Abstract
Background: Unpasteurized fermented milk is used for the production of traditional dairy products in certain rural areas of Greece. Methods: In an attempt to search for candidate beneficial probiotic strains of Enterococcus faecium and Enterococcus faecalis, isolates recovered from traditional dairy products and from faeces of infants fed with such products were evaluated regarding their antimicrobial activity and their standard biochemical profile. Results: Almost all tested strains showed resistance to a pH range from 3.0 to 6.5, tolerance to bile acid within 24 h and resistance to phenol. Field isolates of E. faecalis and E. faecium presented higher antimicrobial activity against classic food pathogenic serovars of Salmonella enteritica (S. typhi, S. typhimurium, S. ancona, S. enteritidis) compared with reference strains. Conclusion: The implementation of beneficial enterococcal strains in the production of fermented dairy products is part of technological process in constant development aimed at obtaining food products of increased quality and safety.
Key words::
Introduction
During the last decade, much of the research efforts have aimed at improving food quality and safety, by focusing on the new production technology of fermented and functional foods. The use of microflora to reduce the occurrence of intestinal pathogenic bacteria in food products has been applied as a pro-biotic strategy (Citation1). The goal of this technique is to introduce a beneficial microflora prevailing in all intestinal microbial niches and thereby preventing the establishment of pathogens.
Administration of antibiotics alters the intestinal microflora in humans rendering them more sensitive to infections. In livestock, a similar condition was observed under intensive rearing with use of antibiotics as feed additives (Citation2–4). Intestinal enterococcal isolates have been shown to be particularly resistant to antimicrobial agents (Citation5–7). This fact necessitates their rapid and accurate identification when implicated in foods.
As there is a permanent need to select new beneficial strains with improved capacities in fermentation processes, our interest is focused on some Enterococcus strains that are mainly linked to dairy products (Citation8). Moreover, similar Enterococcus strains isolated from the intestine of infants are of special interest, being part of the human intestinal microflora and also known to be inoffensive. The reason for combining these two sources of enterococcal isolates in the present study originates from the need to acquire knowledge on the possibility and capacity of food-borne enterococcal strains to colonize the infant intestine.
The rural population in the region of Epirus (NW Greece) traditionally manufactures dairy products (‘feta’ cheese, goat cheese) from unpasteurized milk. These products, which are considered to be of high nutritional quality and value, are fed to infants.
In our study, Enterococcus faecium and E. faecalis isolates recovered from such dairy products as well as from faeces of infants fed with the above traditional dairy foods were screened and evaluated according to their antimicrobial activity against some Salmonella enteritica serovars known for their pathogenicity in humans. Our goal was to investigate the capacity of these enterococcal strains to colonize the infant's intestine while exhibiting probiotic activity.
Material and methods
Samples
Specimens of unpasteurized milk from ewes, goats and cows, as well as rennet ‘pitia’, were taken from the farms before the fermentation process began. Cheese samples were also collected from the end products (home-made feta cheese and home-made goat cheese) from the same farms. Faeces were obtained from healthy infants aged 1–3 years living in households consuming these products.
Reference strains of Salmonella serovars and of E. faecium and E. faecalis
Food-borne pathogens used for testing antibacterial activity were the serovars Typhi, Typhimurium, Ancona and Enteritidis of S. enterica. These reference strains were isolated from faecal flora of infants suffering from gastroenteritis and were obtained from the National Reference Laboratory for Salmonella in Greece. Serotyping of these strains was performed in the above Reference Laboratory of the National School for Public Health, Athens, Greece. The results were as follows: S. typhi (9,12, Vi:d:-), S. typhimurium (4,5:i:1,2), S. ancona (4,5:A,9.5:-) and S. enteritidis (9:g,m:-).
Strains were isolated and reactivated on Nutrient Agar (NA; Oxoid, Basingstoke, UK) before use. E. faecium and E. faecalis strains were kept in NA supplemented with glycerol (70:30) at –20°C and were subcultured three times in Brain Heart Infusion Broth (BHIB; Oxoid) for activation before experimental use.
Reference strains of E. faecium ACA-DC0225 and E. faecalis ACA-DC0240 were obtained from the Milk Laboratory of the Agricultural University in Athens, Greece.
Isolation and identification of field isolates of E. faecium and E. faecalis
E. faecium and E. faecalis were isolated from traditional dairy products as well as from infant intestinal flora, during the period 2001–2004 ().
Table I. Origin of E. faecium and E. faecalis strains tested in the study.
Preliminary rapid identification of Enterococcus isolates was performed by determination of the acid production and stormy fermentation together with determination of production of H2S and indole and motility of the isolates. Litmus milk medium (Fluka Biochemica, WGK, Germany) supplemented with methylene blue (0.1%) was used (Citation9). Ten ml of Litmus milk medium containing an inverted Durham tube was inoculated with an activated culture of the E. faecium and E. faecalis isolates. Incubation was carried out at 37°C and 45°C for 24 h. pH was determined by means of a pH meter (WTW pHmeter, Weilheim, Germany).
The isolates of E. faecium and E. faecalis were tested for their ability to produce H2S, for their motility and for production of indole in 10 ml of the liquid medium SIM – Sulfur reduction, Indole production and Motility (Becton Dickinson, USA), a differential medium that tests three different para meters, which are represented by the three letters in the name. Incubation was carried out at 37°C for 1 week (Citation10). Observation of any change was noted daily.
The identification of both Enterococcus spp. was performed with the conventional methods based on the Facklam scheme (Citation11,Citation12). The isolates were further characterized by the sugar fermentation pattern, which was determined using the API 20 STREP microsystem, as specified by the manufacturer (BioMerieux, Marcy-l’Etoile, France).
VITEK2 (BioMerieux), an automated system for identification, was also applied. It detects bacterial growth and metabolic reactions in microwells of plastic test cards by measuring fluorescence. Freshly subcultured inocula were prepared by adjusting to a McFarland standard of 0.5 in a 0.45% sodium chloride solution. The ID-GPC card of the Vitek 2 system served for identification (Citation13). A total of 88 field isolates of E. faecium and 67 isolates of E. faecalis were recovered from 120 samples. Based on pH, phenol and lysozyme criteria, 25 strains were eventually investigated for their antibiotic susceptibility and antibacterial action against the pathogens.
Survival at low pH
Survival measurement at low pH was performed following the method proposed by Conway et al. (Citation14). Viable cells were enumerated at 0, 0.5 and 2 h on de Man, Rogosa, Sharp (MRS) agar (Fluka, Buchs, Switzerland) plates incubated aerobically at 37°C for 3 days.
pH and bile resistance
MRS broth containing 200 mM KCl/HCl and 100 mM citric acid/200 mM Na2HPO4, buffered at pH 1.0–2.0 and pH 3.0–6.5, respectively, was used for estimating the bacterial growth under the different pH conditions. Growth was measured by monitoring viable cell counts at 37°C at 0 h and after incubation for 24 h.
The bile tolerance was evaluated as follows. Cultures were inoculated (1%) into MRS broth without and with Oxgall 0.15 and 0.5% (Ox-Bile LP0055; Oxoid) added and incubated at 37°C. Viable cell numbers were estimated at 37°C for 0 and 24 h of incubation on MRS agar plates (Citation8).
Resistance to phenol
Ability to grow in the presence of phenol was estimated by inoculating (2%) cultures in MRS broth (10 ml) with and without 0.4% phenol (Sigma, St Louis, MO, USA) (Citation15). Growth was estimated at 0 h and after incubation for 24 h at 37°C on MRS agar plates.
Resistance to lysozyme
To test for lysozyme susceptibility, MRS broth (10 ml) without and with lysozyme (100 ppm; Sigma) (Citation16–18) was inoculated 1:50 with strains of E. faecium and E. faecalis and incubated at 37°C for 24 h. Growth was measured at 0 h and 24 h on MRS agar plates.
Antibiotic susceptibility assay
Antimicrobial susceptibility was determined by Kirby Bauer disk diffusion and breakpoint method according to the standards of the National Committee for Clinical Laboratory Standards (Citation19–21). Seven antibiotic discs were selected to determine the antibiotic susceptibility of the field isolates of E. faecium and E. faecalis. Antibiotic discs (Oxoid) used were the following: vancomycin (30 μg), teicoplanin (30 μg), gentamicin for high level aminoglycoside resistance (HLAR) (125 μg), ampicillin (10 μg), erythromycin (15 μg), tetracycline (30 μg) and ciprofloxacin (5 μg). For quality control, Staphylococcus aureus ATCC 25923 was used with isolates considered resistant when inhibition zone diameters were below 16 mm for ampicillin, below 14 mm for vancomycin and tetracycline, below 10 mm for teicoplanin, equal to 6 mm for gentamicin (HLAR) and below 15 mm for erythromycin and ciprofloxacin.
In addition to the agar disc diffusion method, another reference method was also used to confirm the presence of HLAR and vancomycin resistance in enterococci.
Minimum inhibitory concentrations of the seven selected antibiotics were determined by agar dilution as described by the Clinical and Laboratory Standards Institute (CLSI) (Citation21). The following bacteria were used for quality control: E. faecium ATCC 6569, E. faecalis ATCC 29212 and Staphylococcus aureus ATCC 29213.
Antimicrobial activity assay for the field isolates of E. faecium and E. faecalis
Antibacterial activities were assayed against S. typhi, S. typhimurium, S. ancona and S. enteritidis using the agar disc diffusion technique modified by Fleming et al. and Schillinger and Lucke (Citation22,Citation23).
Preparation of the Salmonella inocula
The Salmonella enterica serovars were incubated in Triple Soy Broth (TSB; Oxoid) at 37°C for 24 h and were subcultured three times in TSB broth for activation before experimental use. An inoculum of approximately 105–107 cfu/ml of the salmonella serovars was used for susceptibility assays.
To determine the antimicrobial activity, 14 auto-chthonous isolates of E. faecium and 11 isolates of E. faecalis were selected as well as reference strains and were inoculated in MRS broth and incubated at 37°C and 45°C for 3, 6, 24 and 48 h accordingly. Colony counting, determination of pH, optical density (OD), and capacity of lactic acid production were performed for each field isolate and reference strain of enterococci at 37°C or 45°C for 3, 6, 24 and 48 h.
Surface spreading of each salmonella serovar was investigated on Mueller-Hinton agar. Wells of 5 mm diameter and 2 mm depth were made in the agar. Then 50 μl of a culture from the enterococcal isolates were used to fill the wells. Incubation was performed at 37°C and 45°C for 24 h before the diameter of the clear zone surrounding each disc was measured. A clear zone larger than 1 mm was scored as positive. Each test was performed in triplicate.
Bacteriocin procedures
The cells of each culture were pelleted by centrifugation (5000 g for 20 min at 4°C) to obtain a supernatant rich in bacteriocins (Citation24,Citation25). The supernatant was adjusted to pH 6.2 by the use of 5 M NaOH followed by treatment with catalase (1 mg/ml) (Sigma) at 18°C for 30 min and filtration through 0.22 μm pore size filter (Citation22,Citation23,Citation26). Then 50 μl of the filtered supernatant of the enterococcal isolates were used to fill the wells made in Mueller-Hinton agar spread with the salmonella serovars as described above. Overnight incubation of cultures was performed at 37°C and 45°C for 24 h before the diameters of the inhibition zones were measured. As a control of non-bacteriocin inhibition of growth, all cultures were tested for production of lactic acid (DL) before introduction into wells following the same procedure as described above. All of the experiments were performed in triplicate and the results are reported as mean values.
Results
Almost all tested isolates of E. faecium (13 of 15) and E. faecalis (12 of 12) showed a tolerance to a pH range from 3.0 to 6.5. At pH 2.0, 4 of the 15 E. faecium isolates were inhibited, in contrast to only 2 of 12 E. faecalis isolates. Finally, at pH 1.0 all isolates were inhibited in their growth.
The ability of all Enterococcus strains to grow in the presence of phenol (0.4%) at 37°C for 24 h was observed. Resistance to bile acid (0.15% and 0.5% ox gall) was observed among all tested isolates exposed for 24 h. Similarly, resistance to lysozyme was found in all isolates tested at a concentration of 100 ppm of lysozyme.
A multiresistant antibiotic profile was observed according to the criteria set by the CLSI ().
Table II. Antibiotic susceptibility* profiles of E. faecium and E. faecalis isolates from traditional dairy products and infant faeces.
The antimicrobial effect of our Enterococcus isolates against S. typhi, S. typhimurium, S. ancona and S. enteritidis at 37°C and 45°C is reported in . Inhibition zones ranged from 6 to 17 mm in most cases. Inhibition zones > 17 mm were observed for five E. faecium isolates against S. typhi, for eight E. faecium isolates against S. typhimurium and for five E. faecalis isolates against S. typhi and one E. faecalis isolate against S. typhimurium (). We must note here the fact that all of the E. faecium and the majority E. faecalis isolates showing important antimicrobial effects against both Salmonella serovars came from rennet pitia samples, while only one E. faecalis strain was isolated from unpasteurized ewe milk and another one E. faecalis isolate derived from home-made feta cheese. Finally, the reference strains of E. faecium and E. faecalis showed inhibition zones ranging from 5 to 11 mm ().
Table III. Antimicrobial activities (mm of inhibition zone) of selected Enterococcus strains toward pathogenic indicator bacteria at 37°C or 45°C for 24 h cultures/supernatant liquid/l actic acid.
The mean inhibition zones resulting from exposure to cultures, supernatant and lactic acid produced by E. faecium and E. faecalis at 37°C and 45°C against Salmonella serovars are presented in . Among the four serovars, S. typhimurium and S. typhi showed large inhibition zones mainly after exposure to culture and lactic acid produced by the enterococcal isolates. All analyses of variance in the test results were statistically significant (p < 0.05), exhibiting differences in inhibition among the pathogens.
Figure 1. Inhibition zones (mm) of cultures, supernatant liquid and lactic acid of E. faecium and E. faecalis against four pathogenic bacteria (S. ancona, S. enteritidis, S. typhimurium, S. typhi). **Analysis of variance test results (p < 0.05).
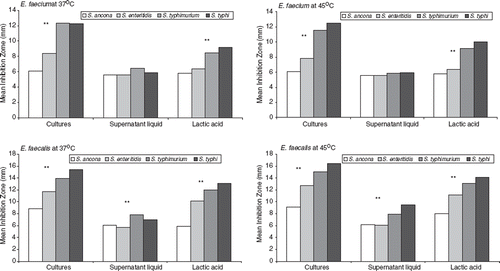
Pearson correlation coefficients among the acidity (%), pH and OD of cultures and supernatant were also estimated. The results were statistically significant (p < 0.05) and showed positive correlations for the OD (r = 0.48 to 0.88) and acidity (r = 0.47 to 0.62) and negative correlations for pH (r = −0.49 to −0.90).
Discussion
The digestive tract of newborn infants is initially sterile, but is rapidly colonized by a characteristic gastrointestinal flora, usually of maternal or environmental origin (Citation27–29). When the microbiota of the newborn becomes stabilized, the newborn is more resistant to infections by exogenous bacteria and this effect is described as colonization resistance (Citation30,Citation31).
Bacteria of the genus Enterococcus, such as E. faecium and E. faecalis, often occur as part of the human and animal intestinal microbiota (Citation32,Citation33). They are also found in large numbers on vegetables, plants, soil, surface waters and in dairy products (Citation8). Despite their frequency in the environment, their accurate identification has always been difficult, as the identification was mainly based on phenotypic traits, which could be highly heterogeneous depending on the isolated strain (Citation8,Citation34,Citation35).
Enterococcus spp. are introduced into a variety of dairy products, including cheese, milk and starter cultures, as they have a primary role in the maintenance and development of specific sensory characteristics in these products during ripening (Citation8,Citation34). Among Enterococcus spp. found in dairy products, E. faecium and E. faecalis have been shown to be more significant (Citation8,Citation34). Their importance to the dairy industry is strengthened by their ability to survive in extreme conditions with respect to pH, temperature and salinity.
In the present study and in agreement with other researchers (Citation14,Citation16) E. faecium was found to exhibit high acid resistance at pH 3.0. Other authors found these species to differ greatly regarding their ability to produce acid, reporting E. faecalis to be a stronger acidifier than E. faecium. However, when pH drops to 1.0, E. faecium and E. faecalis strains were found to be susceptible and non-viable counts were present. This probably also explains their low survival in faeces of breast-fed newborns, as maternal milk causes elimination of an acid stool (Citation27). Enterococcus isolates were inhibited at pH 1.00. This condition is produced when bacteria are transiently passing through the stomach, as human gastric fluid has a pH of 1.2. Many microorganisms found in the stomach represent a transient load of bacteria (Citation36). Acid-resistant bacteria seem to be most frequently found in the stomach (Citation37). Acid-resistant Enterococcus spp. seem to be absent in the human stomach. However, Helicobacter sp. and other bacteria seem to thrive in the mucosa (Citation38). In addition, most bacteria pass through the stomach without being killed during and after the meal (Citation38).
The survival of some bacterial strains in extreme pH conditions may be associated with their resistant cell wall (Citation17). To investigate this parameter, our isolates were exposed to lysozyme concentrations of 100 ppm, as this concentration induces a lytic activity to the bacterial cell mainly by hydrolysis (Citation18). All strains exhibited resistance to lysozyme. Similarly, high resistance to lysozyme in lactic acid-producing bacteria has been observed previously (Citation16–18).
Resistance to bile acid (0.15% and 0.5%) was found among all tested isolates exposed for 24 h. It is of interest to mention that the E. faecium reference strain showed a restraint resistance. Similarly, resistance to 0.3% bile acid was observed by other authors (Citation17,Citation39).
Dissociation and inactivation of some bile components by bacteria, via β-glucuronidase activity (Citation40–43), could explain the inefficiency of the bile upon these bacteria and the observed resistance profile of the Enterococcus isolates in the present study.
In the presence of 0.4% phenol, almost all isolates of E. faecium and E. faecalis were found to grow after incubation for 24 h, showing resistance to phenol. High resistance of some enterococci strains has been shown (Citation17), while a bacteriostatic effect has been observed for several other strains as well (Citation16).
Finally, E. faecium and E. faecalis strains were exposed to pathogenic S. typhi, S. typhimurium, S. ancona and S. enteritidis ().
Limited E. faecium strains showed a pronounced inhibition (> 17 mm clear zone) to S. typhi (n = 5) and S. typhimurium (n = 8). Pronounced inhibition was also effective for five E. faecalis isolates against S. typhi and one E. faecalis isolate against S. typhimurium. As reported already, all E. faecium and the majority of E. faecalis isolates that came from rennet pitia demonstrated an important antimicrobial effect against both S. typhi and S. typhimurium. Only one E. faecalis isolate originating from unpasteurized ewe milk as well as one other from home-made feta cheese exhibited a similar pronounced antimicrobial profile.
The antimicrobial pattern for all other strains against the pathogenic Salmonella serovars was intermediate (11–16 mm) with the exception of the reference strains, which demonstrated a minimal inhibition (6–10 mm) against S. ancona and S. enteritidis. Strains showing a clear zone ≥ 17 mm are considered to demonstrate a pronounced inhibition pattern, those with a clear zone ranging between 11 and 16 mm are compatible with an intermediate inhibition pattern and strains with a clear zone ranging from 6 to 10 mm show a minimal inhibition profile.
Conclusively, no difference in inhibition patterns was observed between the cultures and the supernatant liquids and thus the inhibition effect present cannot be associated with the presence of bacteriocins, but other factors should be considered such as the low pH, the presence of organic acids and the bacterial profile itself.
Moreover, as our Enterococcus strains were cultured in agar plates containing glucose, inhibition must be related to the lactic acid production (Citation16). It is interesting to note that the nature of the growth medium confers stronger antimicrobial properties on Enterococcus (Citation16), as an elevated concentration of glucose in media facilitates the production of cell protective metabolites. The ability of our enterococcal strains, E. faecium and E. faecalis, to inhibit the growth of pathogenic Salmonella varied widely. It is important to note that this characteristic is used as a criterion for the selection of potentially probiotic bacteria for in vivo trials.
The determination of well-defined breakpoints for bacterial species involved in food could be of clinical and biological importance and may even be helpful in strain identification (Citation44–46). CLSI (formerly NCCLS) considered clinical Enterococcus strains with an MIC ≤ 2 μg/ml as susceptible, those with an MIC of 4 μg/ml as intermediate and those with an MIC ≥ 8 μg/ml as resistant to most fluoroquinolones, oxazolidinones and erythromycin (Citation21,Citation47).
In the present study, we have defined the micro-biological breakpoints as the MICs for a given antibiotic and a given species. The aim of determining new microbiological breakpoints is obvious in the case of foods, where international clinical breakpoints are not applied. In the present study the proposed breakpoints for MIC (μg/ml) are: ampicillin (> 4), vancomycin (−), teicoplanin (> 2), tetracycline (> 4), gentamicin-HLAR (–), erythromycin (–) and ciprofloxacin (≥ 2).
The antibiotic patterns of our Enterococcus isolates are shown in . High prevalence of resistant strains was observed through our investigation and our isolates exhibited a multiresistant pattern against most of the studied antibiotics.
Enterococcus from fermented products may act as a potential reservoir of antimicrobial resistance genes that could be transferred either in food or in the intestinal tract (Citation48). This horizontal gene transfer contributes to bacterial adaptation and survival in the environment (Citation49). Substantiating the antimicrobial activities of Enterococcus will affirm their use in food fermentation as probiotic nutrients for the improvement of human health.
Despite the fact that much research concerns the antimicrobial pattern of probiotic strains [Citation22,Citation23,Citation38,Citation39,Citation50,Citation51], officially defined breakpoints to the different antimicrobial agents have not yet been established. It is necessary to perform extended studies concerning the antimicrobial pattern of Enterococcus from different sources.
Multiresistant E. faecium and E. faecalis strains were common in Greek traditional dairy products. Multiresistant E. faecium and E. faecalis strains were also found in the faecal flora of young infants. This is not surprising, as young infants aged from 1 to 3 years are frequently fed, if not daily, with dairy products. Moreover, in rural areas, people used to feed their infants with traditionally prepared foods. Finally, the mean of inhibition for each pathogenic Salmonella in cultures, lactic acid and supernatant liquids of E. faecium and E. faecalis at 37°C and 45°C exhibited profound and statistically significant differences in all parameters at all temperatures. Therefore it is assumed that neither supernatant liquid (bacteriocins) nor lactic acid were involved in the antibacterial action of the strains, but the specific characteristics of the bacterial cells as bacterial counts in cultures were shown to be higher in all cases.
We must report herein that a large number of cells are necessary to obtain bacteriocin concentrations sufficient for effective inhibition of the bacteriocin-sensitive organisms (Citation24). Despite the fact that our study was carried out in vitro, this does not reflect the Enterococcus intestinal implantation as a whole, since a myriad of other factors together with the host itself intervene in the intestinal balance (Citation28,Citation52).
The exhibited antimicrobial activity of E. faecium and E. faecalis supports their successful choice in the development of new fermented foods beneficial to human health. Moreover, the knowledge of the antibiotic resistance of our strains could limit the dissemination of resistance into dairy products and into consumers. It is also known that resistant strains could provide resistance genes to other bacteria, especially when antibiotic resistance is linked to plasmids (Citation17). The search for the presence of the vanA gene by a PCR method could be suggested as a molecular screening marker for vancomycin-resistant Enterococcus (Citation8) before their application in food processing as well as for epidemiological tools. Pheromone-responsible plasmids, conjugative and non-conjugative plasmids and transposons comprise the main gene transfer mechanisms for Enterococcus (Citation53,Citation54). However, their transfer rate was significantly lower when compared with clinical Enterococcus strains (Citation55). Overuse of antibiotics, for example as growth promoters in animals, might be the principal factor with regard to bacterial resistance to antimicrobial drugs. From raw milk, bacteria may be transferred into raw dairy products and then to human intestine. The emergence of resistant enterococcal strains may increase a potential risk related to their use as probiotics in food as well as to their capacity to become opportunistic pathogens in humans. In the latter case serious therapeutic problems may arise.
It is then conceivable that a strict policy must be applied to the entire food and related products chain from production, through to processing and to sale/ retail or export. Food covers primary and processed products for human or animal consumption or use, while related products includes by-products of the food process including hides, skins, wool and the like in terms of safety and assurances.
Specifically, all distinct areas should be covered by this policy including production issues such as, agricultural compounds, veterinary medicines, residues, contamination; processing issues to the extent that they impact on food and related product safety and trade (audit, testing, sampling, animal welfare); assurance, food safety and consumer safety issues; and any other safety issues regarding animal health and welfare.
Systematic monitoring and surveillance of the microbiological quality of selected ecosystems by implementation of a public education campaign must be done, to preserve food quality, optimize sewage treatment and safeguard public health.
Efforts should be made to examine a large number of strains from different origins using the same methodology to clearly define such breakpoints (Citation56). Nevertheless, through our study we have obtained promising results concerning the potential use of beneficial Enterococcus strains in fermented foods, which could contribute considerably in improving the intestinal microbial ecosystem.
Declaration of interest: The authors report no conflicts of interest. The authors alone are responsible for the content and writing of the paper.
References
- Fuller R. Probiotics in man and animals. J Appl Bacteriol. 1989;66:365–78.
- Sutter VL, Finegold SM. The effect of antimicrobial agents on human faecal flora. Skinner FA, Carr JG. The normal microbial flora of man. London: Academic Press. 1974.
- Falkow S. Antibiotics in animal feeds. N Engl J Med. 1970; 282:693–4.
- Bezirtzoglou E, Norin E, Midtvedt T. Influence of roxithromycin on the fecal tryptic activity. Microecol Ther. 1995; 23:102–6.
- Kacmaz B, Aksoy A. Antimicrobial resistance of enterococci in Turkey. Int J Antimicrob Agents. 2005;25:535–8.
- Jung WK, Lim JY, Kwon NH, Kim JM, Hong SK, Koo HC, . Vancomycin-resistant enterococci from animal sources in Korea. Int J Food Microbiol. 2006;113:102–7.
- Temmerman R, Pot B, Huys G, Swings J. Identification and antibiotic susceptibility of bacterial isolates from probiotic products. Int J Food Microbiol. 2003;81:1–10.
- Giraffa G. Functionality of enterococci in dairy products. Int J Food Microbiol. 2003;88:215–22.
- Schierl EA, Blazevic DJ. Rapid identification of enterococci by reduction of Litmus milk. J Clin Microbiol. 1981;14:227–8.
- Lee BH, Simard RE. Evaluation of methods for detecting the production of H2S, volatile sulphides and greening by lacto-bacilli. J Food Sci. 1984;49:981–3.
- Facklam RR. Streptococci and aerococci. Lennette EH, Balows A, Hausler WJ Jr, Truant JP. .Manual of clinical microbiology, 3rd. Washington, DC: ASM. 1980.
- Facklam RR. Comparison of several laboratory media for presumptive identification of enterococci and group D streptococci. Appl Microbiol. 1973;26:138–45.
- Eisner A, Gorkiewicz G, Feierl G, Leitner E, Kofer J, Kessler HH, . Identification of glycopeptide-resistant enterococci by VITEK 2 system and conventional and real-time polymerase chain reaction. Diagn Microbiol Infect Dis. 2005;53:17–21.
- Conway PL, Gorbach SL, Goldin BR. Survival of lactic acid bacteria in the human stomach and adhesion to intestinal cells. J Dairy Sci. 1987;70:1–12.
- Teply M. Ciste mlekarske kultury. Phara. SNTL Nakladatelstvi. Technicke Litertury. Starters for fermented milks. IDF Bulletin (Kurmann JA). 1984;227:41–55.
- Suscovic J, Brkic B, Matosic S, Maric V. Lactobacillus acidophilus M 92 as potential probiotic strain. Milchwissen-schaft. 1997;52:430–5.
- Litopoulou-Tzanetaki E, Xanthopoulos V, Tzanetakis N. Detection of pathogenic and spoilage microorganisms in foods. Food Microbiol. 1998;15:43–50.
- Bottazzi V, Battistitti B, Bosi F, Corradini C, Dellaqua E. Effet du lysozyme sur les ferments lactiques thermophiles. XX International Dairy Congress, Paris. 1978;3:535–6.
- Bauer AW, Kirby WMM, Sheris JC, Tuck M. Antibiotic susceptibility testing by a standardized single disk method. Am J Clin Pathol. 1996;45:493–6.
- NCCLS. Methods for antimicrobial susceptibility testing of anaerobic bacteria, 4th. Approved standard. NCCLS publication no. M11-A4. Wayne, PA: National Committee for Clinical Laboratory Standards. 1997.
- Clinical and Laboratory Standards Institute. Performance standards for antimicrobial susceptibility testing; 16th informational supplement. CLSI, Document M100-S16. 2006.
- Fleming HP, Etchells JL, Costillow RL. Microbial inhibition by an isolate of Pediococcus from cucumber brines. Appl Microbiol. 1985;30:1040–2.
- Schillinger U, Lucke FK. Identification of lactobacilli from meat and meat products. Food Microbiol. 1987;6:229–42.
- De Vugst L, Vandamme EJ. Bacteriocins of lactic acid bacteria. Microbiology, genetics and applications. London: Blackie Academic and Professional. 1994.
- Netles CG, Barefoot SF. Biochemical and genetic characteristics of bacteriocins of food-associated lactic acid bacteria. J Food Prot. 1993;56:338–56.
- Aktipis A. Production of antimicrobial substances from LAB bacteria isolated from traditional Greek cheeses. PhD Thesis, Agricultural University of Athens, Athens, Greece. 1999.
- Bezirtzoglou E. The intestinal microflora during the first days of life. Anaerobe. 1997;3:173–7.
- Bezirtzoglou E. Contribution a l étude de l implantation de la flore fecale anaerobie du nouveau-ne mis au monde par cesarienne. Doctorat no 13, Paris-Sud. 1985.
- Drasar BS, Hill MJ. Human intestinal flora. New York: Academic Press. 1974.
- Kleessen B, Bezirtzoglou E, Matto J. Culture-based knowledge on biodiversity, development and stability of human gastrointestinal microflora. Microb Ecol Health Dis. 2000; 2:53–63.
- Bezirtzoglou E, Maipa V, Voidarou C, Tsiotsias A, Papapetropoulou M. Food-borne intestinal bacterial pathogens. Microb Ecol Health Dis. 2000;S2:96–104.
- Kawai Y, Watanabe T, Suegara N, Mutai M. Distribution and colonization of human fecal streptococci. Am J Clin Nutr. 1980;33:2458–61.
- Bezirtzoglou E, Romond C. Occurrence of Streptococcus in newborns delivered by cesarian section. Pathogenic streptococci: present and future. St Petersburg, Russia: Lancer Publication. 1994.
- Ozawa K, Yabu-Uchi K, Yamanaka K, Yamashita Y, Nomura S, Oku I. Effect of Streptococcus faecalis BIO-4R on intestinal flora of weanling piglets and calves. Appl Environ Microbiol. 1983;45:1513–18.
- Bosley GS, Facklam RR, Grossman D. Rapid identification of enterococci. J Clin Microbiol. 1983;18:1275–7.
- Bezirtzoglou E, Romond C. Bacterial flora of the gastric fluid in newborns delivered by cesarian section. Borriello SP. Clinical and molecular aspects of anaerobes. Peterfied, UK: Wrightson Biomedical Publishing. 1989.
- Borriello P, Hudson M, Hill M. Investigation of the gastrointestinal bacterial flora. Clin Gastroenterol. 1978;7:329–49.
- Sgouras D, Maragkoudakis PA, Petraki K, Martinez-Gongalez B, Eviotou E, Michopoulos S, . In vitro and in vivo inhibition of Helibacter pylori by Lactobacillus casei strain Shirota. Appl Environ Microbiol. 2004;70:518–26.
- Jacobsen CN, Nielsen R, Hayford AE, Moller PL, Michaelsen KF, Paerregaard A, . Screening of probiotic activities of forty-seven strains of Lactobacillus spp. by in vitro techniques and evaluation of the colonization ability of five selected strains in humans. Appl Environ Microbiol. 1999;65:4949–56.
- Gordon ER, Coresky CA, Chan TH, Perlin AS. The isolation and characterization of bilirubin diglucuronide, the major bilirubin conjugate in dog and human bile. Biochem J. 1976;155:477–81.
- Midtvedt T, Gustafsson BE. Microbial conversion of bilirubin to urobilinogen in vitro and in vivo. Acta Pathol Microbiol Scand [B]. 1981;89:57–80.
- Saxerholt H. Intestinal bilirubin metabolism. PhD Thesis, Karolinska Institutet, Stockholm, Sweden. 1990.
- Hawksworth G, Drasar BS, Hill MJ. Intestinal bacteria and the hydrolysis of glycolytic bonds. J Med Microbiol. 1971; 4:451–9.
- Elliot JA, Facklam RR. Antimicrobial susceptibilities of Lactococcus lactis and Lactococcus garviae and a proposed method to discriminate between them. J Clin Microbiol. 1996;34:1296–8.
- Herrero M, Mayo B, Gonzalez B, Suarez JE. Evaluation of technologically important traits in lactic acid bacteria isolated from spontaneous fermentations. J Appl Bacteriol. 1996;81:565–70.
- Husni RN, Gordon SM, Washington JA, Longworth DL. Lactobacillus bacteremia and endocarditis: review of 45 cases. Clin Infect Dis 1997;25:1048–55.
- Olsson-Liljequist B, Larsson P, Walder M, Miorner H. Antimicrobial susceptibility testing in Sweden. III. Methodology for susceptibility testing in Sweden. Scand J Infect Dis. 1997;105S:13–23.
- Felten A, Barreau C, Biget C, Lagrange PH, Philippon A. Lactobacillus species identification, H2O2 production, and antibiotic resistance and correlation with human clinical status. J Appl Microbiol. 1999;37:729–33.
- Kurland CG, Candack B, Berg OG. Horizontal gene transfer: a critical review. Proc Natl Acad Sci USA. 2003;100: 9658–62.
- Maragkoudakis PA, Zoumpopoulou G, Miaris C, Kalantzopoulos G, Pot B, Tsakalidou EP. Probiotic potential of Lactobacillus strains isolated from dairy products. Int Dairy J. 2006;16:189–99.
- Sholeva Z, Stefaniva S, Chipeva V. Screening of antimicrobial activities among Bulgarian Lactobacillus strains. J Culture Collections. 1997;2:15–20.
- Putman M, Vanveen HW, Degener JE, Kowings WN. The lactococcal secondary multidrug transporter LmrP confers resistance to lincosamides, macrolides, streptogramins and tetracyclines. Microbiology. 2001;147:2873–80.
- Bezirtzoglou E, Romond C. Occurrence of Lactobacillus spp. in newborns delivered by cesarian section. Rev Med Microbiol. 1997;8:101–4.
- Salminen S, Vanwright A, Morelli L, Marteau P, Brassart D, Devos WM, . Demonstration of safety probiotics. Int J Food Microbiol. 1998;44:93–106.
- Franz C, Stiles M, Schleifer M, Schleifer K, Holzapfel W. Enterococci in foods – a conundrum for food safety. Int J Food Microbiol. 2003;88:105–22.
- Florez AB, Delgado S, Mayo B. Antimicrobial susceptibility of lactic acid bacteria isolated from a cheese environment. Can J Microbiol. 2005;51:51–8.