Abstract
Effects of the addition of a cationic amino acid-based synthetic amphiphile, arginine N-lauroyl amide dihydrochloride (ALA), to a lipid-based transfection formulation have been investigated. It is shown that the inclusion of ALA results in a substantial enhancement of the transfection capability of lipoplexes prepared with liposomes of 1-palmitoyl-2-oleoyl-sn-glycero-3-ethylphosphocholine and cholesterol, which themselves mediate highly efficient transfection. A possible explanation for the increased biological activity is that ALA adsorbed to the surface of the DNA-lipid complexes is involved in triggering internalization. However, in order to identify possible additional factors underlying the enhanced transfection efficiency, the physical properties of formulations with and without ALA were characterized using cryo-transmission electron microscopy, dynamic light scattering, and an ethidium bromide intercalation assay. ALA seems to have limited influence on the initial internal structure of the complexes and the protection of DNA, but its presence is found to decrease the average effective size of the dispersed particles; this change in size may be important in improving the biological activity. Furthermore, ALA can act to influence the transfection efficiency of the formulation by promoting the release of DNA following internalization in the transfected cells.
Keywords::
Introduction
The success of gene therapy is critically dependent on the development of efficient gene delivery vectors. A successful vector should be capable to bring about a complex multi-step process involving compaction of DNA into a form deliverable to a cell, transport across the cell membrane, release of the DNA inside the cell, and ultimately expression of the gene. Traditionally, gene therapy has been performed with viral vectors. However, due to potential serious safety issues, including immunogenicity, virus recombination, and oncogenic effects, as well as difficulties in large-scale production pertinent to virus-based formulations, focus has gradually shifted towards non-viral vectors (Crystal Citation1995, Rubanyi Citation2001, Patil et al. Citation2005, Edelstein et al. Citation2007). Among these, the use of complexes based on cationic liposomes, often referred to as lipoplexes, has become one of the most common approaches (Lasic and Templeton Citation1996, Templeton and Lasic Citation1999, de Lima et al. Citation2001, Ulrich Citation2002). As compared to viral vectors, lipid-based gene delivery systems are generally found to be safer, easier to produce in large quantities, and can potentially carry a higher load of DNA. A serious drawback, however, is that these formulations generally show a significantly lower transfection efficiency (de Lima et al. Citation2001, Patil et al. Citation2005, Simões et al. Citation2005).
A large body of work, including evaluation of numerous different lipid mixtures and preparation procedures (Felgner et al. Citation1994, Lasic and Templeton Citation1996, Templeton and Lasic Citation1999, de Lima et al. Citation2001), inclusion of additional components, such as lipids with pH-dependent properties (Felgner et al. Citation1994, Reddy and Low Citation2000, Simoes et al. Citation2001), or compounds that are expected to show specific interactions with receptors on the cell surface (Batra et al. Citation1994, Wagner et al. Citation1994, Simões et al. Citation1999, Reddy and Low Citation2000, Faneca et al. Citation2004), have led to significant improvement in the efficacy of lipid-based gene delivery vectors. Certain trends in respect to the influence of, for instance, particle size and surface charge and the types of lipids used have indeed been identified, but further improvements in both transfection efficiency and the understanding of the critical formulation properties and mechanisms underlying a high biological activity are still essential (de Lima et al. Citation2001, Simões et al. Citation2005).
The larger scope objective of the present work was to perform a systematic investigation of the effects of including selected synthetic amino acid-based amphiphiles in transfection formulations based on cationic liposomes. Since it was found in the late 1980s and early 1990s that certain short peptides, so-called cell penetrating peptides (CPPs), can efficiently cross the plasma membrane, an enormous body of work has been dedicated to the identification of such peptide sequences and their application for mediating cellular internalization of various types of cargo (Patel et al. Citation2007, Stewart et al. Citation2008, Fonseca et al. Citation2009). Thousands of CPPs have since been identified. A majority of the known CPPs have a common feature in a large fraction of basic amino acid residues, particularly arginine, in their sequences. It has been found that the exact amino acid sequence is not necessarily critical, and that just the presence of numerous basic amino acids can induce or improve cellular uptake of particles or aggregates. For instance, a significant improvement in transfection efficiency has been obtained by using arginine-grafted polyamidoamine (PAMAM) dendrimers in the preparation of complexes as compared to unmodified dendrimers (Choi et al. Citation2004). Based on these findings, we considered the possibility that the inclusion of cationic amino acid-based amphiphiles in lipoplexes may result in a similar increase in transfection capability, which would offer the advantage of generating efficient gene delivery vectors that could be easier to produce in large amounts. Furthermore, construction of amphiphiles using polar amino acid residues as building blocks for the head group allow great flexibility to tailor the molecular structures, and thus the properties, of the compounds. Since the preparation of amino acid-based amphiphiles can largely be based on non-toxic building blocks and due to their structural relationship with endogenous substances one can also expect good biocompatibility.
Our initial experiments were carried out with esters prepared from long-chain alcohols and betaine (trimethyl glycine), a class of monovalent cationic, single-tailed amphiphiles that are easy to produce in relatively large amounts (Thompson and Allenmark Citation1989, Lundberg et al. Citation2004). There was indeed a clear tendency for an increase in transfection efficiency when betaine ester amphiphiles, for instance dodecyl betainate, were added to a number of different formulations based on net-cationic lipid mixtures (Lundberg and Faneca, unpublished data). However, there was, unfortunately, a substantial variation in the results between repeated experiments, which after consideration was attributed to the betaine esters simply being too readily hydrolyzed in the relevant environment. The hydrolysis rate for betaine esters is strongly pH-dependent and quite small variations in pH of the medium or wait times in the experimental procedures can result in different extents of hydrolysis of the compounds and thus cause variation in the concentration of intact amphiphile (Thompson and Allenmark Citation1989, Lundberg et al. Citation2004).
Nevertheless, the results from the initial study, indicating that the inclusion of single-tailed cationic amphiphiles can substantially improve the efficacy of lipid-based vectors, were encouraging and in the present work we have evaluated a different type of amino acid-based amphiphile, arginine N-lauroyl amide dihydrochloride (ALA) (molecular structure shown in ). ALA differs from the betaine esters in that it carries two cationic charges and, being an amide, shows higher resistance to chemical hydrolysis. This compound, which is known to show low cytotoxicity and good biodegradability (Moran et al. Citation2001, Rosa et al. Citation2008), has previously been used in a transfection study where it was included as a pre-condensing agent in rather complex formulations containing the protein transferrin and a number of different lipids (Rosa et al. Citation2008); it was concluded that ALA played an important role in inducing the high transfection efficiency mediated by some of the evaluated formulations.
In the present work we assessed the effects of adding ALA to a less complex system involving lipoplexes prepared from ALA, DNA and liposomes composed of equimolar amounts of 1-palmitoyl-2-oleoyl-sn-glycero-3-ethylphosphocholine (EPOPC) and cholesterol (Chol) in different ratios. Lipoplexes prepared from EPOPC:Chol liposomes have previously been shown to be highly efficient (Faneca et al. Citation2004, Citation2008); for instance, they can mediate efficiencies several times higher than formulations prepared with the commonly used DOTAP:DOPE liposomes (Faneca et al. Citation2004). After demonstrating that the addition of ALA to a formulation based on EPOPC:Chol liposomes indeed results in a substantial increase in the biological activity, a significant part of this paper is devoted to the identification of possible changes in the physicochemical character of the formulation in the presence of ALA and attempts to correlate these with the biological activity. To this end, EPOPC:Chol:DNA formulations with and without ALA, at otherwise equivalent conditions, were investigated using cryo-transmission electron microscopy (cryo-TEM) and dynamic light scattering (DLS) to assess morphology and size of the aggregates, and an ethidium bromide intercalation assay to estimate the extent of DNA protection.
Methods
Preparation of EPOPC:Chol liposomes
Small unilamellar liposomes were prepared by extrusion of multilamellar liposomes composed of an equimolar mixture of EPOPC and Cholesterol. Briefly, the appropriate amounts of lipids (Avanti Polar Lipids, Alabaster, AL, USA), dissolved in chloroform, were mixed in a test tube and dried under vacuum in a rotatory evaporator, after which the lipid film was hydrated with deionized water to a final lipid concentration of 6–10 mM. The coarse lipid dispersion was then sonicated for 3 min and extruded 21 times through two stacked polycarbonate filters with a 50 nm pore size using a Liposofast device (Avestin, Toronto, Canada). The resulting liposomes, which are expected to be unilamellar, were diluted five times with deionized water and sterilized by filtration through 0.22 μm pore-size filters (Schleicher & Schuell BioScience, Germany). The liposome dispersions were stored at 4°C until use.
Preparation of vector formulations
Lipoplexes were prepared by first mixing 87.5 μl each of EPOPC:Chol liposome dispersions and ALA solutions previously diluted to the desired concentrations with 175 μl of HEPES buffered saline (HBS; 100 mM NaCl, 20 mM HEPES, pH 7.4). After 15 min incubation at room temperature, 350 μl of a 10 μg/ml solution of pCMVluc DNA (VR-1216; a gift from Dr P. Felgner, Vical, San Diego, CA, USA) in HBS was added to the mixture. The dispersions were then incubated for further 15 min before the start of the transfection experiments. The order of mixing the components was chosen based on the observation that this procedure gave the largest increase in transfection efficiency in the initial experiments with betaine esters. The lipoplexes used in the transfection, cryo-TEM, DLS, and ethidium bromide intercalation experiments were all prepared using the same procedure.
The extent of inclusion of ALA into the EPOPC:Chol liposomes was assessed by estimating the difference in the integrated areas of its sharp solution peaks in 1H-NMR spectra (recorded on a Bruker Avance 500 MHz spectrometer) in the absence or presence of the liposomes; ALA bound to the large liposomes has a significantly decreased spin-spin relaxation time, which gives dramatically broadened NMR signals (Ulmius and Wennerström Citation1977). In a dispersion (prepared in D2O-based buffer) with a composition corresponding to that of the sample with ALA:DNA and EPOPC:DNA +:− charge ratios both of 2:1 before the addition of DNA, the fraction of membrane-bound ALA was estimated to 60–80%.
Cells
TSA cells (BALB/c female mouse mammary adenocarcinoma cell line) were maintained at 37°C, under 5% CO2, in Dulbecco's modified Eagle's medium-high glucose (DMEM-HG) (Irvine Scientific, Santa Ana, CA, USA) supplemented with 10% (v/v) heat-inactivated fetal bovine serum (FBS) (Sigma, St Louis, MO, USA), penicillin (100 U/ml) and streptomycin (100 μg/ml). TSA cells grow in adherent monolayers and were detached by treatment with a trypsin solution (0.25%) (Sigma, St Louis, MO, USA).
Transfection experiments
The transfection activity mediated by the different complexes was evaluated using previously described procedures (Faneca et al. Citation2008). In short, cells were rinsed with serum-free high glucose Dulbecco's modified Eagle's medium (DMEM-HG) (Irvine Scientific, Santa Ana, CA, USA) and then covered with 300 μl of the same medium before 200 μl of the lipoplex dispersions, always containing a total of 1 μg of DNA, were carefully added to each well. After 4 h incubation in an atmosphere of 5% CO2 at 37°C the medium was replaced with DMEM-HG containing 10% fetal bovine serum (FBS) (Sigma, St Louis, MO, USA), and the cells were further incubated for 48 h. After assessment of cell viability, see below, the cells were washed with phosphate buffered saline (PBS) after which 100 μl of lysis buffer (1 mM DTT, 1 mM EDTA, 25 mM Tris-phosphate (pH 7.8), 8 mM MgCl2, 15% glycerol, 1% (v/v) Triton X-100) was added to each well. The plates were then gently shaken for ∼ 2 min and kept at −80°C for at least 10 min to ensure complete lysis. The level of gene expression was evaluated by measuring light production by luciferase in the lysates using a LMax II 384 luminometer (Molecular Devices, Union City, CA, USA). The total amount of protein in each lysate was determined using the DC protein assay (Bio-Rad, Hercules, CA, USA) with bovine serum albumin (BSA) as the standard. The transfection activity data are presented as relative light units (RLU) of luciferase per mg of total cell protein.
Cell viability assay
Following the transfection experiments, cell viability was assessed by a modified Alamar Blue assay (Konopka et al. Citation1996). This assay, which allows determination of cell viability without detachment of adherent cells, gives a relative measure of the metabolic activity of the cells, which is proportional to the number of living cells.
In short, the medium in the respective wells was replaced by 0.4 ml of 1% (v/v) Alamar Blue dye in complete DMEM-HG medium. After incubation for ∼ 1 h at 37°C, 150 μl of the supernatant from each well was transferred to a 96-well plate. Absorbance values at 570 and 600 nm were measured using a SpectraMax Plus 384 UV spectrometer (Molecular Devices, Union City, CA, USA) and cell viability (as percentage of positive control), CV, was calculated according to Equation (1):
where: subscripts nc and pc denote negative control, i.e., no cells, and positive control, i.e., cells without added formulation at otherwise identical conditions, respectively.
Cryo-TEM
Samples for the cryo-TEM experiments were prepared using a controlled environment vitrification system (CEVS) (Bellare et al. Citation1988), where the relative humidity was kept close to saturation at around 30°C. A 5 μl drop was placed on a lacey carbon-coated copper grid and the excess fluid was gently blotted away leaving a thin film of aqueous sample covering the grid. The grid was then plunged into liquid ethane at −180°C to allow rapid vitrification of the specimen (and thus avoid crystallization of water). All prepared grids were stored in liquid nitrogen until being transferred to the electron microscope. Images were digitally recorded using a Philips CM120 Bio TWIN electron microscope, operated at 120 kV, at around −183°C, equipped with a Gatan MSC791 cooled CCD camera system. The investigated lipoplexes showed notable beam sensitivity, and to minimize beam damage all samples were imaged under minimal electron dose conditions.
DLS
The DLS measurements were performed on a Zetasizer Nano ZS instrument from Malvern Instruments Ltd, Worcestershire, UK. This instrument measures, using non-invasive backscatter (NIBS) technology, fluctuations in the intensity of scattered light at a set angle of 173° and constructs an intensity autocorrelation function of the scattered light. The laser used is a 4 mW He-Ne laser (632.8 nm) and the detection unit comprises an avalanche photodiode. The corresponding electric field correlation functions were analyzed using the linear regularized inverse Laplace transformation method CONTIN (Provencher Citation1979, Citation1982), which was provided in the instrument software, in order to obtain z-average size distributions in terms of apparent hydrodynamic diameters (D H,app; obtained values are apparent since they are measured at finite concentrations). Polydispersity indices (PDI) were calculated from cumulant fits of the data (Štěpánek Citation1993).
The D H,app and PDI data presented for each sample are averages from at least five measurements. All presented data are based on monomodal size distributions and the variation in average size between experiments was generally within a few percent from the presented numbers.
Ethidium bromide intercalation assay
The accessibility of ethidium bromide to DNA in the lipoplexes at 37°C was monitored using a SPEX Fluorolog 2 fluorometer (SPEX Industries, Edison, NJ, USA), with an experiment time of 2 min. The fluorescence was read at excitation and emission wavelengths of 518 and 605 nm, respectively, using 1 mm excitation and 2 mm emission slits. The sample chamber was equipped with a magnetic stirring device, and the temperature was controlled with circulating water from a thermostated water bath. The fluorescence scale was calibrated so that the initial fluorescence of EtBr (10 μl of a 2.5 mM solution added to a cuvette containing 2 ml HBS solution) was set as residual fluorescence. The value of fluorescence obtained upon the addition of 1 μg DNA (control) was set as 100%. 200 μl of lipoplex dispersion, containing a total of 1 μg DNA, was added to a cuvette containing 1.8 ml HBS solution followed by addition of 10 μl of EtBr. The fraction of DNA accessible to interact with the probe molecule was calculated by subtracting the values of residual fluorescence from those obtained for the samples and expressed in terms of percentage of the value for the control.
Results
Transfection activity
shows the effect of ALA on the transfection activity mediated by EPOPC:DNA lipoplexes prepared at the indicated charge ratios. It is observed for both of the investigated EPOPC:DNA +:− charge ratios that the presence of ALA, at otherwise equal conditions, results in a significant improvement in the biological activity of the formulations. It has previously been shown, in different cell lines, that for lipoplexes prepared with EPOPC:Chol liposomes, in the absence of additional components, the highest transfection efficiency is achieved at a +:− charge ratio of 2:1 (Faneca et al. Citation2004, Citation2008). As seen in , the addition of ALA in amounts corresponding to ALA:DNA +:− charge ratios of 2:1 or 4:1 induces an approximately three-fold increase in the transfection efficiency relative to the highest activity with EPOPC:Chol liposomes alone.
Figure 2. Transfection efficiency, in terms of relative light units (RLU) of expressed luciferase per mg of total protein at the end of the experiment as a function of formulation composition. EPOPC:DNA +:− charge ratios are stated by each group of bars, whereas ALA:DNA +:− charge ratios are indicated by the different shades according to the legend to the right (NA = no ALA). The error bars show +/− the standard errors as calculated from the results on triplicates. These results are representative of at least three independent experiments.
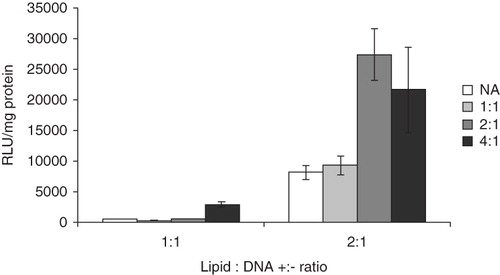
Consistent with previous findings (Rosa et al. Citation2008), formulations prepared with ALA as the only amphilphile (i.e., in the absence of liposomes) show a negligible capacity of mediating transfection.
Cell viability
Cell viabilities in the presence of the different investigated formulations as assessed using a modified Alamar Blue assay are shown in . Although complexes including ALA show a somewhat higher toxicity as compared to those prepared with liposomes only, the cell viabilities are high, above ∼ 85%, with all studied formulations.
Figure 3. Cell viability, relative to control, after exposure to formulations of different composition. EPOPC:DNA +:− charge ratios are stated by each group of bars (NL = no liposomes), whereas ALA:DNA +:− charge ratios are indicated by the different shades according to the description to the right (NA = no ALA). The error bars show +/− the standard errors as calculated from the results on triplicates. These results are representative of at least three independent experiments.
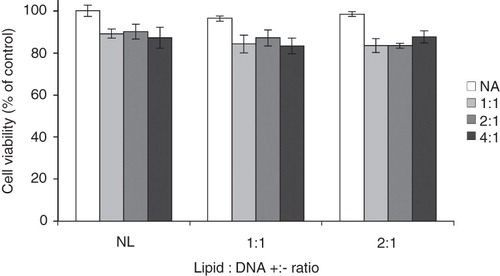
Cryo-TEM
shows representative cryo-TEM images of lipoplex formulations with or without ALA. The images suggest that both of the investigated compositions give complexes of similar appearance, with ‘primary’ aggregates of an almost perfectly spherical shape and similar size, with diameters of the order of 100 nm, and a rather narrow size distribution, which are clustered into larger entities. The close-up micrographs (4 b and d) reveal that also the inner structure of the spherical units seems to be very similar in the two formulations. Although the contrast is rather low, one can see indications that these have a multilamellar structure built up of concentric layers in an onion-like fashion. There is a variation in density within some of the complexes suggesting gaps between some of the lipid bilayers, which can be supposed to be water-filled domains. No free DNA and very few remaining unilamellar liposomes are observed.
Figure 4. Cryo-TEM images of samples prepared using the same procedure as those used in the transfection experiments with a EPOPC:DNA +:− charge ratio of 2:1, without (a, b) or with (c, d) the inclusion of ALA in an amount corresponding to a +:− charge ratio of 2:1, in two different magnifications for each sample.
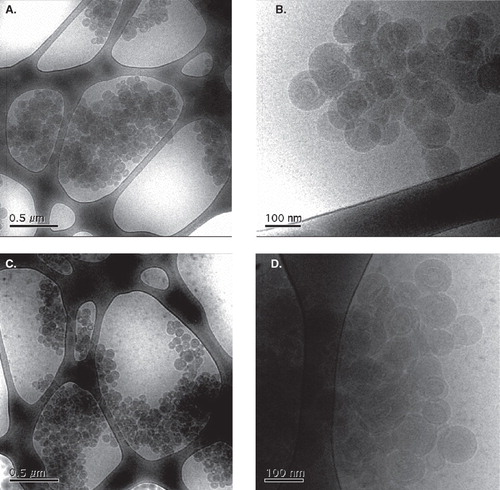
DLS
Selected data from the DLS experiments are presented in . If one first considers the numbers for liposomes in the absence and presence of ALA, one can see that the added amphiphile does not induce any significant changes in the effective size of the liposomes within the relevant time span, i.e., the 15 min during which the EPOPC:Chol liposomes are incubated with ALA before addition of the plasmid.
Table I. Size of particles in formulations of different composition.
For the complete lipoplexes, on the other hand, there is a notable difference in the initial apparent size of the dispersed entities. For both formulations there is then a gradual increase in size over time, which, in terms of relative volume increase, is roughly the same for the different compositions over the relevant time span.
Ethidium bromide intercalation assay
Ethidium bromide intercalation experiments indicate that for samples corresponding to those characterized by cryo-TEM and DLS there is no significant difference in DNA protection. The fraction of DNA accessible to the fluorescent probe is 28.1 ± 3.5 and 31.6 ± 1.5% (SE; n = 3) with and without ALA, respectively, relative to that observed in the absence of amphiphiles. When experiments were repeated for samples maturated for 1 h, the change in fluorescence was within the experimental error.
Discussion
shows that the inclusion of ALA in a lipoplex formulation based on EPOPC:Chol liposomes results in a significant increase in transfection efficiency. By comparing the results for the liposome-DNA formulation (EPOPC:DNA) with a +:− charge ratio of 2:1 with that for the liposome-ALA-DNA formulation with +:− charge ratios for both EPOPC:DNA and ALA:DNA of 1:1 (which thus has a total +:− amphiphile:DNA charge ratio of 2:1) it is clear that the increase in biological activity cannot simply be attributed to the presence of a larger amount of cationic amphiphile. This finding, in combination with the fact that ALA in itself does not mediate transfection, suggests that the higher biological activity of the liposome-ALA-DNA formulations is a consequence of some sort of synergistic effect.
Cationic amphiphiles and cationic lipoplexes often show some cytotoxic activity (Lasic and Templeton Citation1996, de Lima et al. Citation2001). In order to assess the extent of toxicity of the herein used lipoplex formulations, cell viability at the end of the transfection experiment was determined. As can be seen in , the formulations containing ALA show a somewhat higher toxicity as compared to those prepared with liposomes only. However, the difference in cell viability is definitely not large enough to explain the observed difference in transfection efficiency in the absence or presence of ALA (this is important to note since the transfection efficiency is expressed as RLU/mg of total protein, where the latter depends on the total number of cells).
Following administration, a gene delivery formulation encounters a number of physical and chemical obstacles on the route towards the nucleus, and in order for expression of the carried gene(s) to ultimately occur, the following conditions need to be fulfilled (Simoes et al. Citation1999, Zuhorn and Hoekstra Citation2002, Wiethoff and Middaugh Citation2003, Lechardeur et al. Citation2005, Zuhorn et al. Citation2007):
The DNA and the vehicle need to have a certain stability in the extracellular environment;
The complexes have to be taken up by the cells, which can occur via different possible routes of endocytosis;
The complexes have to escape from the endosomes before trafficking to lysosomes;
The released cargo needs to withstand transport through the cytosol; and eventually
The plasmid needs to be translocated into the nucleus for transcription to occur.
Based on the previous finding that transfection efficacy of complexes prepared with arginine-grafted dendrimers is substantially higher than that obtained with the corresponding unmodified, ‘naked’ dendrimers (Choi et al. Citation2004), one can make the reasonable suggestion that the good results obtained with the formulations containing ALA can, at least in part, be ascribed to the presence of arginine in the surfactant head groups. In turn, one can plausibly attribute an improvement in biological activity with arginine residues present in the formulations to a ‘CPP-like’ mechanism.
However, the ‘CPP mechanism’ is but one in a range of possible explanations to the increased activity in presence of ALA; as will be discussed in the following, there is a number of possible additional ways by which this compound might improve the performance of a transfection formulation. In pursuit of observable changes in the physical character of the EPOPC:DNA lipoplexes upon the addition of ALA, formulations with and without ALA were characterized with respect to the size and structure of the complexes as well as the extent of DNA protection. Emphasis was on two formulations both having a EPOPC:DNA +:− ratio of 2:1, one prepared with liposomes and DNA only and the other with ALA added to the mixture in an amount corresponding to a ALA:DNA +:− charge ratio of 2:1. The EPOPC:DNA ratio was chosen because this composition, as was also mentioned above, results in the highest biological activity in the absence of additional components (Faneca et al. Citation2004) and the ALA:DNA ratio was selected since this, at least out of the herein evaluated compositions, gives the largest enhancement in transfection efficiency. In order to allow correlation with the results from the biological experiments, the samples for the physical characterization were prepared using the same procedure as when preparing those for the former experiments.
The sizes and structures of the lipoplexes in the different formulations were assessed using a combination of cryo-TEM and DLS. Cryo-TEM and DLS are two fundamentally different techniques that favorably complement each other. The former technique allows visualization of the shape and morphology of microscopic aggregates whereas the latter can give estimates of the effective sizes of dispersed particles.
As seen in the cryo-TEM images in , the formulations with or without ALA give very similar aggregates, which most likely are best described as multilamellar vesicles. Lamellar structures are commonly observed in complexes prepared from DNA and liposomes containing cationic lipids. In cases with well-defined model systems and thoroughly equilibrated samples highly ordered structures with the DNA chains sandwiched between parallel lipid bilayers can often be identified by, for instance, X-ray diffraction experiments (Lasic et al. Citation1997, Rädler et al. Citation1997, Smisterova et al. Citation2001) or cryo-electron micrographs (Lasic et al. Citation1997, Schmutz et al. Citation1999, Huebner et al. Citation1999, Xu et al. Citation1999, Simberg et al. Citation2001). However, the actual formulations used in transfection studies, which are typically prepared shortly before the experiments, do, in addition to a common polydispersity in size, sometimes also show significant heterogeneity with respect to the structure of the complexes (Lasic et al. Citation1997, Rosa et al. Citation2008). With a significant variation in character of the observed aggregates it is not straightforward to establish an ‘average’ or ‘typical’ particle and thus difficult to correlate formulation properties to biological activity. However, since the herein investigated formulations show good homogeneity, a discussion on the matter is justified.
EPOPC:Chol liposomes constitute one of the most efficient cationic liposome formulations for gene delivery tested in our laboratory (Faneca et al. Citation2004, Citation2008). The finding that this formulation appears to give rather homogeneous complexes with a lamellar internal structure can be taken to support an importance of well-ordered aggregates. However, since the cryo-TEM images do not reveal any obvious changes in the structure of the aggregates on addition of ALA, these results do not provide any direct clues to the improvement in performance of the formulation.
Although ALA does not induce any changes in the initial complexes detectable by cryo-TEM, it is nonetheless valuable to consider the expected consequences of the addition of a single-tailed amphiphile to a lipid bilayer and their possible implications on the aggregate properties. A single-tailed amphiphile has, in comparison to a twin-tailed lipid, typically a smaller volume of the tail part relative to the size of the hydrophilic head group and will thus, for geometric reasons, promote transitions into structures with an on average positive curvature (i.e., curved over the hydrophobic domain) of the lipid film (Israelachvili et al. Citation1976). For a related system (aqueous mixtures of egg lecithin and dodecyltrimethylammonium chloride [without DNA]) there is a transition from lamellar phase into a (normal) hexagonal phase at a mole ratio of single-tailed amphiphile to lipid above the order of one (Gustafsson et al. Citation1997a). In other similar systems (e.g., lecithin and hexadecyltrimethylammonium chloride), it has been observed that addition of a single-tailed cationic surfactant to liposomes induces the formation of pores in the bilayers (Gustafsson et al. Citation1997b). In the latter case there is an overall retention of a bilayer arrangement but with regions of locally high curvature. It is reasonable that the strong electrostatic interaction between the lipid bilayers and the DNA, in combination with a requirement of charge neutrality of the separate phase, imposes geometrical constraints that counteract a phase transition. It is conceivable, however, that the presence of ALA in the aggregates causes an internal ‘frustration’ in the lipid bilayers, which, with changes in the external conditions, could induce structural transformations and/or destabilization of the complexes.
Several investigations have shown that lipoplex formulations that promote the formation of non-lamellar structures in the endosomal environment mediate high transfection efficacies (Felgner et al. Citation1994, Koltover et al. Citation1998, Smisterova et al. Citation2001, Koynova et al. Citation2005, Citation2006, Zuhorn et al. Citation2005). For instance, it is well known that formulations based on lipid mixtures containing the zwitterionic lipid dioleylphosphatidylethanolamine (DOPE), which induces a spontaneous negative curvature at lower pH, are highly efficient, which has been explained by fusion with and destabilization of endosomal membranes. It has recently been found that there is a general strong correlation between transfection efficiency and the phase structure formed when a lipoplex is mixed with anionic and/or zwitterionic lipids representative of those found in cellular membranes; the former increases strongly with an increased tendency for non-lamellar structures on mixing, the formation of which is believed to promote an efficient release of the DNA from the endosomes (Koynova et al. Citation2005, Citation2006, Koynova and MacDonald Citation2007, Pozzi et al. Citation2009).
On the basis that ALA is expected to promote curved structures, it is reasonable to suggest that part of the increased transfection efficiency in its presence can be ascribed to destabilization of the lipoplexes and the endosomal membrane, thus facilitating the crucial DNA release.
Another, but related, possible influence of ALA in promoting release of DNA from the endosomes is related to the aqueous solubility of the compound. A single-tailed amphiphile has dramatically higher molecular solubility as compared to a twin-tailed lipid, and it has been suggested that single-tailed cationic amphiphiles included in lipoplexes are individually dissolved and promote release of the lipoplexes from endosomes by destabilization of the endosomal membrane (Pinnaduwage et al. Citation1989). Individual cationic amphiphile monomers have a strong potential to affect integrity of the endosomal membrane by a combination of hydrophobic and electrostatic interactions and are expected to diffuse much faster than the lipoplex particles.
The presence of ‘open’ domains in the complexes, which are observed both with and without ALA, can reasonably be explained by the excess of cationic lipid as compared to the total number of negative charges from DNA. Since essentially no free liposomes are observed, practically all of the lipid present should reside in the lipoplexes. This requires that surfaces of considerable cationic charge density are forced into close proximity. The integrity of the complexes can likely be explained by bridging of the bilayers by DNA, but it is not unlikely that the DNA is unevenly distributed in the multilamellar structure and that in regions of lower DNA density, repulsion between the cationic lipid surfaces causes the formation of cavities.
If judging from the cryo-TEM images, clustering of the primary spherical aggregates seems to occur in a roughly similar extent in both of the investigated formulations. It is important to note, however, that the observed clustering might in part be an artifact arising in the sample preparation procedure; when a TEM grid is blotted after application of the sample, a certain influence from flow and shear forces, which can cause changes in concentration and induce heterogeneities, is practically inevitable (Cui et al. Citation2007). In this regard, it is relevant to compare the findings from the cryo-TEM to the results from DLS, which are better representative of the situations in the unperturbed bulk samples. As can be seen in , for all investigated samples, the sizes obtained with DLS are significantly larger than the diameters of the spheres observed in the cryo-TEM images, which indicate that the average dispersed entity is indeed a cluster of several multilamellar liposomes. Clustering of the primary aggregates can likely be attributed to bridging between the subunits by DNA chains.
Furthermore, shows that when ALA is present in the system the effective size of the dispersed entities is substantially smaller than in the formulation without it; this is true in the whole investigated time span. After 5 h, the apparent aggregate size is notably increased in both formulations. In measures of the relative increases in volume, however, the growth in cluster size is comparable in the two cases. A simple but likely explanation to the decreased propensity for clustering in the presence of ALA is that these aggregates have a higher surface charge density, which provides for an improved colloidal stability.
If one assumes that the spherical primary aggregates have a diameter of 100 nm and are randomly close-packed, which means that the volume fraction of spheres is 0.64 (Scott and Kilgour Citation1969), the initial average cluster contains a maximum of 30 and 90 spherical units in the cases with and without ALA, respectively. It can be noted that, judging from the DLS results and the calculation presented above, the cluster viewed in would be roughly representative of a dispersed particle in the formulation with ALA at the point of addition to the cells.
Particle size is one of the parameters determining the type of endocytic pathway for cellular internalization of lipoplexes (Simoes et al. Citation1999, Conner and Schmid Citation2003, Rejman et al. Citation2004). In an investigation with the aim to identify the mechanisms of endocytotic internalization for particles of different sizes at otherwise unchanged conditions (which would be virtually impossible to accomplish for a representative lipoplex formulation) (Rejman et al. Citation2004), the uptake in non-phagocytotic murine melanoma cells of a range of practically monodisperse fluorescent latex beads was investigated. It was found that particles with diameters <200 nm were almost exclusively internalized by clathrin-mediated endocytosis, whereas 500 nm particles were preferentially taken up by a calveolae-mediated pathway, and essentially no uptake was observed for particles 1 μm in size. Furthermore, it was observed that the overall efficiency of internalization decreased with particle size. Under the assumption that these are representative numbers for the respective modes of endocytosis, the complexes both with and without ALA are, at the point of addition to the cells, largely in the size range for calveolae-mediated endocytosis. However, there is a significant heterogeneity in size of the lipoplexes and it is possible that a change in the relative fractions of particles taken up by different endocytotic mechanisms, and that, in turn, the respective associated intracellular pathways lead to different transfection efficiency for the lipoplexes in question. It can also be argued that with the generally smaller particle size in the presence of ALA, a larger fraction of particles remains within the size limits of the more efficient pathways for a more extended time period. However, it is important to emphasize that a change in particle size is not an exclusive possible explanation for an increased endocytotic uptake but that also other physical parameters, such as particle surface character (also see above), are important.
The transported DNA is vulnerable to metabolic degradation at several stages on the route towards the nucleus and an important function of a successful vector formulation is to provide protection of the load. On the other hand, the formation of extremely stable complexes between DNA and a compacting agent could be detrimental to a transfection formulation, since it may complicate the essential release of the carried DNA. In practice, the biological activity of a lipoplex is thus dependent on a balance between factors providing protection of DNA and factors promoting its release.
A common means of estimating the exposure of DNA in a transfection formulation to the surrounding is to determine the accessibility to specific DNA-binding probes. Ethidium bromide is a DNA-intercalating agent whose fluorescence is dramatically enhanced upon binding to DNA. The high fluorescence will be quenched when the probe molecule is displaced by a compound with higher affinity for DNA or upon condensation of DNA.
The fact that there is no significant difference in access of DNA to the ethidium bromide with or without ALA suggests that there is no difference in protection in the respective formulations at the point of addition to the cells. Together with the discussion on lipoplex destabilization above, this finding suggests that ALA has a larger influence by promoting release rather than providing protection of DNA.
Conclusion
It has been shown that inclusion of the synthetic, amino acid-based amphiphile ALA into lipoplex formulations prepared from EPOPC:Chol liposomes can induce a substantial increase in the transfection capacity. Based on the previous knowledge that presence of arginine can induce transmembrane transport of particles, a likely explanation to the increased biological activity is that ALA adsorbed to the surface of the complexes triggers cellular internalization. In an attempt to elucidate possible additional mechanisms in which ALA might participate to improve the transfection efficiency, formulations with and without the amphiphile were characterized. It was found that the formulations form spherical primary aggregates that are very similar in both size and morphology independently of the presence of ALA. Furthermore, it is shown that the initial protection of DNA, in terms of access to a fluorescent probe, was essentially the same with and without ALA. The most obvious consequence on the physical characteristics of the formulation from the inclusion of ALA is that the apparent average size of the dispersed entities is smaller in the presence of the amphiphile. This finding can be taken to suggest that the improvement in transfection from the presence of ALA might, at least in part, be explained by this keeping the dimensions of the particles within a size span suitable for a more efficient route of internalization over the duration of the experiment. Furthermore, there are arguments that ALA, following internalization to the transfected cells, can act to promote release of the DNA by destabilizing the lipoplexes and/or the endosomal membrane.
Acknowledgments
The authors would like to thank Gunnel Karlsson of Lund University, who performed the cryo-TEM experiments.
Declaration of interest: This work was financially supported by the Hans Werthén foundation, the sixth EU framework program as being part of a EU-STREP project with NEST program (NEONUCLEI, Contract 12967), the Linnaeus Center of Excellence on Organizing Molecular Matter through the Swedish Research Council, and Fundação para a Ciência e a Tecnologia (Portuguese Science Council) (Contracts SFRH/BPD/48522/2008, SFRH/BPD/43838/2008, and PPCDT/QUI/58689/2004). The authors report no conflicts of interest. The authors alone are responsible for the content and writing of the paper.
References
- Batra RK, Wangjohanning F, Wagner E, Garver RI, Curiel DT. 1994. Receptor-mediated gene delivery employing lectin-binding specificity. Gene Ther 1:255–260.
- Bellare JR, Davis HT, Scriven LE, Talmon Y. 1988. Controlled environment vitrification system – an improved sample preparation technique. J Electron Microscopy Techn 10:87–111.
- Choi JS, Nam K, Park J, Kim JB, Lee JK, Park J. 2004. Enhanced transfection efficiency of PAMAM dendrimer by surface modification with L-arginine. J Controlled Release 99:445–456.
- Conner SD, Schmid SL. 2003. Regulated portals of entry into the cell. Nature 422:37–44.
- Crystal RG. 1995. Transfer of genes to humans – early lessons and obstacles to success. Science 270:404–410.
- Cui H, Hodgdon TK, Kaler EW, Abezgauz L, Danino D, Lubovsky M, Talmon Y, Pochan DJ. 2007. Elucidating the assembled structure of amphiphiles in solution via cryogenic transmission electron microscopy. Soft Matter 3:945.
- de Lima MCP, Simoes S, Pires P, Faneca H, Duzgunes N. 2001. Cationic lipid-DNA complexes in gene delivery: From biophysics to biological applications. Adv Drug Del Rev 47:277–294.
- Edelstein ML, Abedi MR, Wixon J. 2007. Gene therapy clinical trials worldwide to 2007 – an update. J Gene Med 9:833–842.
- Faneca H, Faustino A, De Lima MCP. 2008. Synergistic antitumoral effect of vinblastine and HSV-Tk/GCV gene therapy mediated by albumin-associated cationic liposomes. J Contr Release 126:175–184.
- Faneca H, Simoes S, de Lima MCP. 2004. Association of albumin or protamine to lipoplexes: Enhancement of transfection and resistance to serum. J Gene Med 6:681.
- Felgner JH, Kumar R, Sridhar CN, Wheeler CJ, Tsai YJ, Border R, Ramsey P, Martin M, Felgner PL. 1994. Enhanced gene delivery and mechanism studies with a novel series of cationic lipid formulations. J Biol Chem 269:2550–2561.
- Fonseca SB, Pereira MP, Kelley SO. 2009. Recent advances in the use of cell-penetrating peptides for medical and biological applications. Adv Drug Del Rev 61:953–964.
- Gustafsson J, Orädd G, Almgren M. 1997a. Disintegration of the lecithin lamellar phase by cationic surfactants. Langmuir 13:6956–6963.
- Gustafsson J, Orädd G, Lindblom G, Olsson U, Almgren M. 1997b. A defective swelling lamellar phase. Langmuir 13:852–860.
- Huebner S, Battersby BJ, Grimm R, Cevc G. 1999. Lipid-DNA complex formation: Reorganization and rupture of lipid vesicles in the presence of DNA as observed by cryoelectron microscopy. Biophys J 76:3158–3166.
- Israelachvili JN, Mitchell DJ, Ninham BW. 1976. Theory of self-assembly of hydrocarbon amphiphiles into micelles and bilayers. J Chem Soc Faraday Trans II 72:1525–1568.
- Koltover I, Salditt T, Radler JO, Safinya CR. 1998. An inverted hexagonal phase of cationic liposome-DNA complexes related to DNA release and delivery. Science 281:78–81.
- Konopka K, Pretzer E, Felgner PL, Duzgunes N. 1996. Human immunodeficiency virus type-1 (HIV-1) infection increases the sensitivity of macrophages and THP-1 cells to cytotoxicity by cationic liposomes. Biochim Biophys Acta – Molec Cell Res 1312:186–196.
- Koynova R, MacDonald RC. 2007. Natural lipid extracts and biomembrane-mimicking lipid compositions are disposed to form nonlamellar phases, and they release DNA from lipoplexes most efficiently. Biochim Biophys Acta – Biomembranes 1768:2373–2382.
- Koynova R, Wang L, MacDonald RC. 2006. An intracellular lamellar-nonlamellar phase transition rationalizes the superior performance of some cationic lipid transfection agents. Proc Nat Acad Sci USA 103:14373–14378.
- Koynova R, Wang L, Tarahovsky Y, MacDonald RC. 2005. Lipid phase control of DNA delivery. Bioconjugate Chem 16:1335–1339.
- Lasic DD, Strey H, Stuart MCA, Podgornik R, Frederik PM. 1997. The structure of DNA-liposome complexes. J Am Chem Soc 119:832–833.
- Lasic DD, Templeton NS. 1996. Liposomes in gene therapy. Adv Drug Deliv Rev 20:221–266.
- Lechardeur D, Verkman AS, Lukacs GL. 2005. Intracellular routing of plasmid DNA during non-viral gene transfer. Adv Drug Del Rev 57:755–767.
- Lundberg D, Ljusberg-Wahren H, Norlin A, Holmberg K. 2004. Studies on dodecyl betainate in combination with its degradation products or with phosphatidyl choline-phase behavior and hemolytic activity. J Colloid Interface Sci 278:478–487.
- Morán C, Clapes P, Comelles F, Garcia T, Perez L, Vinardell P, Mitjans M, Infante MR. 2001. Chemical structure/property relationship in single-chain arginine surfactants. Langmuir 17:5071–5075.
- Patel LN, Zaro JL, Shen WC. 2007. Cell penetrating peptides: Intracellular pathways and pharmaceutical perspectives. Pharm Res 24:1977–1992.
- Patil SD, Rhodes DG, Burgess DJ. 2005. DNA-based therapeutics and DNA delivery systems: A comprehensive review. AAPS J 7:E61–77.
- Pinnaduwage P, Schmitt L, Huang L. 1989. Use of a quaternary ammonium detergent in liposome mediated DNA transfection of mouse L-cells. Biochim Biophys Acta 985:33–37.
- Pozzi D, Caracciolo G, Caminiti R, De Sanctis SC, Amenitsch H, Marchini C, Montani M, Amici A. 2009. Toward the rational design of lipid gene vectors: Shape coupling between lipoplex and anionic cellular lipids controls the phase evolution of lipoplexes and the efficiency of DNA release. ACS Appl Mater Interfaces 1:2237–2249.
- Provencher SW. 1979. Inverse problems in polymer characterization – direct analysis of polydispersity with photon correlation spectroscopy. Macromol Chem Phys 180:201–209.
- Provencher SW. 1982. Contin – a general-purpose constrained regularization program for inverting noisy linear algebraic and integral-equations. Comp Phys Comm 27:229–242.
- Rädler JO, Koltover I, Salditt T, Safinya CR. 1997. Structure of DNA-cationic liposome complexes: DNA intercalation in multilamellar membranes in distinct interhelical packing regimes. Science 275:810–814.
- Reddy JA, Low PS. 2000. Enhanced folate receptor mediated gene therapy using a novel pH-sensitive lipid formulation. J Contr Release 64:27–37.
- Rejman J, Oberle V, Zuhorn IS, Hoekstra D. 2004. Size-dependent internalization of particles via the pathways of clathrin-and caveolae-mediated endocytosis. Biochem J 377:159–169.
- Rosa M, Penacho N, Simoes S, Lima MCP, Lindman B, Miguel MG. 2008. DNA pre-condensation with an amino acid-based cationic amphiphile. A viable approach for liposome-based gene delivery. Mol Membr Biol 25:23–34.
- Rubanyi GM. 2001. The future of human gene therapy. Mol Aspects Med 22:113–142.
- Schmutz M, Durand D, Debin A, Palvadeau Y, Etienne A, Thierry AR. 1999. DNA packing in stable lipid complexes designed for gene transfer imitates DNA compaction in bacteriophage. Proc Nat Acad Sci USA 96:12293–12298.
- Scott GD, Kilgour DM. 1969. Density of random close packing of spheres. J Phys D-Appl Phys 2:863–866.
- Simberg D, Danino D, Talmon Y, Minsky A, Ferrari ME, Wheeler CJ, Barenholz Y. 2001. Phase behavior, DNA ordering, and size instability of cationic lipoplexes – relevance to optimal transfection activity. J Biol Chem 276:47453–47459.
- Simões S, Filipe A, Faneca H, Mano M, Penacho N, Düzgünes N, de Lima MCP. 2005. Cationic liposomes for gene delivery. Expert Opin Drug Deliv 2:237–254.
- Simões S, Pires P, Duzgunes N, de Lima MCP. 1999. Cationic liposomes as gene transfer vectors: Barriers to successful application in gene therapy. Curr Opin Mol Ther 1:147–157.
- Simoes S, Slepushkin V, Duzgunes N, de Lima MCP. 2001. On the mechanisms of internalization and intracellular delivery mediated by pH-sensitive liposomes. Biochim Biophys Acta – Biomembranes 1515:23–37.
- Simões S, Slepushkin V, Pires P, Gaspar R, de Lima MCP, Duzgunes N. 1999. Mechanisms of gene transfer mediated by lipoplexes associated with targeting ligands or pH-sensitive peptides. Gene Ther 6:1798.
- Smisterova J, Wagenaar A, Stuart MCA, Polushkin E, ten Brinke G, Hulst R, Engberts JBFN, Hoekstra D. 2001. Molecular shape of the cationic lipid controls the structure of cationic lipid/dioleylphosphatidylethanolamine-DNA complexes and the efficiency of gene delivery. J Biol Chem 276:47615–47622.
- Štěpánek P. 1993. In: Brown W, editor. Dynamic light scattering: The method and some applications. Oxford, UK: Oxford University Press.
- Stewart KM, Horton KL, Kelley SO. 2008. Cell-penetrating peptides as delivery vehicles for biology and medicine. Org Biomol Chem 6:2242–2255.
- Templeton NS, Lasic DD. 1999. New directions in liposome gene delivery. Mol Biotechnol 11:175–180.
- Thompson RA, Allenmark S. 1989. Effects of molecular association on the rates of hydrolysis of long-chain alkyl betainates (alkoxycarbonyl-N,N,N-trialkylmethanaminium halides). Acta Chem Scand 43:690–693.
- Ulmius J, Wennerström H. 1977. Proton NMR bandshapes for large aggregates – micellar solutions of hexadecyltrimethylammonium bromide. J Magn Reson 28:309.
- Ulrich AS. 2002. Biophysical aspects of using liposomes as delivery vehicles. Biosci Rep 22:129–150.
- Wagner E, Curiel D, Cotten M. 1994. Delivery of drugs, proteins and genes into cells using transferrin as a ligand for receptor-mediated endocytosis. Adv Drug Del Rev 14:113–135.
- Wiethoff CM, Middaugh CR. 2003. Barriers to nonviral gene delivery. J Pharmaceut Sci 92:203–217.
- Xu YH, Hui SW, Frederik P, Szoka FC. 1999. Physicochemical characterization and purification of cationic lipoplexes. Biophys J 77:341–353.
- Zuhorn IS, Bakowsky U, Polushkin E, Visser WH, Stuart MCA, Engberts JBFN, Hoekstra D. 2005. Nonbilayer phase of lipoplex-membrane mixture determines endosomal escape of genetic cargo and transfection efficiency. Mol Ther 11:801–810.
- Zuhorn IS, Engberts JBFN, Hoekstra D. 2007. Gene delivery by cationic lipid vectors: Overcoming cellular barriers. Eur Biophys J 36:349–362.
- Zuhorn IS, Hoekstra D. 2002. On the mechanism of cationic amphiphile-mediated transfection. To fuse or not to fuse: Is that the question? J Membr Biol 189:167–179.