Abstract
Lipid rafts constitute dynamic assemblies within a bilayer, engaged in, e.g., signal transduction, membrane trafficking and cell polarization. Despite wide interest in the process of domain formation in binary or ternary lipid model systems, only a limited number of papers are devoted to the influence of different additives on this process. In particular, works devoted to the role of drugs in raft formation are missing. In the present study, the influence of trifluoperazine, thioridazine and chlorpromazine on domain organization in raft-mimicking model membranes was investigated. Using giant unilamellar vesicles formed from an equimolar DOPC:sphingomyelin:cholesterol mixture, we found that phenothiazines elevated the number of domains, decreased their area and markedly increased the total length of the domain border. The impact of studied drugs on phase separation in the raft lipid mixture was also confirmed by Laurdan generalized polarization measurements. Alteration of domain organization induced by antipsychotic drugs was very likely to arise from selective accumulation of phenothiazines in interfacial regions between liquid ordered and liquid disordered domains. Interpretation of the results allowed us to demonstrate new aspects underlaying mechanisms of action of phenothiazine-type antipsychotic drugs. To the best of our knowledge, this is the first report demonstrating the influence of drugs on domain morphology directly visualized in giant unilamellar vesicles.
Introduction
The idea of lipid rafts was first introduced on the basis of observation that some biological membrane fragments were resistant to solubilization by cold, non-ionic detergents (like Triton X-100). Nowadays, rafts are defined as dynamic nanoscale cholesterol and sphingolipid-enriched membrane domains that laterally segregate from the bulk membrane and can provide a platform for certain membrane proteins (for a recent review, see Lingwood and Simons Citation2010). Rafts are believed to constitute the dynamic assemblies within the bilayer, engaged in such processes as signal transduction, membrane trafficking and cell polarization. These membrane domain structures have been hypothesized to be platforms assembling different classes of signal complexes (Simons and Toomre Citation2000). The role of membrane sub-domains, such as caveolae and lipids rafts, is highly relevant to the biology of cancer (Freeman et al. Citation2005, Arcaro et al. Citation2007). For example, it has been shown that signal transduction in prostate cancer cells may be dependent on the cholesterol content in rafts (Zhuang et al. Citation2005). Since rafts were revealed by Simons and Ikonen (Citation1997) and Brown and London (Citation1998) as important functional membrane components, they have attracted the attention of many laboratories. Apart from the characterization of composition and function of rafts occurring in biological membranes, numerous studies were also devoted to the problem of their origin, i.e., phase separation phenomenon and formation mechanisms.
Domains observed in different lipid systems and/or formed under different experimental conditions displayed a variety of shapes as well as different formation dynamics (Bagatolli Citation2006). Although many aspects of domain existence and formation have been extensively studied using both theoretical and experimental methods, we are far from the full understanding of these phenomena. For example, two dissimilar types of the domain growth dynamics were observed by Yanagisawa et al. (Citation2007) in ternary DPPC:DOPC:Chol mixtures – normal coarsening and trapped coarsening. It is worth emphasizing that both types of coarsening were observed in the same sample. The problem of domain formation and triggering between normal and trapped coarsening processes was also studied theoretically using models based on different approaches. Some authors considered the elastic membrane properties (Semrau et al. Citation2009, Ursell et al. Citation2009) while others used entropy-based approach (Pata and Dan Citation2005; Dean and Manghi Citation2006). These models, however, usually possessed serious limitations and they mostly did not allow us to analyze any electric interactions, particularly dipolar, in the studied systems.
Although different aspects of domain formation in binary or ternary lipid model systems have been described in many works, only a limited number of papers is devoted to the problem of the influence of different additives (such as anesthetics, drugs or other impurities) on this process. One example is the paper by Soderlund et al. (Citation1999) describing the influence of cyclosporin A on DPPC and DPPC:Chol monolayers. The presence of foreign molecules might be very important for the raft formation and stability. Veatch et al. (Citation2007) have demonstrated that lipid miscibility, a crucial factor for raft formation, can be significantly affected even by trace amounts of fluorescent probes, which usually are treated as non-disturbing agents. Jorgensen and co-workers (Citation1991) developed a model of a single-component membrane in which two phases (gel and fluid) could co-exist in the temperatures near the lipid main phase transition temperature. Using this model, they showed that addition of foreign molecules (anesthetics and/or cholesterol) alters the process of domain formation in membranes (Jorgensen et al. Citation1993). The presence of anesthetics decreased the average domain size and the foreign molecules showed a tendency to accumulate in the domain interface between gel and liquid-crystalline phases (Mouritsen and Jorgensen Citation1994). Cholesterol added in low concentration (<10 mol %) stabilized the domains and excluded the anesthetic molecules from the interfacial regions. The possibility of the trapping of small particles in the domain border region was also theoretically confirmed by Ruckerl et al. (Citation2008). Using Monte Carlo simulations, they have shown that due to the interactions between the dipole moments of nanoparticles and dipole moments of domains the diffusion of small molecules becomes restricted to the domain border region. The time during which molecules are trapped depends on the domain size – small domains are more ‘sticky’ and keep small molecules longer in their vicinity.
Since more than 50 years phenothiazine derivatives, trifluoperazine (TFP), thioridazine (TDZ) and chlorpromazine (CPZ) have been widely used in the therapy of mental disorders such as schizophrenia (Marques et al. Citation2004, Fenton et al. Citation2007, Leucht et al. Citation2008). Almost 10 years ago, we published a paper in which we demonstrated that a well known antipsychotic agent – trifluoperazine – induced phase separation in bilayers formed from synthetic phospholipids (Hendrich et al. Citation2001). In the subsequent article (Hendrich et al. Citation2007), we additionally showed that phenothiazine derivatives influenced phase separation also in lipid systems containing low amounts of cholesterol and sphingomyelin. In the previous projects, using microcalorimetry and fluorescence spectroscopy as experimental methods, we were not able to directly visualize lipid domains. The present study on the influence of TFP and two other phenothiazines (thioridazine and chlorpromazine) on the organization of domains in raft-mimicking model membranes is a natural consequence of our previous interests combined with the application of giant unilamellar vesicle (GUV) technique that was recently introduced to our laboratory (Wesolowska et al. Citation2009). The equimolar mixture of unsaturated phospholipid (DOPC), cholesterol (Chol) and sphingomyelin (SM) has been chosen because it is a widely accepted model system mimicking lipid rafts of biological membranes. Interpretation of the new data together with some conclusions drawn previously (Hendrich et al. Citation2001), allowed us to demonstrate new aspects underlaying the mechanisms of action of phenothiazine-type antipsychotic drugs. To the best of our knowledge, this is the first report demonstrating the influence of low-molecular weight additives on domain morphology directly visualized in giant unilamellar vesicles.
Materials and methods
18:1 (Δ9-Cis) 1,2-dioleoyl-sn-glycero-3-phosphocholine (DOPC), 18:0 porcine brain sphingomyelin and cholesterol were purchased from Avanti Polar Lipids (Alabaster, AL, USA). Lipids were used without further purification. Trifluoperazine and thioridazine were from ICN Biomedicals Inc. (Costa Mesa, CA, USA). Chlorpromazine was bought from Sigma (Poznan, Poland). Laurdan and 1,1′-didodecyl-3,3,3′,3′-tetramethylindocarbocyanine perchlorate (DiIC12(3)) were from Molecular Probes, Inc. (Eugene, OR, USA). The solvents (ethanol, chloroform, DMSO) were of analytical grade.
Laurdan generalized polarization
Chloroform solutions of DOPC, Chol and SM were mixed (molar ratio 1:1:1), dried under stream of nitrogen and kept under vacuum for at least 2 h to remove traces of organic solvent. Next, the lipid film was hydrated with 20 mM Tris buffer containing 150 mM NaCl and 0.5 mM EDTA (pH 7.4), heated to approximately 50°C and vortexed. Multilamellar liposomes were extruded seven times through 400 nm nucleopore polycarbonate filter (Whatman Millipore, USA).
Liposomes (final lipid concentration 200 μM) were incubated with Laurdan (final concentration 5 μM) for 15 min in the dark at room temperature. Then TFP was added and the incubation was continued for further 30 min. Laurdan was dissolved in DMSO, and TFP was dissolved in water. Steady-state fluorescence emission spectra were recorded by LS 50B spectrofluorimeter (Perkin-Elmer Ltd, Beaconsfield, Buckinghamshire, UK) equipped with a xenon lamp, with excitation and emission slits of 5 nm. Excitation wavelength for Laurdan ranged from 320–390 nm. Spectroscopic measurements were performed at room temperature. Data were processed with FLDM Perkin-Elmer software. Laurdan generalized polarization (GP) was calculated according to the equation given by Parasassi et al. (Citation1990):
where IB = fluorescence intensity at the blue region of Laurdan emission spectrum (440 nm), and IR = fluorescence intensity at the red region of Laurdan emission spectrum (490 nm). It was checked that DMSO in the concentration used did not influence Laurdan GP.
Preparation of GUVs
Giant unilamellar vesicles were prepared by electroformation method, first introduced by Angelova and Dimitrov in Citation1986 (Dimitrov and Angelova Citation1988), using a self-designed chamber described previously (Wesolowska et al. Citation2009). Stock solutions of lipids (0.6 mM) and DiIC12(3) (0.3 mM) were prepared in chloroform, whereas phenothiazine derivatives (5 mM) were dissolved in ethanol. Equimolar DOPC:SM:Chol mixture was prepared with addition of the fluorescent probe (DiIC12(3) at final concentration 0.1 mol %) and – in the case of modified samples – an appropriate amount of phenothiazine derivative solution. Drops of 1 μl of the pre-mixed solution were applied onto platinum wire electrodes built in a Teflon cylindrical chamber with a transparent bottom (a coverslip). The electrodes were then dried under a stream of nitrogen and the whole chamber was kept under vacuum for at least 1 h to remove traces of chloroform. Next, the lipid sample was hydrated with approximately 1 ml of high purity water; the chamber was closed and mounted at a microscope stage. GUVs were produced by an application of sinusoidal 10 Hz wave of the amplitude of 3 V (peak-to-peak) for 60 min at 50°C. The temperature of the chamber was controlled with 0.2°C accuracy by a thermocouple built in the wall of the chamber, close to the electrodes. After formation of GUVs, the voltage was turned off and the chamber was slowly cooled down using a heat block connected to a microscope stage. Microscopic observations were performed at room temperature after ca. 60 min after the formation of GUVs. Such experimental conditions allowed the system to reach the state in which both GUVs and domain sizes were stable in time. The obtained vesicles had spherical shapes and diameters ranging from 10–45 μm.
Image acquisition and analysis
Images were collected using a Nikon D-Eclipse C1 confocal system (Nikon Instruments, Amstelveen, The Netherlands) equipped with an argon 488 nm laser. It was configured with a Nikon Eclipse TE2000-E microscope, equipped with a PlanFluor 40× (0.60) air objective. Fluorescent light emitted by the samples was collected in the range 565–605 nm. Pinhole was set to 60 μm. Each slice was scanned three times and an average picture was further analyzed. EZ-C1 and NIS ELEMENTS C AR software (Nikon) was used for image acquisition and analysis. For each lipid:modifier mixture, at least two independent preparations were made. A minimum of 50 individual vesicles were analyzed within each preparation. Calculation of domain areas was performed from sequential z-scans according to the method described by Juhasz et al. (Citation2009). Domain border length GUV was calculated as a total perimeter of all domains observed on the surface of a given vesicle. The Mann-Whitney U-test was used to compare parameters obtained for the control samples and the samples modified with phenothiazine derivatives. The results were accepted to be significant if p < 0.05.
Molecular modelling
Theoretical calculations were performed using Titan 1.0.8 software (Wavefunction, Inc., Irvine, USA & Schrodinger, Inc., Portland, OR, USA). The properties of studied phenothiazine derivatives were modeled using AM1 semi-empirical molecular orbital method.
Results
Influence of phenothiazine derivatives on Laurdan generalized polarization
The analysis of Laurdan generalized polarization (GP) in liposomes formed from an equimolar DOPC:SM:Chol mixture was performed in reference to our previous studies in DPPC, and DPPC mixtures with low amounts of cholesterol and sphingomyelin (Hendrich et al. Citation2001, Citation2007). The measurement of Laurdan GP as a function of excitation wavelength (λex) is a recognized method for bilayer phase state determination and also for demonstration of the existence of microdomains in model membrane (Parasassi and Gratton Citation1995, Bagatolli et al. Citation1999). Shortly, the line mirroring GP dependence on λex is horizontal in the gel phase, descending in liquid-crystalline and liquid-ordered phase, and ascending when separate domains of different phases coexist (Mukherjee and Chattopadhyay Citation2005, Baumgart et al. Citation2007). As shown in , Laurdan GP remained constant with the rise of excitation wavelength in case of DOPC:SM:Chol (1:1:1) model system studied at room temperature. Such a shape of GP(λex) function was observed in temperature range 20–30°C, while in temperatures from 35–55°C the function was descending (). As the temperature was raised, Laurdan GP values recorded in DOPC:SM:Chol liposomes evolved from ca. 0.6 at 20°C to ca. 0.2 at 55°C. The influence of phenothiazine derivative on Laurdan generalized polarization was also monitored. As can be seen in , the addition of TFP to the liposomes reduced GP values and caused the line mirroring GP dependence on λex to become ascending. The effect was more pronounced in higher TFP concentrations. The shape of GP(λex) function remained ascending in temperatures from 20–55°C. Two other compounds, TDZ and CPZ, exerted qualitatively similar effects (data not shown), however significantly less pronounced than in case of TFP.
Figure 1. The effect of TFP on the Laurdan generalized polarization dependence on the excitation wavelength in DOPC:SM:Chol (1:1:1) liposomes. Symbols represent: – pure lipid,
– lipid + 50 μM of TFP, Δ – lipid + 100 μM of TFP,
– lipid + 200 μM of TFP. Measurements were performed at 25°C (A) and 50°C (B).
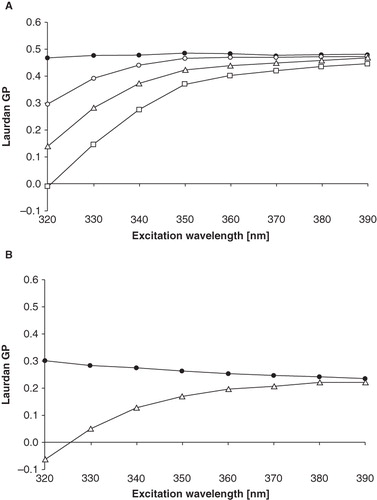
Influence of phenothiazine derivatives on lipid domains
Each preparation of giant unilamellar vesicles from DOPC:SM:Chol (1:1:1) mixture yielded from several tens to more than a hundred of spherical vesicles whose diameters ranged from 10–45 μm. Carbocyanine fluorescent probe DiIC12(3) that possesses 12-carbon-long hydrophobic tail was used for labeling of GUVs. This fluorescent probe is known to partition preferentially into liquid disordered (Ld) domains in the DOPC:sphingomyelin:cholesterol system (Mouritsen and Jorgensen Citation1992). It was therefore assumed that the bright regions visible on the surface of GUVs corresponded to the liquid disordered phase, whereas the dark regions corresponded to the liquid ordered (Lo) phase.
The inspection of the GUVs formed from the equimolar DOPC:SM:Chol mixture revealed vesicles with a few, relatively big, round, bright-fluorescent regions (). The addition of phenothiazine derivatives to the lipid mixture from which liposomes were formed dramatically influenced the domain pattern observed on the surface of GUVs. present typical images recorded in the presence of 200 μM of trifluoperazine, the drug that exerted the most pronounced effect. Generally, the large increase in domain number was observed accompanied by their surface area reduction; although the domains remained round. It should be stressed that TFP affected individual vesicles to a different extent what resulted in a highly heterogeneous population of GUVs, whose representatives are illustrated.
Figure 2. Representative confocal images (hemispherical projections) of GUVs formed from equimolar DOPC:SM:Chol mixture (A), and GUVs modified with 200 μM of TFP (B-D). GUVs were labelled with DiIC12(3) (probe concentration 0.1 mol %). Scale bar is 10 μm. Images were recorded at room temperature.
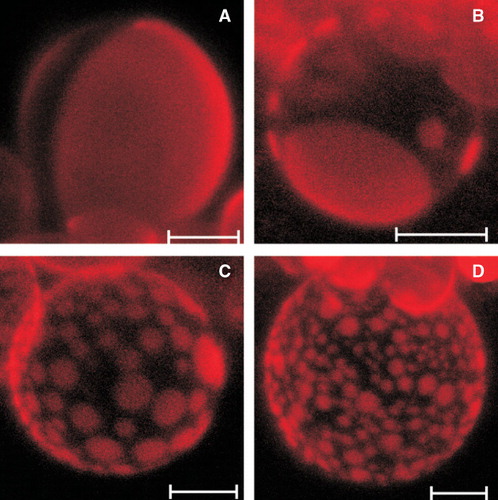
The domains observed on the surface on individual GUVs were counted and their diameter was measured both in preparations made of pure lipid mixture and lipid modified with phenothiazine derivatives. Since the population of GUVs was quite heterogeneous, not only in drug-modified samples but also in pure lipid ones, we decided to analyze the data in terms of median rather than mean values. Moreover, the inspection of the data revealed that they were not distributed normally. presents the number of domains recorded on the surface of GUVs for vesicles prepared from DOPC:SM:Chol (1:1:1) without and in the presence of the studied drugs. Median values and 25–75% ranges are shown for each sample. To provide the reader with a notion about the heterogeneity within the population of GUVs, it should be mentioned that the lowest number of domains observed on a single vesicle was 1 and the highest was 7 in case of pure lipid mixture (the least heterogeneous sample), while for lipid modified with TFP at 200 μM concentration (the most heterogeneous sample) the minimal value was 5 and the maximal one – 139. As can be seen in , in the presence of phenothiazine derivatives the number of domains per GUV increased as compared with pure DOPC:SM:Chol mixture. The most dramatic increase was induced by TFP, followed by TDZ and CPZ that was the weakest active compound in this respect.
Figure 3. The number of domains observed in GUVs formed from DOPC:SM:Chol (1:1:1) under control conditions, modified with 100 μM of TFP, 200 μM of TFP, 200 μM of TDZ, and 200 μM of CPZ (from left to right, respectively). Median values (squares) and 25–75% values (boxes) are shown. An asterisk marks the results significantly different from the control (p < 0.05).
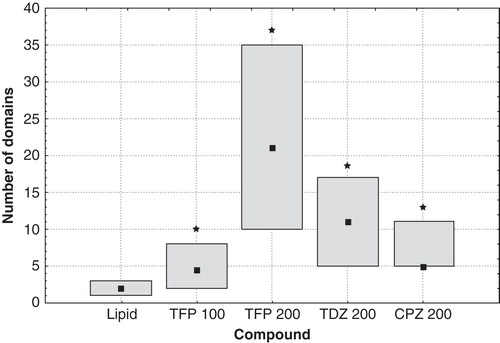
The additional effect of phenothiazine derivatives on domain pattern observed in giant unilamellar vesicles composed of the equimolar DOPC:SM:Chol mixture was the substantial reduction of the surface area of individual domains (). The median value was reduced from 27.6 μm2 in pure lipid, to 13 μm2 in samples modified with 100 μM of TFP, 2.3 μm2 in samples containing 200 μM of either TFP or CPZ, and 1.3 μm2 for 200 μM of TDZ. Moreover, the decrease of individual domain size was accompanied by the reduction of the total area occupied by the Ld domains (data not shown).
Figure 4. Domain's area in GUVs formed from DOPC:SM:Chol (1:1:1) under control conditions, modified with 100 μM of TFP, 200 μM of TFP, 200 μM of TDZ, and 200 μM of CPZ (from left to right, respectively). Median values (squares) and 25–75% values (boxes) are shown. An asterisk marks the results significantly different from the control (p < 0.05).
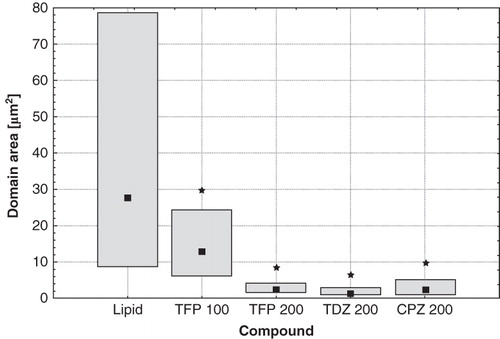
The total domain border length per GUV was also calculated to estimate the size of the interfacial region in pure lipid and drug-modified samples (). Trifluoperazine in both concentrations tested (100 μM and 200 μM) caused a significant increase of this parameter in comparison with pure DOPC:SM:Chol mixture. For CPZ and TDZ such an effect was not observed or was insignificant.
Figure 5. The total length of domains' border recorded in GUVs formed from DOPC:SM:Chol (1:1:1) under control conditions, modified with 100 μM of TFP, 200 μM of TFP, 200 μM of TDZ, and 200 μM of CPZ (from left to right, respectively). Median values (squares) and 25–75% values (boxes) are shown. An asterisk marks the results significantly different from the control (p < 0.05).
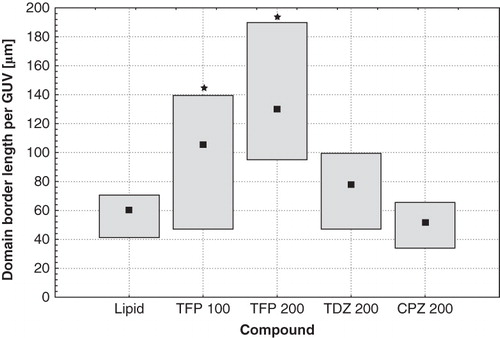
Molecular modelling
Molecular calculations were performed to obtain the values of some molecular descriptors of the studied phenothiazine-type drugs. It was found that the octanol:water partition coefficient values (logP) were 3.61, 3.73 and 4.22 for CPZ, TFP and TDZ, respectively. Dipole moments of phenothiazine derivatives were also calculated. Trifluoperazine was characterized by the highest dipole moment (5.12 D), while this parameter was significantly lower for thioridazine and chlorpromazine (2.84 D and 2.52 D, respectively). The dipole moment vector was found to be spatially oriented in a similar way (in relation to a heterocyclic moiety of molecule) for all the studied drugs.
Discussion
An increasing number of publications in recent years support the hypothesis that one of the major functions of lipid rafts is their role as the platforms collecting proteins involved in certain membrane processes (Lingwood and Simons Citation2010). This particular raft function, even after a brief inspection, reveals at least two separate aspects: grouping of proteins in a certain area and providing a proper surrounding for their activity. To achieve the first of the above goals, rafts cannot be too small and their lipid composition must ‘attract’ proteins, otherwise their capacity to hold the proper set of proteins would be insufficient. Thus, it seems obvious that factors which affect the domain size may also affect the raft functioning. On the other hand, membrane proteins are sensitive to alteration of lipid matrix biophysical properties (Mouritsen and Jorgensen Citation1992). Therefore, any agent that interacts with raft lipids could potentially also affect the activity of raft proteins. For the reasons presented above, it seems to be of the utmost importance to study how membrane domain properties can be altered by compounds such as anesthetics, antipsychotic drugs and other membrane-active substances.
It is commonly accepted that domain formation in lipid membranes composed of not fully miscible components starts with the nucleation followed by the domains' growth and membrane coarsening. However, as found by Yanagisawa et al. (Citation2007), two different scenarios are possible for the coarsening process. In the normal type of coarsening, the domains grow in a diffusion-and-coalescence manner according to the phenomenological power law providing the relation between domain diameter (d) and formation time (d ∼ t2/3). During the trapped coarsening, the domain size growth is suppressed by the repulsive interactions between the domains. As suggested by Yanagisawa et al. (Citation2007), this domain repulsion and trapping might be caused by the coupling between phase separation and membrane elasticity under the incompressibility constraint.
The physical background of raft formation lies in immiscibility of some membrane components, which in certain circumstances tend to stay in separate domains differing in the phase state. The basic mechanism for phase separation could be, however, very easily perturbed by even trace quantities of compounds that intercalate into lipid bilayer and thus influence polar and/or hydrophobic regions of lipid bilayers. The poor stability of SM-rich domains in membranes formed from the PC:SM:Chol mixture was predicted by Heerklotz et al. (Citation2003) in the study on the influence of low concentrations of Triton X-100 on the domain formation process. Analysis of thermodynamical data obtained by isothermal titration calorimetry has led to the conclusion that small perturbation (i.e., its energy is not exceeding 0.2 kJ/mol) might induce substantial domain formation in the previously homogeneous membranes. Another unexpected and striking example of domain formation perturbing agents is fluorescent probes, though their usage in lipid membrane research is based on the general assumption that they do not affect the studied systems. Veatch et al. (Citation2007) demonstrated that two fluorescent probes: DiIC12 and DiOC18 (usually treated as non-perturbing at 0.5 mol %, as used in this work), might significantly alter the miscibility of lipids in ternary DOPC:DPPC:Chol systems. The observed effects were explained in terms of the modulation of interfacial energy between coexisting phases by impurities.
As reported in our previous papers (Hendrich et al. Citation2001, Citation2007), phenothiazine-type antipsychotic drugs interfere with the phase separation process both in pure synthetic DPPC or DMPC as well as in ternary DOPC:SM:Chol mixtures. Although these studies have shown new features of TFP, TDZ and CPZ as membrane active compounds, two disadvantages should be mentioned: the applied model systems poorly mimicked the biological membranes and no direct insight into domain morphology was possible. In the present study we used GUVs formed from the so-called canonical ‘lipid raft mixture’ – equimolar DOPC:SM:Chol. This model, though still artificial, better represents the circumstances existing in biological membranes.
Unique environment-dependant spectral properties of Laurdan were employed to study the phase state of the raft mimicking mixture. For pure DOPC:SM:Chol (1:1:1) liposomes GP was independent on excitation wavelength in temperatures 20–30°C, and in higher temperatures GP(λex) function was descending. Such a picture is typical for phosphatidylcholine membranes with high cholesterol content (Parasassi et al. Citation1994) and was also observed for biological membranes studied with the use of Laurdan (Mukherjee and Chattopadhyay Citation2005, Roche et al. Citation2008). It should be born in mind that Lo and Ld phases present in model raft-like systems and biological membranes are both liquid, however distinct phases. The rate of dipolar relaxation processes is comparable in both phases (Bagatolli et al. Citation1999, Mukherjee and Chattopadhyay Citation2005) and therefore their properties are not dissimilar enough to allow for the detection of phase separation with the use of Laurdan in spectrofluorimetric studies. Only under certain conditions, if the properties of the phases are differentiated enough by some external factor, Laurdan could show the co-existence of separated domains. For example, in temperatures as low as 4°C, ascending Laurdan GP (λex) function was recorded in natural membranes (Roche et al. Citation2008). A similar observation was made by Dietrich et al. (Citation2001), who recorded the existence of Laurdan-detectable domains in GUVs composed of the equimolar DOPC:SM:Chol mixture only in low temperatures, and their disappearance in temperatures above 25°C. The introduction of trifluoperazine into the model lipid system studied in the present work resulted in GP gradual increase with the rise of excitation wavelength. It was therefore concluded that TFP induced phase separation in DOPC:SM:Chol liposomes in a concentration-dependant manner in temperatures between 20°C and 55°C. A similar but much weaker effect was also recorded for TDZ and CPZ. This was likely to be a result of the stronger differentiation between Lo and Ld properties caused by phenothiazine derivatives in the raft-mimicking model membrane as compared to the non-modified lipid system.
In our present study, the impact of phenothiazine drugs on the organization of lipid domains was visualized by confocal microscopy with the application of DiIC12(3) fluorescent probe in GUVs used as model membranes. In vesicles formed from ternary DOPC:SM:Chol mixture comparatively large Ld domains (bright areas) coexisted with Lo domains (dark areas). Although DilC12(3) at 0.5 mol % concentration was previously reported by Veatch et al. (Citation2007) to affect lipid miscibility in the ternary DOPC:DPPC:Chol system, this putative problem was minimized in the present work by using a five-times lower concentration of fluorescent probe. Additionally, the same amount of the probe was used in all experiments and even if it introduced any changes to the studied system, the pure lipid samples and mixtures containing drugs were affected identically. The presence of trifluoperazine at 200 μM concentration induced significant changes of the domain size and number. CPZ and TDZ induced qualitatively similar changes but they were less pronounced comparing to the extent of domain alteration induced by TFP.
Quantitative analysis of GUV experiments was also performed. First we noticed that all used phenothiazine derivatives increased the number of domains in examined vesicles. In the case of TFP, this increase seemed to be also dose-dependent because the drug at 100 μM concentration elevated the number of domains much less effectively than at 200 μM. Comparing the effects exerted on the number of domains by the same (200 μM) concentration of the examined drugs one can conclude that their efficacy follows the order of TFP > TDZ > CPZ. It should be stressed that the overall effect of TFP was dominant. Also, the area occupied by individual Ld domains was affected by the studied drugs. In the case of this parameter, the effects exerted by all the studied compounds were comparable – the median value of the domain area was decreased approximately 10 times, when compared to domain sizes recorded for GUVs without the addition of drugs. For the purpose of discussion presented below, it is worth emphasizing that domain areas were calculated by the method enabling for the correction of the effects caused by the projection of spherical domains onto the two-dimensional image.
Chlorpromazine was previously reported to increase the number of domains with the concomitant reduction of their size in monolayers formed from the mixture of DPPC with negatively charged brain phosphatidylserine (Jutila et al. Citation2001). The authors attributed the observed effects to the preferential interaction of CPZ with acidic phospholipid that resulted in an increased gel-liquid domain boundary length.
The propensity of certain drugs to interact preferentially with lipid rafts was shown by Eisensamer et al. (Citation2005). They reported that antidepressant (desipramine, fluoxetine, reboxetine) and antipsychotic (fluphenazine, haloperidol, clozapine) drugs were markedly enriched in raft fractions isolated from HEK293 cells stably transfected with the 5-HT3A serotonin receptor. Additionally, the concentrations of drugs within these fractions correlated with their ability to inhibit the cationic currents induced by serotonin. Taking into account the results of our experiments presented above, we may conclude that also phenothiazine derivatives interact with lipid rafts and perturb their properties. The mechanism of this perturbation is, in our opinion, based on the influence of these compounds on the Lo/Ld phase separation and domain formation. It is generally accepted that the crucial role in this process is played by the specific interactions of cholesterol with the ‘higher melting’ component of ternary lipid mixture (Wenz and Barrantes Citation2003, Simons and Vaz Citation2004, Lingwood and Simons Citation2010). In most cases, this ‘higher melting’ component is sphingomyelin or phospholipid possessing fully saturated acyl chains. Phase separation occurs because at room or at physiological temperature, cholesterol – due to the rigid structure of its molecule – fits better to the less fluid, saturated chains than to the kinked and more flexible unsaturated chains of ‘lower melting’ lipid component. Despite this molecular fitting, domains (rafts) can exist only when the cholesterol content in the system does not exceed certain limits; moreover even within these limits, the size of domains depends on the sterol concentration (de Almeida et al. Citation2005). Furthermore, analyzing the role of cholesterol in heterogeneous systems, it is also important to notice that lateral distribution of this compound could be non-uniform. For example, Cruzeiro-Hansson et al. (Citation1989) have theoretically demonstrated that cholesterol might accumulate in the domain interfacial region. Further modification of this model, presented by Jorgensen et al. (Citation1993), allowed them to study the influence of foreign molecules (like anesthetics) on the domain formation process. The calculations revealed that anesthetics had high affinity to domain interfaces and they might compete with cholesterol molecules for localization in these regions. Accumulation of interfacially active molecules additionally increases the length of interfaces. Molecules called in Jorgensen's model ‘anesthetics’ were purely theoretical foreign molecules possessing certain properties and in fact they could be replaced by any other real molecules sharing the most essential features of the model ones. Phenothiazine derivatives fulfill the conditions to be regarded as such interfacially active molecules.
The drug-cholesterol competition mentioned above was experimentally demonstrated by Takegami et al. (Citation1999) for CPZ and TFP. It was shown that increasing amounts of cholesterol in liposome membranes effectively decreased the lipid/water partition coefficient of these drugs. Effects exerted by phenothiazine derivatives on lipid bilayers were similar to those of cholesterol. Several years ago we showed that 2-trifluoromethyl-10-(4-[methylsulfonylamid]buthyl)-phenothiazine affects the DPPC bilayer fluidity in a cholesterol-like manner (Hendrich et al. Citation2002). In temperatures below the main phase transition it caused membrane fluidization, while above the transition temperature, it decreased bilayer fluidity. CPZ was shown to affect the fluidity of DMPC bilayers in a similar way. The decrease of both fluorescence anisotropy of DPH and general polarization of Laurdan at 18°C and the increase of the same parameters at 37°C with the rise of CPZ concentration was observed by Suwalsky et al. (Citation2008). Studies carried out with electron spin resonance technique (Wisniewska and Wolnicka-Glubisz Citation2004) indicated that the effect of CPZ on lipid order and mobility in phosphatidylcholine liposomes depended on physical state of membrane and the presence of cholesterol. Similarly as in studies by Takegami et al. (Citation1999) cholesterol reduced the partition of the drug into lipid bilayer.
On the other hand, X-ray diffraction technique was used to demonstrate that some antipsychotic drugs (including CPZ) altered the structure of both Lo and Ld phases in bilayers formed from ternary PC:SM:Chol mixture (Tessier et al. Citation2008). The observed reduction of Lo bilayer thickness in the presence of CPZ was attributed to the decreased number of SM-cholesterol complexes in this phase as a consequence of a competition between cholesterol and CPZ. According to the theoretical models the interfacial energy at the domain edge increases with a phase height mismatch (Garcia-Saez et al. Citation2007). Therefore, the alteration of the Lo bilayer thickness induced by phenothiazine derivatives might also affect the interfacial energy. The drug-induced domain thickness alteration might additionally enhance the morphological changes resulting from the presence of phenothiazine molecules in domain interfacial region.
Summarizing all the above considerations, we can conclude that phenothiazine derivatives studied in this work interfered with Lo/Ld phase separation in ternary DOPC:SM:Chol membranes, and TFP occurred to be the most active compound. First of all, according to the results of spectroscopic studies, the drugs (TFP, TDZ and CPZ) enhanced the difference between Lo and Ld phases to such an extent that it affected spectral properties of Laurdan and allowed us to record phase separation with this method. On the other hand, the decrease of the domain area and the increase of the number of domains in GUVs suggest that the presence of phenothiazine derivatives favors the trapped mode of domain coarsening. According to the theoretical considerations presented in the Introduction section (Ruckerl et al. Citation2008) and above (Jorgensen et al. Citation1993) it is possible that phenothiazine-type molecules could accumulate in the domain interfacial region. This hypothesis seems to be supported by the fact that the studied drugs effectively increased the total length of domain borders. In the case of TFP, which was again the most active in this aspect, the effect was significant (200 μM of this drug doubled the total domain border length) and dose-dependent. The influences of TDZ and CPZ were statistically insignificant (with respect to the pure raft mixture).
In order to elucidate which molecular property of phenothiazine derivatives could be responsible for the strength of their influence on domain morphology, we performed the computer modeling of these molecules. Analysis of the results revealed that out of the whole set of molecular descriptors, only the dipole moments of the studied phenothiazine derivatives followed the same order as recorded in all presented herein experiments (TFP > TDZ > CPZ). The role of drug's dipole moments could be explained using the arguments given by Thirumoorthy et al. (Citation2007). These authors, combining theoretical considerations and monolayer experiments, stressed the role of molecular dipole moments in the process of domain formation. Domain repulsion, playing the main role in the trapped domain coarsening mode, could result at least partially from the dipolar interactions between the domains. Similar reasoning was proposed by Tarahovsky et al. (Citation2008) for plant flavonoids. It was suggested that flavonoids might control membrane heterogeneity by influencing dipole membrane potential. According to the above hypotheses it is likely that phenothiazine derivative molecules accumulating in the interphase region may increase the dipolar repulsion between domains and thus promote their trapped coarsening and that this effect would depend on the values of dipole moments of individual drugs.
Conclusions
In the present study, using GUVs formed from equimolar DOPC:SM:Chol mixtures as model membranes, we have shown that phenothiazine-type antipsychotic drugs alter the organization of lipid domains in this system. Such an activity is very likely to arise from the selective phenothiazines' accumulation in the interfacial regions between Lo and Ld domains. Such features of TFP, TDZ and CPZ, reported here for the first time, shed a new light on the possible mechanisms of pharmacological activity of these drugs. This reasoning can also be extended to other substances. Namely, the modulation of lipid domains' structure and organization could putatively form a basis for the activity of any other drug affecting either membrane lipid components or raft-associated proteins.
Declaration of interest: This work was supported by the Polish Committee for Scientific Research, funds for the Wrocław Medical University (Department of Biophysics). The authors report no conflicts of interest. The authors alone are responsible for the content and writing of the paper.
References
- de Almeida RFM, Loura LMS, Fedorov A, Prieto M. 2005. Lipid rafts have different sizes depending on membrane composition: A time-resolved fluorescence resonance energy transfer study. J Mol Biol 346:1109–1120.
- Angelova MI, Dimitrov DS. 1986. Liposome electroformation. Faraday Discuss Chem Soc 81:303–308.
- Arcaro A, Aubert M, Espinosa del Hierro ME, Khanzada UK, Angelidou S, Tetley TD, Bittermann AG, Frame MC, Seckl MJ. 2007. Critical role for lipid raft-associated Src kinases in activation of PI3K-Akt signaling. Cell Signal 19:1081–1092.
- Bagatolli LA, Parasassi T, Fidelio GD, Gratton E. 1999. A model for the interaction of 6-lauroyl-2-(N,N-dimethylamino)naphthalene with lipid environments: Implications for spectral properties. Photochem Photobiol 70:557–564.
- Bagatolli LA. 2006. To see or not to see: Lateral organization of biological membranes and fluorescence microscopy. Biochim Biophys Acta 1758:1541–1556.
- Baumgart T, Hunt G, Farkas ER, Webb WW, Feigenson GW. 2007. Fluorescence probe partitioning between Lo/Ld phases in lipid membranes. Biochim Biophys Acta 1768:2182–2194.
- Brown DA, London E. 1998. Structure and origin of ordered lipid domains in biological membranes. J Membr Biol 164:103–114.
- Cruzeiro-Hansson L, Ibsen JH, Mouritsen OG. 1989. Intrinsic molecules in lipid membranes change the lipid-domain interfacial area: Cholesterol at domain interfaces. Biochim Biophys Acta 979:166–176.
- Dean DS, Manghi M. 2006. Fluctuation-induced interactions between domains in membranes. Phys Rev E 74:021916.
- Dietrich C, Bagatolli LA, Volovyk ZN, Thompson NL, Levi M, Jacobson K, Gratton E. 2001. Lipid rafts reconstituted in model membranes. Biophys J 80:1417–1428.
- Dimitrov DS, Angelova MI. 1988. Lipid swelling and liposome formation mediated by electric fields. Bioelectrochem Bioenerg 19:323–333.
- Eisensamer B, Uhr M, Meyr S, Gimpl G, Deiml T, Rammes G, Lambert JJ, Zieglgansberger W, Holsboer F, Rupprecht R. 2005. Antidepressants and antipsychotic drugs colocalize with 5-HT3 receptors in raft-like domains. J Neurosci 25:10198–10206.
- Fenton M, Rathbone J, Reilly J, Sultana A. 2007. Thioridazine for schizophrenia. Cochrane Database Syst Rev 3:CD001944.
- Freeman MR, Cinar B, Lu ML. 2005. Membrane rafts as potential sites of nongenomic hormonal signaling in prostate cancer. Trends Endocrinol Metab 16:273–279.
- Garcia-Saez AJ, Chiantia S, Schwille P. 2007. Effect of line tension on the lateral organization of lipid membranes. J Biol Chem 282:33537–33544.
- Heerklotz H, Szadkowska H, Anderson T, Seelig J. 2003. The sensitivity of lipid domains to small perturbations demonstrated by the effect of Triton. J Mol Biol 329:793–799.
- Hendrich AB, Wesolowska O, Michalak K. 2001. Trifluoperazine induces domain formation in zwitterionic phosphatidylcholine but not in charged phosphatidylglycerol bilayers. Biochim Biophys Acta 1510:414–425.
- Hendrich AB, Wesolowska O, Komorowska M, Motohashi N, Michalak K. 2002. The alterations of lipid bilayer fluidity induced by newly synthesized phenothiazine derivative. Biophys Chem 98:275–285.
- Hendrich AB, Michalak K, Wesolowska O. 2007. Phase separation is induced by phenothiazine derivatives in phospholipid/sphingomyelin/cholesterol mixtures containing low levels of cholesterol and sphingomyelin. Biophys Chem 130:32–40.
- Jorgensen K, Ipsen JH, Mouritsen OG, Bennett D, Zuckermann MJ. 1991. A general model for the interaction of foreign molecules with lipid membranes: Drugs and anaesthetics. Biochim Biophys Acta 1062:227–238.
- Jorgensen K, Ipsen JH, Mouritsen OG, Bennett D, Zuckermann MJ. 1993. The effect of anaesthetics on the dynamic heterogeneity of lipid membranes. Chem Phys Lipids 65:205–216.
- Juhasz J, Sharom FJ, Davis JH. 2009. Quantitative characterization of coexisting phases in DOPC/DPPC/cholesterol mixtures: Comparing confocal fluorescence microscopy and deuterium nuclear magnetic resonance. Biochim Biophys Acta 1788:2541–2552.
- Jutila A, Soderlund T, Pakkanen AL, Huttunen M, Kinnunen PK. 2001. Comparison of the effects of clozapine, chlorpromazine, and haloperidol on membrane lateral heterogeneity. Chem Phys Lipids 112:151–163.
- Leucht C, Kitzmantel M, Chua L, Kane J, Leucht S. 2008. Haloperidol versus chlorpromazine for schizophrenia. Cochrane Database Syst Rev 1:CD004278.
- Lingwood D, Simons K. 2010. Lipid rafts as a membrane-organizing principle. Science 327:46–50.
- Marques LO, Lima MS, Soares BG. 2004. Trifluoperazine for schizophrenia. Cochrane Database Syst Rev 1:CD003545.
- Mouritsen OG, Jorgensen K. 1992. Dynamic lipid-bilayer heterogeneity: A mesoscopic vehicle for membrane function? BioEssays 14:129–136.
- Mouritsen OG, Jorgensen K. 1994. Dynamic order and disorder in lipid bilayer. Chem Phys Lipids 73:3–25.
- Mukherjee S, Chattopadhyay A. 2005. Monitoring the organization and dynamics of bovine hippocampal membranes utilizing Laurdan generalized polarization. Biochim Biophys Acta 1714:43–55.
- Parasassi T, De Stasio G, d'Ubaldo A, Gratton E. 1990. Phase fluctuation in phospholipid membranes revealed by Laurdan fluorescence. Biophys J 57:1179–1186.
- Parasassi T, Di Stefano M, Loiero M, Ravagnan G, Gratton E. 1994. Influence of cholesterol on phospholipid bilayers phase domains as detected by Laurdan fluorescence. Biophys J 66:120–132.
- Parasassi T, Gratton E. 1995. Membrane lipid domains and dynamics as detected by Laurdan fluorescence. J Fluorescence 5:59–69.
- Pata V, Dan N. 2005. Effect of membrane characteristics on phase separation and domain formation in cholesterol-lipid mixtures. Biophys J 88:916–924.
- Roche Y, Gerbeau-Pissot P, Buhot B, Thomas D, Bonneau L, Gresti J, Mongrand S, Perrier-Cornet JM, Simon-Plas F. 2008. Depletion of phytosterols from the plant plasma membrane provides evidence for disruption of lipid rafts. FASEB J 22:3980–3991.
- Ruckerl F, Kas JA, Selle C. 2008. Diffusion of nanoparticles in monolayers is modulated by domain size. Langmuir 24:3365–3369.
- Semrau S, Idema T, Schmidt T, Storm C. 2009. Membrane-mediated interactions measured using membrane domains. Biophys J 96:4906–4915.
- Simons K, Ikonen E. 1997. Functional rafts in cell membranes. Nature 387:569–572.
- Simons K, Toomre D. 2000. Lipid rafts and signal transduction. Nat Rev Mol Cell Biol 1:31–39.
- Simons K, Vaz WLC. 2004. Model systems, lipid rafts, and cell membranes. Annu Rev Biophys Biomol Struct 33:269–295.
- Soderlund T, Lehtonen JY, Kinnunen PK. 1999. Interactions of cyclosporin A with phospholipid membranes: Effect of cholesterol. Mol Pharmacol 55:32–38.
- Suwalsky M, Villena F, Sotomayor CP, Bolognin S, Zatta P. 2008. Human cells and cell membrane molecular models are affected in vitro by chlorpromazine. Biophys Chem 135:7–13.
- Takegami S, Kitamura K, Kitade T, Hasegawa K, Nishihira A. 1999. Effects of particle size and cholesterol content on the partition coefficients of chlorpromazine and trifluoperazine between phosphatidylcholine-cholesterol bilayers of unilamellar vesicles and water studied by second-derivative spectrophotometry. J Colloid Interf Sci 220:81–87.
- Tarahovsky YS, Muzafarov EN, Kim YA. 2008. Rafts making and rafts braking: How plant flavonoids may control membrane heterogeneity. Mol Cell Biochem 314:65–71.
- Tessier C, Nuss P, Staneva G, Wolf C. 2008. Modification of membrane heterogeneity by antipsychotic drugs: An X-ray diffraction comparative study. J Colloid Interf Sci 320:469–475.
- Thirumoorthy K, Nandi N, Vollhardt D. 2007. Role of dipolar interaction in the mesoscopic domains of phospholipid monolayers: Dipalmitoylphosphatidylcholine and dipalmitoylphosphatidylethanolamine. Langmuir 23:6991–6996.
- Ursell TS, Klug WS, Phillips R. 2009. Morphology and interaction between lipid domains. Proc Natl Acad Sci USA 106:13301–13306.
- Veatch SL, Leung SS, Hancock RE, Thewalt JL. 2007. Fluorescent probes alter miscibility phase boundaries in ternary vesicles. J Phys Chem 111:502–504.
- Wenz JJ, Barrantes FJ. 2003. Steroid structural requirements for stabilizing or disrupting lipid domains. Biochemistry 42:14267–14276.
- Wesolowska O, Michalak K, Maniewska J, Hendrich AB. 2009. Giant unilamellar vesicles – a perfect tool to visualize phase separation and lipid rafts in model systems. Acta Biochim Pol 56:33–39.
- Wisniewska A, Wolnicka-Glubisz A. 2004. ESR studies on the effect of cholesterol on chlorpromazine interaction with saturated and unsaturated liposome membranes. Biophys Chem 11:43–52.
- Yanagisawa M, Imai M, Masui T, Komura S, Ohta T. 2007. Growth dynamics of domains in ternary fluid vesicles. Biophys J 92:115–125.
- Zhuang L, Kim J, Adam RM, Solomon KR, Freeman MR. 2005. Cholesterol targeting alters lipid raft composition and cell survival in prostate cancer cell and xenografts. J Clin Invest 115:959–968.