becton, Dickinson & Co., Sparks, MD, USA) to elicit the enterobacterial growth. After mixing the homogenized samples with PBS, 2 ml of each sample was mixed with 18 ml of MacConkey broth and then aspirated into 20 ml syringes. The syringes were placed into the PMEU and the samples were enriched at 37°C for 8 h aerobically using sterile-filtered ambient air as an agitating gas.
After enrichment, 0.5 g of each enriched sample was diluted 1:9 with PBS (pH 7.4). Serial dilutions and plating were performed as described above. The plates were studied after 2 days of aerobic cultivation at 37°C. All colonies that were recognized by their typical morphology to belong to the family Enter-obacteriaceae were enumerated. The characteristics of different types of these colonies were documented by size, shape, edge, profile, color, opacity, and any other noteworthy characteristics. If the colony morphology did not clearly indicate the strain's potential relatedness to the Enterobacteriaceae, Gram staining was also done and the gram-negative bacilli were selected for further tests. The confirmed enterobacterial strains were then isolated as pure cultures using BD CHROMagar™ Orientation plates.
The homologies of the isolated strains were tested by the PhenePlate™ RS (Bactus AB, Huddinge, Sweden) phenotypic comparison technique (Citation21,Citation22). The plates were read manually after incubation for 16, 40, and 64 h at 37°C. The results were analyzed by PhPWIN software (PhPlate AB, Stockholm, Sweden). Isolates were regarded as being the same strain when the similarity level was 97.5% or more.
The species level identification of the isolated strains was performed by PhenePlate™ patterns and by BBL Crystal Enteric/Nonfermenter test (Becton, Dickinson & Co.) combined with BBL DrySlide Indole and BBL DrySlide Oxidase tests (Becton, Dickinson & Co.).
Statistics
SPSS 16.0 for Windows software was used in the statistical analysis. The significance of difference at different ages and between direct plating and combination of direct plating and enrichment was evaluated utilizing Wilcoxon's nonparametric test. When direct plating was compared to total counts of isolations or strains one-tailed Wilcoxon's test was used. The differences were considered statistically significant in cases when the p value was ≤ 0.05. The validity of the methods used in statistical analysis has been approved by a certified statistician.
Ethical considerations
This study belongs to the ongoing study ‘The fecal microbiota of infants with genetic risk of type 1 diabetes’, which is a substudy of the Finnish Dietary Intervention Trial for the Prevention of Type 1 Diabetes (FINDIA). The protocol of the study was approved by the Joint Ethical Committee for Human Research at the University of Kuopio and the Kuopio University Hospital. Informed parental consent was obtained from all parents of the subjects before enrolment in the study.
Results and Discussion
To study the variation between the normal flora, 21 and 46 enterobacterial strains were isolated from samples of infants I–VIII taken at the ages of 3 and 12 months, respectively. The isolates are shown in . A significant increase in the number of isolated strains was achieved by using PMEU protocols in the enrichment.
Table I. Enterobacterial isolates from fecal samples taken at the ages of 3 and 12 months from infants I–VIII.
The study of fecal samples is a practical but still indirect method for surveying the intestinal microbiota, and gives only suggestive information on the microflora. Samples taken during endoscopy or otherwise directly from different parts of the intestinal tract are extremely valuable when available. However, it is not acceptable to perform endoscopy on a large group of healthy children. Our approach was to follow up the development of the microbe composition of the fecal samples by enhanced cultivation methods in order to isolate as many enterobacterial strains as possible; these strains probably originated from the lower gastrointestinal tract of the subjects. The total number of enterobacterial strains was 21 and 46 at the ages of 3 and 12 months, respectively. The most commonly isolated strains were Klebsiella oxytoca (6/21), E. coli (4/21), and Enterobacter cloacae (3/21) at the age of 3 months and E. coli (25/46), Klebsiella pneumoniae (5/46), Citrobacter freundii, and Klebsiella oxytoca (both 4/46) at the age of 12 months. The total of E. coli strains was on average higher in samples taken at the age of 12 months than at the age of 3 months (p < 0.05).
At the age of 3 months the enterobacterial strains were found in the samples with a median of 2.5 different strains per sample (range 0–6). At the age of 12 months there was on average more complexity and variation in the enterobacterial flora (p < 0.05), with a median of 6.5 different strains per sample (range 0–9). These results were obtained from the PMEU enriched samples. By direct plating the corresponding median values were 1.5 and 3.0. The results are presented in . At the age of 12 months significantly more strains were isolated by the combination of direct plating and enrichment than by direct plating alone (p = 0.02). The influence of the enrichment on isolation of different enterobacterial species is presented in . At the age of 12 months on average more E. coli strains were isolated by the combination of direct plating and enrichment than by direct plating alone (p = 0.03). The increased complexity at the age of 12 months compared to the samples taken at the age of 3 months may reflect acidic and nutritional changes resulting, for example, from remarkable dietary changes taking place between those time points. However, there were big differences between individuals in the number of strains at the age of 12 months. The reasons for this phenomenon are in the scope of further investigations.
Table II. Enterobacterial isolates detected by direct plating and enrichment in the PMEU in samples from infants I–VIII.
Figure 2. Cumulative presentation of enterobacterial isolates by species detected only by direct plating (DP), both by direct plating and enrichment (ENR; 8 h, +37°C, MacConkey broth in the PMEU), and only after enrichment at the age of (a) 3 months and (b) 12 months. Note that, at the age of 12 months, on average more Escherichia coli strains were isolated by combination of direct plating and enrichment than by direct plating alone (p = 0.03).
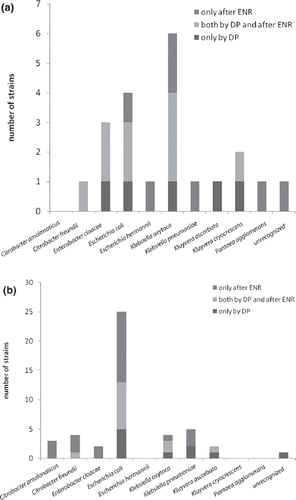
As shown in , which shows the enterobacterial strains isolated from the samples from infants I–VIII, the proportions of isolated strains detected by direct plating varied between different samples and between species. It is noteworthy that although an average of 53% of all strains were detected by direct plating, the proportion was 40% or lower for many species, such as Citrobacter amalonaticus, Citrobacter freundii, Escherichia hermannii, K. pneumoniae, and Pantoea agglomerans ().
The phenotypes of the isolates were compared by the PhenePlate™ technique, which provides an automated system for biochemical fingerprinting, thus offering practical tools for bacterial phenotypic comparison (Citation22). The isolates were regarded as the same strain when the similarity level was 97.5% or more. The PhenePlate™ phenotypic comparison also makes it possible to study the stability of the isolated strains between different time points. Two enterobacterial strains were isolated from both studied samples, i.e. at the ages of 3 and 12 months, of individual infants. PhenePlate™ dendrograms of the enterobacterial isolates from infants I and III indicate one Kluyvera ascorbata strain from infant I and one E. coli strain from infant III that were detected at both time points ().
Figure 3. Dendrograms showing phenotypic homologies of the enterobacterial isolates from infants I (a) and III (b) studied by the PhenePlateTM technique (Bactus AB, Huddinge, Sweden) [21,22]. The figures were processed by PhPWIN software (PhPlate AB, Stockholm, Sweden). Isolates were regarded as the same strain when the similarity level was 97.5% or more (marked by a vertical dotted line). (1) Kluyvera ascorbata strain that was isolated from samples taken at the ages of both 3 and 12 months from infant I. (2) Escherichia coli strain that was isolated from samples taken at the ages of both 3 and 12 months from infant III.
![Figure 3. Dendrograms showing phenotypic homologies of the enterobacterial isolates from infants I (a) and III (b) studied by the PhenePlateTM technique (Bactus AB, Huddinge, Sweden) [21,22]. The figures were processed by PhPWIN software (PhPlate AB, Stockholm, Sweden). Isolates were regarded as the same strain when the similarity level was 97.5% or more (marked by a vertical dotted line). (1) Kluyvera ascorbata strain that was isolated from samples taken at the ages of both 3 and 12 months from infant I. (2) Escherichia coli strain that was isolated from samples taken at the ages of both 3 and 12 months from infant III.](/cms/asset/23b061bd-80b8-42e4-9aea-cf02e3afc214/zmeh_a_436959_f0003_b.gif)
Diet and antibiotic medications have profound effects on the development of the intestinal micro-biota. The type of feeding, introduction of solid foods, weaning, and the use of prebiotics, probiotics, and antibiotics have multiple influences on the composition of the intestinal flora. The effect of the nutrition also needs to be further elucidated.
Biomolecular methods are highly effective in screening large microbial populations but they also have certain limitations. These methods require careful preparation of the samples, they are vulnerable as regards different sources of contamination, and the outcome of the analysis may be highly dependent on the sample matrix (Citation23,Citation24). To overcome these problems and achieve more accuracy and reliability, a short pre-enrichment before the eventual analysis has been proposed (Citation18).
The data produced by genetic methods mainly give an idea as to the hereditary traits of the strains without providing information on the phenotype or the behavior of the microbes in different circumstances. When fecal microbiota is studied as a whole it is also important to isolate strains in order to study the characteristics and physiological properties of the strains, and their interactions. The isolation, storage, and further studies of strains provide possibilities for investigating both different symbiotic or pathogenic host–bacterial interactions and commensal or antagonistic interactions between bacterial strains. It is obvious that combinations of conventional and molecular analysis tools are needed to gain better understanding of the diversity of the human microbiota (Citation25).
The present method was applied for the recovery and growth acceleration of bacterial strains. New procedures for their isolation are needed for understanding of the impact of various strains of the family Enterobacteriaceae on human well-being. Our results indicate that the efficacy and feasibility of the plate cultivation method can be markedly enhanced by prior enrichment in the PMEU, where temperature, gas atmosphere, and nutrient availability are optimized in a practical way, providing optimal culture conditions for the recovery and growth of the studied bacteria. In fact, most of the enterobacterial species were isolated only after the enrichment in the PMEU. These findings are in accordance with earlier studies where the PMEU was shown to improve the recovery of bacteria in environmental samples (Citation26).
Conclusions
In this study a novel approach for the enhancement of cultivation-based methods in the study of fecal microbiota was tested by studying healthy infants. The combination of direct plating and enrichment in the PMEU was proven to give statistically signifi-cant improvement in isolation of the enterobacterial strains when compared with direct plating. This enhanced cultivation method provided higher numbers of different cultivable strains from fecal samples, offering more precise coverage of the actual enterobacterial population in the intestines. It also made the comparisons of various time points and between different individuals more rewarding, thus providing novel tools for investigating the effectiveness of various probiotic treatments in the future.
In this study the enterobacterial microbiota seemed to be fairly simple at the age of 3 months but much more complex at the age of 12 months. Also, major differences were seen between individuals in the numbers of strains at the age of 12 months. The factors causing these differencies and the importance of these findings for human health remain to be elucidated by further studies.
The present method offers practical and feasible tools for investigating the characteristics and changes of the composition of fecal enterobacterial microbiota. Further studies parallel to this study are on the way to evaluate the influence of, for example, age, diet, and antibiotics on the development of the intestinal microbiota during infancy. It is noteworthy that these developments that occur during early childhood may also have a profound influence on the composition of individual normal flora throughout the life. As Ellis-Pegler et al. (Citation27) and Tomkins et al. (Citation28) reported, most changes towards the adult pattern take place between 4 and 12 months. Gradual changes continue even after 2–4 years of age, but by 6 years of age the counts of total anaerobes, enterobacteria, Bacteroides, and Veillonella have reached those of adults in English children formula-fed during infancy.
Acknowledgments
We express our gratitude to the Päivikki and Sakari Sohlberg Foundation, National Graduate School of Clinical Investigation and Foundation for Paediatric Research for financial support of the study. We are grateful to Professor Atte von Wright and Professor Raimo Voutilainen from the University of Kuopio for useful discussions and advice. We are also grateful to our laboratory assistant Tanja Auraniemi for her skillful work.
Declaration of interest: The study has been supported by National Graduate School of Clinical Investigation and Foundation for Paediatric Research from Finland by grants to J.P. and by Päivikki and Sakari Sohlberg Foundation by grants to O.V. - E.H. is the inventor of the PMEU and the founder of Finnoflag Oy. He has been involved in multiple scientific projects applying PMEU e.g. at medical, environmental and industrial fields. The authors alone are responsible for the content and writing of the paper.
References
- Ramotar K, Conly JM, Chubb H, Louie TJ. Production of menaquinones by intestinal anaerobes. J Infect Dis 1984;150:213–18.
- Hooper LV, Gordon JI. Commensal host-bacterial relationships in the gut. Science 2001;292:1115–18.
- Hakalehto E, Humppi T, Paakkanen H. Dualistic acidic and neutral glucose fermentation balance in small intestine: simulation in vitro. Pathophysiology 2008;15:211–20.
- Goldin BR, Gorbach SL. Clinical indications for probiotics: an overview. Clin Infect Dis 2008;46(Suppl 2): S96–100.
- Hakalehto E, Santa H, Vepsäläinen J, Laatikainen R, Finne J. Identification of a common structural motif in the disordered N-terminal region of bacterial flagellins. Evidence for a new class of fibril-forming peptides. Eur J Biochem 1997;250:19–29.
- Hakalehto E. 2000. Characterization of Pectinatus cerevisiiphilus and P. frisingiensis surface components. Use of synthetic peptides in the detection of some Gram-negative bacteria. PhD Thesis. Kuopio, Finland: Kuopio University Publications C, Natural and Environmental Sciences 112.
- Stark PL, Lee A. The microbial ecology of the large bowel of breast-fed and formula-fed infants during the first year of life. J Med Microbiol 1982;15:189–203.
- Kühn I, Tullus K, Möllby R. Colonization and persistence of Escherichia coli phenotypes in the intestines of children aged 0 to 18 months. Infection 1986;14:7–12.
- Majamaa H, Isolauri E, Saxelin M, Vesikari T. Lactic acid bacteria in the treatment of acute rotavirus gastroenteritis. J Pediatr Gastroenterol Nutr 1995;20:333–8.
- Tapiainen T, Ylitalo S, Eerola E, Uhari M. Dynamics of gut colonization and source of intestinal flora in healthy newborn infants. APMIS 2006;114:812–17.
- Ahtonen P, Lehtonen OP, Kero P, Eerola E. Faecal fatty acids and gastrointestinal upset in newborn infants. Lett Appl Microbiol 1997;24:91–4.
- Harmsen HJM, Gibson GR, Elfferich P, Raangs GC, Wildeboer-Veloo ACM, Argaiz A, . Comparison of viable cell counts and fluorescence in situ hybridization using specific rRNA-based probes for the quantification of human fecal bacteria. FEMS Microbiol Lett 2000;183:125–9.
- Ouwehand AC, Salminen S, Arvola T, Ruuska T, Isolauri E. Microbiota composition of the intestinal mucosa: association with fecal microbiota? Microbiol Immunol 2004;48:497–500.
- Bernbom N, Nørrung B, Saadbye P, Mølbak L, Vogensen FK, Licht TR. Comparison of methods and animal models commonly used for investigation of fecal microbiota: effects of time, host and gender. J Microbiol Methods 2006;66:87–95.
- Millar MR, Linton CJ, Cade A, Glancy D, Hall M, Jalal H. Application of 16S rRNA gene PCR to study bowel flora of preterm infants with and without necrotizing enterocolitis. J Clin Microbiol 1996;34:2506–10.
- Conte MP, Schippa S, Zamboni I, Penta M, Chiarini F, Seganti L, . Gut-associated bacterial microbiota in paediatric patients with inflammatory bowel disease. Gut 2006;55:1760–7.
- Malinen E, Rinttilä T, Kajander K, Mättö J, Kassinen A, Krogius L, . Analysis of the fecal microbiota of irritable bowel syndrome patients and healthy controls with real-time PCR. Am J Gastroenterol 2005;100:373–82.
- Hakalehto E. Semmelweis’ present day follow-up: updating bacterial sampling and enrichment in clinical hygiene. Pathophysiology 2006;13:257–67.
- Hakalehto E. Menetelmä ja laite mikrobiologisten näytteiden rikastamista ja tutkimista varten. [Method for detecting microbes from an enrichment culture.] Finnish Patent No. 106561. 2001.
- Hakalehto E, Pesola J, Heitto L, Närvänen A, Heitto A. Aerobic and anaerobic growth modes and expression of type 1 fimbriae in Salmonella. Pathophysiology 2007;14:61–9.
- Kühn I. Biochemical fingerprinting of Escherichia coli: a simple system method for epidemiological investigations. J Microbiol Methods 1985;3:159–70.
- Kühn I, Ayling-Smith B, Tullus K, Burman LG. The use of colonization rate and epidemic index as tools to illustrate the epidemiology of faecal Enterobacteriaceae strains in Swedish neonatal wards. J Hosp Infect 1993;23:287–97.
- da Mota FF, Gomes EA, Paiva E, Seldin L. Assessment of the diversity of Paenibacillus species in environmental samples by a novel rpoB-based PCR-DGGE method. FEMS Microbiol Ecol 2005;53:317–28.
- González Á, Guillamón JM, Mas A, Poblet M. Application of molecular methods for routine identification of acetic acid bacteria. Int J Food Microbiol 2006;108:141–6.
- Mai V, Morris JG. Colonic bacterial flora: changing understandings in the molecular age. J Nutr 2003;134:459–64.
- Heitto L, Heitto A, Hakalehto E. 2006. Tracing wastewaters with faecal coliforms and enterococci. Simola H. Seminar on large lakes 2006, University of Joensuu. Publications of the Karelian Institute, 145:101–6 (in Finnish with English abstract).
- Ellis-Pegler RB, Crabtree C, Lambert HP. The faecal flora of children in the United Kingdom. J Hyg (Lond) 1975;75:135–42.
- Tomkins AM, Bradley AK, Oswald S, Drasar BS. Diet and the faecal microflora of infants, children and adults in rural Nigeria and urban U. K. J Hyg (Lond) 1981;86:285–93.
- Pitkänen T, Bräcker J, MIettinen IT, Heitto A, Pesola J, Hakalehto E. Enhanced enrichment and detection of thermotolerant Campylobacter species from water using the Portable Microbe Enrichment Unit and real-time PCR. Can J Microbiol 2009;55:849–58.