Abstract
Small GTPases of the Rho family (RhoA, Rac1, and Cdc42) and the Ras family GTPase Rap1 are essential for the assembly and function of epithelial cell-cell junctions. Through their downstream effectors, small GTPases modulate junction formation and stability, primarily by orchestrating the polymerization and contractility of the actomyosin cytoskeleton. The major upstream regulators of small GTPases are guanine nucleotide exchange factors (GEFs) and GTPase activating proteins (GAPs). Several GEFs and a few GAPs have been localized at epithelial junctions, and bind to specific junctional proteins. Thus, junctional proteins can regulate small GTPases at junctions, through their interactions with GEFs and GAPs. Here we review the current knowledge about the mechanisms of regulation of small GTPases by junctional proteins. Understanding these mechanisms will help to clarify at the molecular level how small GTPases control the morphogenesis and physiology of epithelial tissues, and how they are disregulated in disease.
Introduction
Small GTPases are molecular switches that control a broad range of processes in eukaryotic cells. Rho family GTPases, a subset of small GTPases, comprise over 20 isoforms in mammalian organisms, whereas only 5, 10 and 11 isoforms have been described in yeast, Caenorhabditis elegans and Drosophila melanogaster, respectively (Jaffe and Hall Citation2005). This suggests that the evolution of vertebrate species correlates with an increasing complexity of small GTPases. Among the many targets of Rho family GTPases, cell-cell junctions are of critical importance for the morphogenesis of epithelial tissues. Rho family GTPases modulate cell-cell junctions mainly by controlling the assembly and contractility of the actin cytoskeleton, an essential structural scaffold of junctions. Specific proteins recruit the regulators of these GTPases (GEFs and GAPs) to junctions, thereby modulating their function at spatially defined sites. The dynamic interplay between these molecules ultimately orchestrates cell shape, cell motility and tissue morphogenesis.
In this review we focus on the molecular mechanisms that control the activity of Rho family GTPases (RhoA, Rac1, and Cdc42), and the Ras family GTPase Rap1 at vertebrate epithelial cell-cell junctions. This subject is introduced by a brief discussion of junctions, small GTPases, and their regulation. The reader is referred to excellent review articles, for a more comprehensive analysis of these areas.
The epithelial junctional complex
Epithelial cells form single- and multi-layered sheets that cover all body surfaces and cavities, and constitute the parenchyma of glandular organs. A key feature of epithelial cells is the presence, at their apicolateral surfaces, of a specialized junctional complex, which comprises tight junctions (TJ), zonula adherens (also denoted as adherens junctions [AJ]) and desmosomes (Farquhar and Palade Citation1963). Since most published work identifies TJ and AJ as targets and regulators of Rho family GTPases, we will focus here on TJ and AJ, and refer the reader to recent reviews about desmosomes (Garrod and Chidgey Citation2008, Green et al. Citation2010) for additional information in this area.
The functions of TJ and AJ
TJ and AJ are of fundamental importance in the development and physiology of epithelial cells and tissues, since they allow cells to adhere to each other, to form barriers between body compartments, and to receive and transmit extracellular and intracellular signals. TJ form a selective paracellular permeability barrier to the movement of water, ions, and other solutes (Van Itallie and Anderson Citation2006), and act as anatomical fences separating the apical from the basolateral domains of the plasma membrane (Diamond Citation1977). AJ are responsible for cell-cell adhesion, and play a key role in tissue sorting during development, through the expression of specific cadherin isoforms (Wheelock and Johnson Citation2003, Gumbiner Citation2005, Stepniak et al. Citation2009). Moreover, TJ and AJ are focal sites of anchoring and organization of the cytoskeleton (Mege et al. Citation2006, Meng and Takeichi Citation2009), and function as signaling hubs in the control of gene expression and cell proliferation, through the interaction of several of their components with transcription factors and other signaling molecules (Heuberger and Birchmeier Citation2010, McCrea et al. Citation2009).
The molecular structure of TJ and AJ
The architectural organization of TJ and AJ comprises transmembrane proteins that function either as adhesion receptors or as paracellular channels, and cytoplasmic proteins, that associate with the intracellular domains of transmembrane proteins, and link them to the cytoskeleton and to adaptor and signaling proteins. The molecular constituents of the membrane domain of TJ comprise four-pass membrane proteins and Ig-like adhesion molecules. The four-pass membrane proteins of TJ include occludin, tricellulin and claudins, the latter playing a pivotal role in defining the ionic paracellular permeability properties of TJ (Tsukita and Furuse Citation2002, Van Itallie and Anderson Citation2006, Furuse Citation2009). The Ig-like adhesion molecules of TJ include JAM-1, the cocksackie adenovirus receptor (CAR) (Hirabayashi and Hata Citation2006) and LSR (Masuda et al. Citation2011). The cytoplasmic domain of TJ contains several proteins with PDZ domains, such as ZO-1, ZO-2, ZO-3, MUPP1, and MAGI1 (Gonzalez-Mariscal et al. Citation2003, Guillemot et al. Citation2008b, Fanning and Anderson Citation2009). These proteins form complexes that act as docking platforms for membrane proteins, and recruit signaling and adaptor proteins, such as cingulin, paracingulin, ZONAB and symplekin (Gonzalez-Mariscal et al. Citation2003, Schneeberger and Lynch Citation2004, Guillemot et al. Citation2008b). Two major polarity complexes involved in the establishment and maintenance of apico-basal polarity are also associated with TJ. The aPKC-Par3-Par6 complex is linked to TJ through interactions of Par3 with JAM-A, whereas the Crumbs-PALS1-PATJ polarity complex is linked to TJ through interactions of PATJ with ZO-3 and claudins (Shin et al. Citation2006). At AJ, the Ig-like adhesion molecule nectin is associated with the cytoplasmic proteins afadin and ponsin (Takai and Nakanishi Citation2003), whereas the calcium-dependent adhesion receptor E-cadherin interacts with several intracellular proteins, including p120-catenin (p120ctn), β-catenin and α-catenin (Meng and Takeichi Citation2009). These cytoplasmic complexes organize the anchoring of AJ to the actin and microtubule cytoskeletons. Indeed, α-catenin, together with α-actinin, vinculin and eplin, participates in linking E-cadherin to actin filaments (Drees et al. Citation2005, Meng and Takeichi Citation2009), whereas the afadin-ponsin complex links nectin to actin filaments (Takai and Nakanishi Citation2003). p120ctn not only regulates E-cadherin clustering and stability (Reynolds Citation2007), but also connects E-cadherin to microtubules, through the PLEKHA7-Nezha complex (Meng and Takeichi Citation2009). At TJ, the link to the actin cytoskeleton is provided primarily through the ZO proteins ZO-1, ZO-2 and ZO-3 (Fanning et al. Citation1998, Wittchen et al. Citation1999). Importantly, the cytoskeletal networks associated with TJ and AJ undergo a profound reorganization and reorientation upon junction assembly, disassembly and maturation (Mege et al. Citation2006), and small GTPases play a key role in such cytoskeletal remodeling.
Rho family GTPase: Targets and effectors
In mammalian organisms, the Rho family of GTPases can be divided into six major groups: the Rho proteins (RhoA, RhoB and RhoC), the Rac proteins (Rac 1, 2, 3, and RhoG), the Cdc42-like proteins (Cdc42, TC10, TC10, TCL, Chp/Wrch2 and Wrch1), the Rnd proteins (Rnd1, Rnd2, Rnd3/RhoE), the RhoBTB proteins (RhoBTB1, RhoBTB2, RhoBTB3) and the Miro (mitochondrial Rho) proteins (Miro1, Miro2) (Wennerberg and Der, Citation2004). RhoA, Rac1 and Cdc42 are the best studied among the Rho family GTPases, and will be the focus of this review. When activated by binding to GTP, Rho family GTPases activate effectors, including protein and lipid kinases, which affect multiple cellular processes: cytoskeletal organization, membrane trafficking, cell survival, apoptosis, and gene expression (Fukata and Kaibuchi Citation2001, Ridley Citation2001, Benitah et al. Citation2004, Jaffe and Hall Citation2005). Through these targets, Rho family GTPases ultimately control cell-cell adhesion, cell motility, cell proliferation and morphogenesis.
Rho family GTPases have a major impact on the organization and contractility of the cytoskeleton (). Rho promotes the formation of actin stress fibers, and enhances the contractility of actomyosin bundles. This is achieved through several mechanisms, including ROCK kinase-mediated phosphorylation and inhibition of the myosin light chain phosphatase (MLCP), and phosphorylation and activation of the myosin light chain kinase (MLCK), both of which increase myosin light chain phosphorylation, and hence actomyosin contractility (Amano et al. Citation2010). Moreover, actin filaments are stabilized by inhibition of the actin-severing protein cofilin, through ROCK kinase-mediated phosphorylation and activation of LIM kinase () (Maekawa et al. Citation1999). Furthermore, RhoA, Rac1 and Cdc42 regulate actin polymerization, albeit through different mechanisms. While RhoA activation stimulates unbranched actin polymerization through Diaphanous (Dia) and formins, Rac1 and Cdc42 promote polymerization and branching of actin filaments through the effectors WAVE and WASP, both of which activate the Arp2/3 complex to nucleate and polymerize actin filaments (). Rac1 and Cdc42 also stimulate, through PAK kinases, the activity of LIM kinase (Edwards et al. Citation1999, Jaffe and Hall Citation2005). Moreover, activation of Rac1 and Cdc42 inhibits the association of IQGAP with β-catenin, thereby promoting cadherin-mediated cell-cell adhesion (Kuroda et al. Citation1998) ().
Figure 1. Cytoskeletal effectors of Rho family GTPases. Schematic diagram representing the Rho family GTPases RhoA, Rac1 and Cdc42, their activities on major downstream effectors and targets, and their effects on cytoskeletal and junction organization. Green lines with arrows indicate activation, red lines with bar indicate inhibition, black lines with arrows indicate functional target/effect. Dia, diaphanous; ROCK, Rho kinase; PAK, p21-activated kinase; IQGAP, IQ motifs and GAP-related-domain containing protein; WAVE, WASP family Verprolin homologous protein; WASP, Wiskott-Aldrich syndrome protein; MLCK, myosin light chain kinase; MLCP, myosin light chain phosphatase; CRMP-2, Collapsin response mediator protein-2; LIMK, LIM domain kinase; CLIP-170, CAP-GLY domain containing linker protein; Arp, actin-related protein. See text for additional details. This Figure is reproduced in colour in Molecular Membrane Biology online.
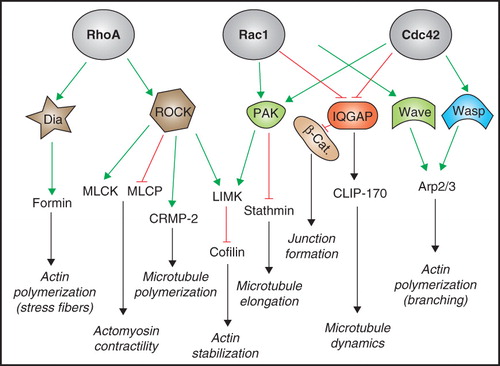
Microtubule dynamics is also regulated by Rho family GTPases (Fukata et al. Citation2002) (). In cancer cells, Cdc42- and Rac1-dependent activation of PAK results in phosphorylation of stathmin, resulting in microtubule stabilization (Daub et al. Citation2001, Wittmann et al. Citation2004). In neurons, Rho-dependent activation of ROCK inactivates CRMP-2, leading to microtubule disassembly and growth cone collapse (Arimura et al. Citation2005), and in Hela cells the Rho effector mDia coordinates the alignment of actin and microtubules along the long axis of the cell (Ishizaki et al. Citation2001). Moreover, Rac1 and Cdc42 promote the capture of microtubule plus-end at the leading edge of migrating cells, by promoting the interaction of CLIP-170 with the Rac effector IQGAP (Kuroda et al. Citation1998, Fukata et al. Citation1999). Finally, Rho family GTPases also regulate the interaction of the plus-end binding protein EB1 with the adenomatous polyposis coli (APC) tumor suppressor protein (Jaffe and Hall Citation2005).
Besides being master regulators of cytoskeletal organization, Rho family GTPases influence signaling pathways that regulate gene expression, for example through the serum-response factor (SRF), a coactivator of promoters containing the serum-response element (SRE), and through YAP/TAZ (Dupont et al. Citation2011). SRF translocation to the nuleus, together with the coactivator MAL, is promoted by Rho family GTPase-dependent actin remodeling, and depends on E-cadherin (Miralles et al. Citation2003, Busche et al. Citation2010). Recently it was shown that RhoA activity is required to allow the transcription factors YAP and TAZ to act as nuclear relays of mechanical signals exerted by extracellular matrix rigidity and cell shape, to stimulate cell proliferation and growth (Dupont et al. Citation2011). Additional mechanisms of regulation of gene expression by Rho family GTPases include modulation of kinases and scaffold proteins of the JNK and p38 MAP kinase pathways (Benitah et al. Citation2004).
Finally, the activities of Rho family GTPases influence many other cellular processes, such as the cell cycle, membrane trafficking, and apoptosis (Ridley Citation2001, Coleman et al. Citation2004, Jaffe and Hall Citation2005). In view of their multiple roles in regulating such fundamental cellular processes, it is not surprising that deregulation of Rho family GTPases has been implicated in cancer development and metastasis (Sahai and Marshall Citation2002a). This appears to depend primarily on deregulated expression and mutation of GEFs and GAPs and deregulated GTPase expression, but not on GTPase mutations (Vigil et al. Citation2010).
Regulation of Rho family GTPases
Small GTPases are active when bound to GTP, and inactive when bound to GDP. The hydrolysis of GTP, and thus the inactivation of GTPases, is promoted by GTPase activating proteins (GAPs). The release of GDP, and thus activation of the GTPase by binding to GTP, is stimulated by guanine exchange factors (GEFs). A third class of Rho GTPase regulatory proteins consists of guanine nucleotide dissociation inhibitors (GDIs), which bind to and stabilize the GDP-bound, inactive forms of GTPases. Regulation can also occur through pathways that regulate the stability and the localization of small GTPases. For example, the E3 ubiquitin ligase Smurf regulates RhoA degradation downstream of the Par-6/Par-3/aPKC polarity complex (Wang et al. Citation2003), and plakophilin targets RhoA to epithelial junctions (Godsel et al. Citation2010).
Guanine nucleotide exhange factors (GEFs)
The human genome contains over 70 genes coding for GEFs, many of which have not been characterized in detail (Schmidt and Hall Citation2002, Rossman et al. Citation2005). The same GTPase can be activated by different GEFs, and many GEFs activate more than one GTPase. The common structural feature of GEFs is the presence of two Dbl-homology (DH) domains that are crucial for their catalytic activity and one Pleckstrin Homology (PH) domain, responsible for binding phospholipids, and thus potentially important for plasma membrane targeting. A small number of GEFs, related to Dock180, lack the DH domain, and contain instead Dock Homology Regions (DHR), which promote guanine nucleotide exchange (Meller et al. Citation2005). A tight regulation of GEFs activity is crucial, and is carried out by different mechanisms, including protein-protein interactions (Schmidt and Hall Citation2002, Rossman et al. Citation2005), targeting to defined intracellular compartments by interaction with GTPases and adaptor proteins (Vaughan et al. Citation2011), relief of intramolecular inhibitory sequences (Katzav et al. Citation1991), micro-RNA dependent modulation of their levels of expression (Cottonham et al. Citation2010), and signal-mediated phosphorylation and degradation (Woodcock et al. Citation2009).
GTPase Activating Proteins (GAPs)
The human genome contains about 80 genes coding for GAPs, which act as inhibitors of small GTPases, by stimulating the low hydrolytic activity of RhoGTPases (Moon and Zheng Citation2003). Members of this family are multi-domains proteins characterized by the presence of a conserved RhoGAP domain. Two GAP proteins, Abr and Bcr, also have GEF activity, and the characteristic DH domain of GEFs (Tan et al. Citation1993, Cho et al. Citation2007). Many other GAPs have additional domains involved in protein-protein and protein-lipid interactions, such as PH and SH3 domains (Moon and Zheng Citation2003). A large numbers of GAPs show a weak selectivity towards RhoGTPases, while others are specific to a defined partner (Ridley et al. Citation1993, Moon and Zheng Citation2003, Tcherkezian and Lamarche-Vane Citation2007). GAPs are regulated by several mechanisms, including protein-protein interactions, post-translational modifications, and binding of second messenger molecules (Moon and Zheng Citation2003, Bernards and Settleman Citation2005).
Guanine nucleotide dissociation inhibitors (GDIs)
There are only three RhoGDIs so far described in the human genome (Olofsson Citation1999). RhoGDI1 is the most abundantly represented, and interacts with a large number of Rho family members (Fukumoto et al. Citation1990), whereas RhoGDI2 is specific of hematopoietic cells, and show a higher specificity for the Rac1 family (Scherle et al. Citation1993). RhoGDI3 is found in low abundance and is specifically expressed in lung, brain and testis (Zalcman et al. Citation1996). GDIs interfere with Rho GTPase signaling pathway by various mechanisms, including inhibition of GDP dissociation, inhibition of GAP-mediated GTP hydrolysis (Van Aelst and D'Souza-Schorey Citation1997), and cytoplasmic sequestration of RhoGTPases (DerMardirossian and Bokoch Citation2005). Interestingly, several RhoGTPases do not effectively interact with GDIs, suggesting that this inhibitory mechanism is restricted to a subset of GTPases (Olofsson Citation1999, DerMardirossian and Bokoch Citation2005).
The role of Rho family GTPases and Rap1 in the formation and maintenance of epithelial junctions
The formation, maintenance and remodeling of junctions, and hence epithelial morphogenesis and differentiation, require a precisely orchestrated mechanism of activation and inactivation of Rho family GTPases in space and time (Braga and Yap Citation2005, Samarin and Nusrat Citation2009, Watanabe et al. Citation2009). In addition to Rho family GTPases, the Ras-like protein Rap1 also regulates cadherin-based adhesion, JAM-A, and TJ-mediated barrier regulation (Kooistra et al. Citation2007, Boettner and Van Aelst Citation2009, Severson et al. Citation2009).
RhoA
The first evidence for the role of RhoA in the control of epithelial junctions came from the observation that inhibition of RhoA activity, either by expression of the Rho-inactivating enzyme C3 transferase, or by expression of dominant-negative mutants of RhoA, resulted in the thinning of the junction-associated actin filament belt, and the disassembly of both TJ and AJ (Nusrat et al. Citation1995, Braga et al. Citation1997, Takaishi et al. Citation1997, Gopalakrishnan et al. Citation1998, Jou et al. Citation1998, Bruewer et al. Citation2004). Conversely, the expression of constitutively active mutants of RhoA induced a thickening of actin filaments, which stabilized the AJ (Nusrat et al. Citation1995, Bruewer et al. Citation2004). Both ROCK and Diaphanous (Dia) have been identified as downstream RhoA effectors involved in the maintenance of the structure and function of TJ and AJ (Hirase et al. Citation2001, Walsh et al. Citation2001, Sahai and Marshall Citation2002b, Shewan et al. Citation2005) (). In addition, the PRK2 effector is also involved in the Rho-dependent assembly of keratinocyte junctions, through the Fyn tyrosine kinase (Calautti et al. Citation2002). RhoA is also implicated in the modulation and disassembly of AJ in response to pathological or experimental stimuli, such as inflammatory cytokines or calcium depletion. The mechanisms include translocation of the guanine-exchange factor GEF-H1 to the AJ actomyosin contractile ring, and activation of ROCK kinase (Utech et al. Citation2005, Samarin et al. Citation2007).
Rac1
Rac1 plays a key role in the formation and the remodeling of AJ, but does not appear to act directly on TJ (Nakagawa et al. Citation2001). By promoting the formation of lamellipodia, Rac1 initiates the assembly of the primordial epithelial cell-cell contacts and allows the subsequent accumulation of E-cadherin at these sites. This leads to a positive feedback loop of spreading cell-cell adhesion, through the E-cadherin-dependent activation of phosphatidylinosotol-3-kinase (PI3-K) (Pece et al. Citation1999), the junctional recruitment of the Rac1 activator Tiam1, and the further activation of Rac1 (Nakagawa et al. Citation2001, Kovacs et al. Citation2002). Indeed, Tiam-1-mediated Rac1 activation promotes E-cadherin adhesion, whereas Tiam1 depletion inhibits the formation of AJ (Hordijk et al. Citation1997, Malliri et al. Citation2004, Mertens et al. Citation2005). Although it has been proposed that Tiam1 is recruited to membranes through its interaction with phospholipids generated by PI3-K activation (Michiels et al. Citation1997, Sander et al. Citation1998), some studies have questioned the role of PI3-K in the initial phases of E-cadherin-induced Rac1 activation (Nakagawa et al. Citation2001, Ehrlich et al. Citation2002, Lambert et al. Citation2002, Goodwin et al. Citation2003), and additional mechanisms that contribute to Tiam1 recruitment to membranes have been characterized (Bourguignon et al. Citation2000, Schmidt and Hall Citation2002, Guillemot et al. Citation2008a). Importantly, a basal Rac1 activity is necessary for the maintenance of AJ, since expression of both constitutively active and dominant negative isoforms of Rac1 leads to disruption of AJ (Braga et al. Citation1997, Takaishi et al. Citation1997, Jou and Nelson Citation1998, Bruewer et al. Citation2004). Rac1 stabilizes AJ by promoting actin polymerization through WAVE and Arp2/3, and by negatively regulating the interaction of IQGAP with β-catenin, which destabilizes the E-cadherin-based cell-cell contacts (Kuroda et al. Citation1998, Fukata et al. Citation1999) (). Interestingly, activation of Rac1 can have different consequences on cell-cell adhesion and cell migration, depending on experimental context and environmental signals (Sander et al. Citation1998). For example, growth-factor-induced activation of Rac1 results in disruption of AJ, lamellipodia formation and scattering of epithelial cells (Ridley et al. Citation1995, Potempa and Ridley Citation1998). In contrast, in other model systems Rac1 protects AJ from disassembly, prevents cell scattering, and promotes the epithelial phenotype (Hordijk et al. Citation1997, Gopalakrishnan et al. Citation2002).
Cdc42
The role of Cdc42 in the assembly and maintenance of epithelial junctions is controversial. Nectin- and E-cadherin-mediated adhesion promote the activation of Cdc42 (Kim et al. Citation2000, Kawakatsu et al. Citation2002, Fukuhara et al. Citation2003, Citation2004), and depletion of the Cdc42 GEF Tuba results in thinning of lateral actin filaments, and changes in the junctional architecture in epithelial cells (Otani et al. Citation2006), suggesting that Cdc42 controls actin dynamics at junctions. However, expression of dominant negative Cdc42 does not induce significant changes in the perijunctional actin cytoskeleton (Kodama et al. Citation1999, Rojas et al. Citation2001). The stimulation of filopodia formation could be a mechanism through which Cdc42 activation promotes the formation of cadherin-based contacts (Vasioukhin et al. Citation2000, Otani et al. Citation2006). However, in keratinocytes Cdc42 activation and filopodia formation do not occur upon new junction formation (Erasmus et al. Citation2010). Overexpression of constitutively active Cdc42 disrupts both TJ and AJ, and Cdc42 activation is implicated in epithelial-mesenchymal transition, and in the Src-mediated phosphorylation and subsequent ubiquitination and degradation of E-cadherin (Kroschewski et al. Citation1999, Patel et al. Citation2005: Chen et al. Citation2006. Shen et al. Citation2008b). The role of Cdc42 in regulating the establishment of apico-basal epithelial polarity is unique, and distinguishes Cdc42 from Rac1. Cdc42 activation is essential in the formation of the Par-3/Par-6/aPKC polarity complex and in the establishment of epithelial apico-basal polarity (Yamanaka et al. Citation2001). Furthermore, Cdc42 and its GEF Tuba control lumen formation in epithelial cells in 3-D culture, by regulating mitotic spindle orientation and apical membrane exocytosis (Bryant et al. Citation2010, Qin et al. Citation2010).
Rap1
The role of Rap1 in cell-cell junction formation was first demonstrated in D. melanogaster, where Rap1 maintains the circumferential distribution of AJ (Asha et al. Citation1999, Knox and Brown Citation2002). In mammalian cells, Rap1 is required for the homotypic interaction of E-cadherin, rescues the junction disruption phenotype of cells expressing oncogenic Ras, and promotes AJ maturation (Hogan et al. Citation2004, Price et al. Citation2004). Rap1 is activated upon cadherin- and nectin-mediated cell-cell contact, through different GEFs, including Epac in endothelial cells, and C3G, PDZ-GEF1 and PDZ-GEF2 in epithelial cells (Kooistra et al. Citation2007, Boettner and Van Aelst Citation2009, Severson et al. Citation2009). The downstream effectors of Rap1 in epithelial cells include afadin/AF-6 (Boettner et al. Citation2000), and Rac1/Cdc42, both of which are activated following Rap1 activation (Sato et al. Citation2006). The activation of these latter GTPases has in turn been linked to the junctional recruitment and/or activation of Vav2, Tiam1 and FRG, following cadherin-dependent adhesion (Fukuyama et al. Citation2006). Thus, Rap1 is part of a positive feedback loop that involves Rho family GTPases, to promote the maturation of nectin- and cadherin-based cell-cell adhesion. However, Rap1 does not seem to be important for maintenance of AJ, once they have been assembled (Hogan et al. Citation2004, Fukuyama et al. Citation2005, Dube et al. Citation2008).
The role of junctional molecules in the regulation of Rho family GTPases
p120catenin
p120ctn is a Src kinase substrate and an armadillo domain family protein, which associates with the juxtamembrane domain of E-cadherin (Reynolds et al. Citation1994). In mice, p120ctn knockout leads to embryonic death, and its conditional inactivation leads to abnormal signaling, proliferation and growth (Davis and Reynolds Citation2006, Perez-Moreno et al. Citation2006, Schlegelmilch et al. Citation2011, Silvis et al. Citation2011). In fibroblasts, p120ctn overexpression inhibits RhoA activity and stimulates Rac1 and Cdc42 activities, resulting in a migratory phenotype, loss of stress fibers, and increased lamellipodia and filopodia formation (Anastasiadis et al. Citation2000, Noren et al. Citation2000, Grosheva et al. Citation2001). It has been proposed that cytoplasmic p120ctn binds to and inhibits RhoA, acting as a GDP dissociation inhibitor, whereas cadherin clustering promotes RhoA activation, by recruiting cytoplasmic p120ctn to junctions (Anastasiadis et al. Citation2000, Noren et al. Citation2000, Grosheva et al. Citation2001) (). In fibroblasts, p120ctn is present in a complex with the Rac1 GEF Vav2 (Noren et al. Citation2000), suggesting that p120ctn may stimulate Rac1 activity through Vav2, and thus indirectly inhibit RhoA through activation of the antagonistic Rac1 pathway (Nimnual et al. Citation2003) (). Another possible mechanism is indicated by the observation that Rac1 activation through receptor-mediated signaling promotes the association of the Rho GAP p190 RhoGAP with cadherin-bound p120ctn, resulting in localized inhibition of RhoA (Wildenberg et al. Citation2006) (). Since these regulatory interactions have been characterized in cultured fibroblasts, it is not clear to what extent they are applicable to epithelial cells (Anastasiadis and Reynolds Citation2001). In fact, studies on epithelial model systems have not provided a definitive answer about the role of p120ctn in RhoA regulation in normal polarized columnar epithelial cells. RhoA activity was reported to be increased upon depletion of p120ctn in serum-starved MDCK monolayers (Dohn et al. Citation2009) and breast cancer cells (Yanagisawa and Anastasiadis Citation2006, Yanagisawa et al. Citation2008). Moreover, the epidermis and primary keratinocytes isolated from p120ctn knockout mice show increased RhoA activity, increased stress fibers and reduced lamellipodia (Perez-Moreno et al. Citation2006). However, in another study, adenovirus-mediated overexpression of p120ctn resulted increased RhoA activity in primary keratinocytes, and decreased RhoA activity in fibroblasts, suggesting a cell-context-dependent function of p120ctn (Cozzolino et al. Citation2003). Furthermore, RhoA activity was significantly decreased upon p120ctn depletion in MDCK cells gown as cysts in 3-D culture (Marciano et al. Citation2011), supporting the notion that in epithelial cells junctional p120ctn stimulates, rather than inhibits, RhoA activity (). It should also be noted that the p120ctn isoform 1A, which associates with p190RhoGAP, is expressed in significant amounts in mesenchymal cells, but in very low amounts in epithelial cells (Marciano et al. Citation2011). Therefore the possible involvement of p190RhoGAP in down-regulating RhoA activity at epithelial junctions remains to be clarified ().
Figure 2. Regulation of small GTPases by junctional proteins. Schematic diagrams illustrating the major proteins and pathways involved in the regulation of RhoA (A), Rac1 (B), Rap1 (C), and Cdc42 (D) at junctions of mammalian epithelial cells. (E) is a graphic legend for panels A, B, C, D. Arrows indicate either physical/functional interaction, activation, or promotion of junctional recruitment. Lines with bar indicate inhibition. Dotted lines indicate indirect/unknown interaction. α = α-catenin; PL7 = PLEKHA7; p114RG = p114 RhoGEF; β-cat. = β-catenin. See text for additional details. This Figure is reproduced in colour in Molecular Membrane Biology online.
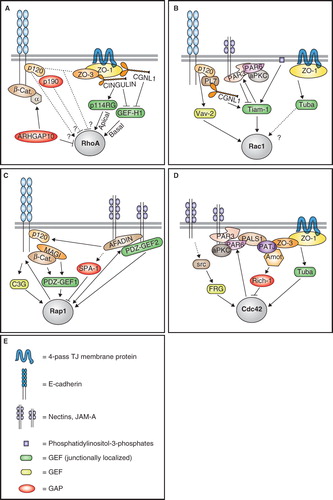
MAGI1
MAGI1 is a member of the membrane-associated-guanylate kinase (MAGUK) family of proteins, which are characterized by the presence of PDZ, SH3 and GUK domains. MAGI1 is localized in the cytoplasmic region of TJ and AJ, where it has been reported to associate with cadherin and nectin/JAM-A-based junctional protein complexes, and β-catenin (Ide et al. Citation1999, Dobrosotskaya and James Citation2000). In endothelial cells, MAGI1 is required for the activation of the small GTPase Rap1, by acting as a scaffolding protein for the Rap1 activator PDZ-GEF1 (Sakurai et al. Citation2006) ().
JAM-A, nectins and afadin
Both JAMs (Junctional Adhesion Molecules) and nectins are members of the family of immunoglobulin-like adhesion molecules, and interact with the cytoplasmic scaffold proteins afadin and ZO-1 at epithelial junctions (Mandai et al. Citation1997, Martin-Padura et al. Citation1998, Ebnet et al. Citation2000, Takai et al. Citation2008). In addition, JAM-A interacts with the polarity complex protein Par-3 (Martin-Padura et al. Citation1998, Ebnet et al. Citation2001, Itoh et al. Citation2001) (). Afadin is a multidomain protein containing PDZ, Ras- and actin-binding domains (Kuriyama et al. Citation1996, Mandai et al. Citation1997). JAM-A knockout mice show altered intestinal function, with increased permeability, proliferation and inflammation (Laukoetter et al. Citation2007), indicating multiple roles of JAM-A in TJ barrier regulation and signaling, in agreement with studies on cultured cells (Liu et al. Citation2000, Mandell et al. Citation2005). Conversely, knockout of afadin leads to early embryonic death, with loss of epithelial polarity (Ikeda et al. Citation1999, Zhadanov et al. Citation1999), indicating an essential role in morphogenesis. Interestingly, conditional knockout of afadin in intestinal epithelial cells after birth phenocopies the effect of JAM-A knockout in the intestine (Tanaka-Okamoto et al. Citation2011). JAM-A and nectins stimulate the activity of Rap1, and thus regulate junction integrity and cell migration, through afadin, which binds to Rap1 and functions as a Rap1 effector (Boettner et al. Citation2000, Mandell et al. Citation2005, Severson et al. Citation2009) (). One characterized mechanism is through the formation of a complex between JAM-A, afadin and the Rap1 GEF PDZ-GEF2 (Dube et al. Citation2008) at junctions (Severson et al. Citation2009). Afadin also promotes E-cadherin stabilization through its interaction with p120ctn, and has also been reported to bind to the Rap1 GAP SPA-1, and thus influence integrin-mediated adhesion (Su et al. Citation2003) (). Another putative Rap1 GAP, SPAL-3, has been localized at junctions (Matsuda et al. Citation2008), but its interaction with junctional proteins is not known. Finally, nectins are involved in Rac1 and Cdc42 activation upon the formation of the primordial cell-cell contacts, through mechanisms involving Rap1 (Fukuyama et al. Citation2005), c-Src, the Cdc42 GEF FRG, and the Rac1 GEF Vav2 (Kim et al. Citation2000, Kawakatsu et al. Citation2002, Fukuhara et al. Citation2003, Citation2004) ().
Par-6 and Par-3
Par-3 and Par-6 are PDZ-domain-containing proteins, which form a complex with atypical protein kinase C (aPKC) and participate in the biogenesis of epithelial TJ (Izumi et al. Citation1998, Joberty et al. Citation2000, Johansson et al. Citation2000, Lin et al. Citation2000). The Par-3/Par-6/aPKC complex is involved in the establishment of apico-basal and antero-posterior polarity in a variety of invertebrate and vertebrate cell types (Kemphues et al. Citation1988, Ohno Citation2001), and is a signaling module that acts both as an upstream regulator and a downstream target of the small GTPases (). Binding of activated Cdc42 to Par-6 is required for the association of the Par-6/aPKC complex with Par-3, leading to TJ formation and establishment of apico-basal polarity (Joberty et al. Citation2000, Johansson et al. Citation2000, Lin et al. Citation2000) (). Par-6 also interacts with the transforming Cdc42-like GTPase Wrch-1, but activation of Wrch-1 results in the disruption of TJ (Brady et al. Citation2009). Par-3 is both a target and a regulator of Rac1 activity. Rac1 activation is required to recruit Par-3 to junctions, since in keratinocytes lacking Tiam1 junction assembly and localization of Par-3 to TJ are delayed (Mertens et al. Citation2005) (). However, in MDCK cells depletion of Par-3 results in a Tiam-1-dependent increase in Rac1 activity, suggesting that Par-3 recruits and negatively regulates Tiam-1 function at junctions (Chen and Macara Citation2005) (). In neuronal cells and fibroblasts, the Par-3/Par-6 complex promotes the recruitment of Tiam-1 to the growing cone and lamellipodia, respectively, but in this case Rac1 activity is stimulated, and not inhibited (Nishimura et al. Citation2005). In this context, RhoA-ROCK-kinase-mediated phosphorylation of Par-3 acts as an inhibitory switch, resulting in Rac1 inactivation, and disruption of the Par complex (Nakayama et al. Citation2008). In neuronal cells, Par-6 also controls RhoA activity through p190RhoGAP (Zhang and Macara Citation2008). However, such regulation has not been demonstrated in epithelial cells.
ZO proteins
ZO proteins (ZO-1, ZO-2 and ZO-3) are MAGUK family proteins localized in the cytoplasmic domain of TJ (Mitic and Anderson Citation1998), where they play redundant and non-redundant roles as scaffolds of TJ membrane proteins and anchors for the actin cytoskeleton (Umeda et al. Citation2006, Van Itallie et al. Citation2009, Xu et al. Citation2009). Mice knockout for either ZO-1 or ZO-2 have an embryonic lethal phenotype, whereas mice lacking ZO-3 have an apparently normal phenotype (Katsuno et al. Citation2008, Xu et al. Citation2008).
Several lines of evidence indicate that ZO proteins are involved in the regulation of RhoA, Cdc42 and Rac1. MDCK cells overexpressing the N-terminal part of ZO-3 (NZO-3) show reduced RhoA activity, increased migratory behaviour, and decreased stress fibers (Wittchen et al. Citation2003). Since the C-terminal region of ZO-3 binds both to NZO-3 and p120ctn, it was proposed that NZO-3 competes with p120ctn for binding to the C-terminus of endogenous ZO-3, and thus renders p120ctn available to interact with other proteins, such as Vav-2 or Rho, resulting in the inhibition of RhoA activity (Wittchen et al. Citation2003) (). However, a direct role of ZO-3 in regulating RhoA activity in epithelial cells has not been demonstrated. The Cdc42 GEF Tuba forms a complex with ZO-1, and requires ZO-1 in order to be recruited to junctions of colonic epithelial cells (Otani et al. Citation2006) (). MDCK cells depleted of Tuba show delayed AJ formation and reduced activation of Cdc42 during the calcium switch assay (Otani et al. Citation2006). Similarly, MDCK cells lacking ZO-1 and depleted of ZO-2 show delayed AJ assembly, and fail to activate Rac1 during the calcium switch (Ikenouchi et al. Citation2007). Taken together, these data suggest that the ZO protein complex is required to achieve efficient activation of Rac1 and Cdc42 during junction assembly, possibly through the direct or indirect recruitment of Tuba to junctions ().
E-cadherin, β-catenin and α-catenin
E-cadherin is the major calcium-dependent adhesion molecule of epithelial AJ, and its cytoplasmic domain interacts with the armadillo family proteins p120ctn and β-catenin. In turn, β-catenin binds α-catenin, which plays a key role in anchoring AJ to the actin cytoskeleton (Meng and Takeichi Citation2009). The importance of E-cadherin and associated proteins for cell-cell adhesion and morphogenesis is highlighted by the observation that the targeted disruption of these proteins gives an embryonic lethal phenotype in mice (Larue et al. Citation1994, Haegel et al. Citation1995, Torres et al. Citation1997).
E-cadherin is involved in the activation of both Rac1/Cdc42 and Rap1. Phosphatidylinositol-triphosphates (PIP3) generated at the membrane upon cadherin-mediated activation of PI-3-kinase (PI3-K) have been implicated in the binding to the PH domain of Rac1/Cdc42 GEFs, such as Tiam1 and Asef, leading to their recruitment to junctions (Sander et al. Citation1998, Pece et al. Citation1999, Kovacs et al. Citation2002, Malliri et al. Citation2004, Muroya et al. Citation2007) (see also Rac1 section). Furthermore, E-cadherin stimulates the activation of Rap1 during the early phases of junction assembly, through the transient interaction of its cytoplasmic domain with the Rap1 GEF C3G (Hogan et al. Citation2004) (). The ability of E-cadherin to signal to small GTPases may depend on its phosphorylation, as suggested by the observation that depletion of the cadherin-associated protein tyrosine phosphatase PTP-PEST affects RhoA and Rac1 activities, and enhances migratory activity of colon carcinoma cells (Espejo et al. Citation2010).
β-catenin contributes to the local activation of Rap1, by interacting with the Rap1 GEF, PDZ-GEF1, directly, and through its interacting partners MAGI-1 and MAGI-2 (Mino et al. Citation2000, Sakurai et al. Citation2006) ().
With regards to α-catenin, its possible involvement as a target of RhoA is indicated by its interaction with the RhoA GAP ARHGAP10 (Sousa et al. Citation2005). The activity of ARHGAP10 favors the association of α-catenin with cell-cell contacts, as well as enabling invasion by the bacterial pathogen Listeria (Sousa et al. Citation2005) (). Another Rho GAP, ARHGAP12, has been localized at epithelial junctions, but its interaction with junctional proteins has not been characterized (Matsuda et al. Citation2008).
Angiomotin
Angiomotin (Amot) belongs to a family of proteins characterized by a glutamine-rich domain, a coiled-coil domain, and a C-terminal PDZ-binding domain (Bratt et al. Citation2002, Sugihara-Mizuno et al. Citation2007). Amot interacts with the polarity complex proteins PALS1, PATJ and Par-3, and recruits the Cdc42 GTPase activating protein Rich1 to TJ in MDCK cells (Wells et al. Citation2006) (). The activity of Rich1 does not affect AJ, but is required to maintain the organization and barrier function of TJ, probably by allowing the cycling of Cdc42 between the GDP- and GTP-bound states, which is necessary to maintain the stability of the TJ polarity complexes (Wells et al. Citation2006). Amot has also been implicated in promoting cell migration and the breakdown of junctions in endothelial cells (Troyanovsky et al. Citation2001, Bratt et al. Citation2005). In fact, Amot interacts with the RhoGEF Syx through a ternary complex with Pals1 and PatJ, thereby controlling the spatially restricted activation of RhoA at lamellipodia of migrating endothelial cells (Ernkvist et al. Citation2009). In summary, Amot is a dual regulator of small GTPase activity: during endothelial migration it promotes local RhoA activation through Syx, whereas in epithelial cells it contributes to the maintenance of TJ by negatively regulating Cdc42 activity through Rich1. Studies on knockout mice show that Amot has a critical role during vascular patterning and endothelial polarization (Aase et al. Citation2007).
Cingulin and paracingulin
Cingulin and paracingulin are structurally similar proteins, comprising globular N-terminal domains, a central coiled-coil domain and small globular tails (Citi et al. Citation1988, Ohnishi et al. Citation2004, Guillemot and Citi Citation2006b). Both proteins are localized at TJ, where they are recruited by interaction with ZO-1 and other proteins (Citi et al. Citation1989, Cordenonsi et al. Citation1999, Ohnishi et al. Citation2004, Pulimeno et al. Citation2011). However, paracingulin is also localized at AJ (Ohnishi et al. Citation2004), where it forms a complex with E-cadherin, p120ctn and PLEKHA7 (Paschoud et al. Citation2010, Pulimeno et al. Citation2011). Both cingulin and paracingulin form a complex with and interact directly with the Rho GEF Lfc/GEF-H1 (Ren et al. Citation1998, Krendel et al. Citation2002, Benais-Pont et al. Citation2003), providing a mechanism to down-regulate Rho signaling and G1/S phase transition, as cell density increases (Aijaz et al. Citation2005, Guillemot et al. Citation2008a, Citi et al. Citation2009) (). Interestingly in fibroblasts, which lack TJ, GEF-H1 is sequestered by microtubules, and microtubule depolymerization leads to RhoA activation (Liu et al. Citation1998, Ren et al. Citation1998, Glaven et al. Citation1999, Krendel et al. Citation2002). Cingulin is also implicated in the recruitment to TJ of the Rho GEF p114RhoGEF, which has been proposed to promote spatially restricted, apical RhoA activation during junction assembly of corneal epithelial cells, while GEF-H1 would act on basally localized RhoA (Terry et al. Citation2011) (). However, cingulin depletion from columnar epithelial cells (Guillemot and Citi Citation2006a), and cingulin knockout in epithelial endodermal cells of embryoid bodies (Guillemot et al. Citation2004) do not have detectable inhibitory effects on TJ assembly, suggesting that the p114RhoGEF-mediated regulation of TJ assembly by cingulin may be restricted to specific cell types. The spatial regulation of RhoA activity at apical junctions versus basal stress fibers of epithelial cells is an example of how the subcellular distribution of adaptors for different GEFs and GAPs can modulate cell differentiation (Terry et al. Citation2011). Interestingly, modulation of RhoA activity is one of the mechanisms through which cingulin and paracingulin regulate gene expression (Guillemot et al. Citation2004, Citation2008a, Guillemot and Citi Citation2006b, Citi et al. Citation2009).
A unique feature of paracingulin, that distinguishes it from cingulin, is its role in the regulation of Rac1 activation in epithelial cells. The junctional localization of the Rac1 GEF Tiam1 is reduced in paracingulin-depleted cells, and Tiam1 forms a complex with and interacts directly with paracingulin (Guillemot et al. Citation2008a). As a result, depletion of paracingulin results in decreased Rac1 activation during junction assembly, with a delay in the formation of both AJ and TJ (Guillemot et al. Citation2008a). In summary, paracingulin is a dual regulator of small GTPase activity: (1) During junction assembly (e.g., low confluence cells), it promotes Rac1 activation, by recruiting Tiam1 to junctions; and (2) when junctions are mature (e.g., high confluency monolayers), it contributes to down-regulating RhoA activity, by sequestering GEF-H1 at junctions ().
Plakophilin2
Plakophilin-2 is a member of the armadillo family of proteins, and links desmosomal cadherins to the cytoskeleton, through its interaction with desmoplakin, plakoglobin, and intermediate filaments (Bass-Zubek et al. Citation2009). Desmosomes provide strong adhesion between epithelial cells, and also regulate differentiation and homeostasis (Garrod and Chidgey Citation2008, Green et al. Citation2010). Although desmosomes are associated with intermediate filaments, desmosome assembly requires the integrity of actin microfilaments (Pasdar and Li Citation1993). Interestingly, loss of plakophilin results in changes in cortical actin organization during junction assembly, with increased stress fiber formation (Godsel et al. Citation2010). Overall RhoA activity is increased in plakophilin-2-depleted cells, but active RhoA fails to accumulate at cadherin-based junctions (Godsel et al. Citation2010). However, it is not clear whether plakophilin regulates the activity and localization of RhoA directly, by interacting with it, or indirectly, by influencing the localization or activity of either a Rho GEF or a Rho GAP.
Dynamic regulation of small GTPases at junctions
Studies on the dynamics of localization and activation of Rho family GTPases in living cells have greatly improved our understanding of the spatio-temporal pattern of their regulation. The notion that Cdc42 activation occurs upon formation of new junctions was first demonstrated using fluorescently tagged effector domains that bind to activated Cdc42 (Kim et al. Citation2000). In epithelial cells, activation of Cdc42 is required to direct the establishment of apico-basal polarity, through the Par-3/Par-6/aPKC polarity complex (Par-6 and Par-3 section) (). Cdc42 activation is regulated by the GEF Tuba and the GAP Rich1, which associate with cytoplasmic protein complexes of TJ, including ZO-1 and PATJ/Amot, and by the GEF FRG, which is activated in a src-dependent manner upon nectin engagement (JAM-A, nectins and afadin, ZO proteins and Angiomotin sections) ().
Experiments using GFP-tagged Rac1 and FRET-based biosensors were crucial to clarify how Rac1 is activated at junctions upon formation of new cadherin-based cell-cell contacts (Ehrlich et al. Citation2002, Yamada and Nelson Citation2007). Cytoplasmic Rac1 transiently accumulates at the tips of lamellipodia in isolated cells, and its association with the membrane is stabilized by the formation of new junctions. As the region of contact ‘matures’, Rac1-GFP signal progressively decreases at these sites, whereas it increases along the expanding, newest sites of cell-cell contact (Ehrlich et al. Citation2002). Significantly, both the Arp2/3 complex, an effector of Rac1, and paracingulin, a Tiam-1-interacting protein, are distributed in a manner similar to Rac1 during junction expansion (Yamada and Nelson Citation2007, Paschoud et al. Citation2010). The relocalization of Rac1 to the edges of the cell-cell contact area, where it promotes further E-cadherin accumulation, generates a positive feedback loop that expands the area of cell-cell contact. The major Rac1 activator in epithelial cells is Tiam1, and it is recruited to junctions and regulated by paracingulin, Par-3 and phosphatidylinositol-3-phosphates (Rac1, Par-6 and Par-3 and Cingulin and paracingulin sections) (). Interestingly, although new junctional GAPs are being identified (Matsuda et al. Citation2008), it is unclear which Rac1-specific GAP is operating at epithelial junctions. New junction formation also requires the activation of Rap1, which is regulated by GEFs and GAPs that associate with E-cadherin, JAM-A, afadin, β-catenin and MAGI (MAGI1, JAM-A, nectins and afadin and E-cadherin, β-catenin and α-catenin sections) ().
Activated RhoA specifically accumulates at the expanding edges of cell-cell contact, and not in the center of these areas, and drives the myosinII-dependent, lateral expansion of the junction (Yamada and Nelson Citation2007). Accordingly, cycling of RhoA between active and inactive forms, which promotes the contractility of actomyosin filaments, must occur at these sites. Significantly, cingulin and paracingulin, adaptor molecules for the Rho GEFs, GEF-H1 and p114RhoGEF (), accumulate at the edges of the expanding regions of cell-cell contact during junction formation in living cells (Paschoud et al. Citation2010). Concerning the mechanisms through which RhoA is inactivated at junctions, a role could be played by the cytoplasmic protein complex associated with E-cadherin, since the Rho GAPs p190 and ARHGAP associate with p120ctn and α-catenin, respectively (). However, whether p120ctn stimulates or inhibits RhoA activity in epithelial cells is not clear, since the outcome of p120ctn depletion or overexpression experiments appears to be cell-context dependent (p120catenin and E-cadherin, β-catenin and α-catenin sections) ().
Conclusions and perspectives
Regulation of Rho family GTPases is of paramount importance in coordinating fundamental cellular activities, such as cytoskeletal organization, cell migration, and cell adhesion and proliferation (). This regulation is achieved through a complex network of activators and inhibitors, which bind to specific junctional adaptors. Thus, the expression, modulation and subcellular localization of such regulatory molecules is critical in shaping the cell-context-dependent signaling output.
Epithelial junctions constitute specific, spatially restricted signaling modules for Rho family GTPases, since several transmembrane and cytoplasmic proteins belonging to TJ and AJ act either as regulators or targets of Rho family GTPases (). For most of these proteins, specific interactions with GTPases, GEFs and GAPs have been demonstrated, providing an insight onto the molecular mechanisms of GTPase regulation. However, additional antibody and live imaging experimental tools to clarify the localization of GEFs and GAPs in fixed and living cells will be required to clarify the spatio-temporal dynamics of their interaction with junctional proteins.
Another important challenge for future studies will be to determine the relevance of GEFs, GAPs and their interacting junctional proteins not only in cultured cell model systems, but also at the whole organism level. Considering the large number of GEFs and GAPs that have been identified by genome sequence analysis, functional redundancies must be carefully examined, before definitive conclusions are drawn on the physiological roles of GEFs, GAPs, and their adaptor molecules.
Another key question concerns the mechanisms underlying the interactions between GEFs, GAPs and their adaptor molecules. How do junctional proteins, most of which exist in an equilibrium between junction-associated and cytoplasmic soluble pools (Shen et al. Citation2008a), activate or inactivate GEFs and GAPs? Do they bind to GEFs and GAPs only when they are associated with their partner molecules at junctions? Do they modulate the function of GEFs and GAPs by affecting their conformation or phosphorylation status? Or do they function mainly by sequestering GEFs and GAPs to a specific subcellular compartment?
Finally, a critical issue for future studies is how the mutually antagonistic cross-talk of GTPases is generated at junctions. In the Xenopus laevis cell wounding model, segregation of the zones of RhoA and Cdc42 activity depends on Abr, a Rho GEF that also contains a Cdc42 GAP domain (Benink and Bement Citation2005, Vaughan et al. Citation2011). However, such a GEF is unusual, and has not been localized at mammalian junctions. Thus, additional studies on the functional cross-talk of GEFs and GAPs, and the role of adaptor proteins in this cross-talk, are necessary to address these questions, and provide insights on the molecular basis mechanisms of small GTPase regulation at junctions.
Acknowledgements
We are grateful to the State of Geneva and the Swiss National Funds for supporting our research and to Nicolas Roggli for graphics help and advice. We apologize for not being able to cite several original articles, due to space limitations.
Declaration of interest: The authors report no conflicts of interest. The authors alone are responsible for the content and writing of the paper.
References
- Aase K, Ernkvist M, Ebarasi L, Jakobsson L, Majumdar A, Yi C, Birot O, Ming Y, Kvanta A, Edholm D, Aspenstrom P, Kissil J, Claesson-Welsh L, Shimono A, Holmgren L. 2007. Angiomotin regulates endothelial cell migration during embryonic angiogenesis. Genes Dev 21:2055–2068.
- Aijaz S, D'atri F, Citi S, Balda MS, Matter K. 2005. Binding of GEF-H1 to the tight junction-associated adaptor cingulin results in inhibition of Rho signaling and G1/S phase transition. Dev Cell 8:777–786.
- Amano M, Nakayama M, Kaibuchi K. 2010. Rho-kinase/ROCK: A key regulator of the cytoskeleton and cell polarity. Cytoskeleton (Hoboken) 67:545–554.
- Anastasiadis PZ, Moon SY, Thoreson MA, Mariner DJ, Crawford HC, Zheng Y, Reynolds AB. 2000. Inhibition of RhoA by p120 catenin. Nat Cell Biol 2:637–644.
- Anastasiadis PZ, Reynolds AB. 2001. Regulation of Rho GTPases by p120-catenin. Curr Opin Cell Biol 13:604–610.
- Arimura N, Menager C, Kawano Y, Yoshimura T, Kawabata S, Hattori A, Fukata Y, Amano M, Goshima Y, Inagaki M, Morone N, Usukura J, Kaibuchi K. 2005. Phosphorylation by Rho kinase regulates CRMP-2 activity in growth cones. Mol Cell Biol 25:9973–9984.
- Asha H, De Ruiter ND, Wang MG, Hariharan IK. 1999. The Rap1 GTPase functions as a regulator of morphogenesis in vivo. EMBO J 18:605–615.
- Bass-Zubek AE, Godsel LM, Delmar M, Green KJ. 2009. Plakophilins: Multifunctional scaffolds for adhesion and signaling. Curr Opin Cell Biol 21:708–716.
- Benais-Pont G, Punn A, Flores-Maldonado C, Eckert J, Raposo G, Fleming TP, Cereijido M, Balda MS, Matter K. 2003. Identification of a tight junction-associated guanine nucleotide exchange factor that activates Rho and regulates paracellular permeability. J Cell Biol 160:729–740.
- Benink HA, Bement WM. 2005. Concentric zones of active RhoA and Cdc42 around single cell wounds. J Cell Biol 168:429–439.
- Benitah SA, Valeron PF, Van Aelst L, Marshall CJ, Lacal JC. 2004. Rho GTPases in human cancer: An unresolved link to upstream and downstream transcriptional regulation. Biochim Biophys Acta 1705:121–132.
- Bernards A, Settleman J. 2005. GAPs in growth factor signalling. Growth Factors 23:143–149.
- Boettner B, Govek EE, Cross J, Van Aelst L. 2000. The junctional multidomain protein AF-6 is a binding partner of the Rap1A GTPase and associates with the actin cytoskeletal regulator profilin. Proc Natl Acad Sci USA 97:9064–9069.
- Boettner B, Van Aelst L. 2009. Control of cell adhesion dynamics by Rap1 signaling. Curr Opin Cell Biol 21:684–693.
- Bourguignon LY, Zhu H, Shao L, Chen YW. 2000. CD44 interaction with tiam1 promotes Rac1 signaling and hyaluronic acid-mediated breast tumor cell migration. J Biol Chem 275:1829–1838.
- Brady DC, Alan JK, Madigan JP, Fanning AS, Cox AD. 2009. The transforming Rho family GTPase Wrch-1 disrupts epithelial cell tight junctions and epithelial morphogenesis. Mol Cell Biol 29:1035–1049.
- Braga VM, Machesky LM, Hall A, Hotchin NA. 1997. The small GTPases Rho and Rac are required for the establishment of cadherin-dependent cell-cell contacts. J Cell Biol 137:1421–1431.
- Braga VM, Yap AS. 2005. The challenges of abundance: Epithelial junctions and small GTPase signalling. Curr Opin Cell Biol 17:466–474.
- Bratt A, Birot O, Sinha I, Veitonmaki N, Aase K, Ernkvist M, Holmgren L. 2005. Angiomotin regulates endothelial cell-cell junctions and cell motility. J Biol Chem 280:34859–34869.
- Bratt A, Wilson WJ, Troyanovsky B, Aase K, Kessler R, Van Meir EG, Holmgren L. 2002. Angiomotin belongs to a novel protein family with conserved coiled-coil and PDZ binding domains. Gene 298:69–77.
- Bruewer M, Hopkins AM, Hobert ME, Nusrat A, Madara JL. 2004. RhoA, Rac1, and Cdc42 exert distinct effects on epithelial barrier via selective structural and biochemical modulation of junctional proteins and F-actin. Am J Physiol Cell Physiol 287:C327–335.
- Bryant DM, Datta A, Rodriguez-Fraticelli AE, Peranen J, Martin-Belmonte F, Mostov KE. 2010. A molecular network for de novo generation of the apical surface and lumen. Nat Cell Biol 12:1035–1045.
- Busche S, Kremmer E, Posern G. 2010. E-cadherin regulates MAL-SRF-mediated transcription in epithelial cells. J Cell Sci 123:2803–2809.
- Calautti E, Grossi M, Mammucari C, Aoyama Y, Pirro M, Ono Y, Li J, Dotto GP. 2002. Fyn tyrosine kinase is a downstream mediator of Rho/PRK2 function in keratinocyte cell-cell adhesion. J Cell Biol 156:137–148.
- Chen X, Macara IG. 2005. Par-3 controls tight junction assembly through the Rac exchange factor Tiam1. Nat Cell Biol 7:262–269.
- Chen L, Necela BM, Su W, Yanagisawa M, Anastasiadis PZ, Fields AP, Thompson EA. 2006. Peroxisome proliferator-activated receptor gamma promotes epithelial to mesenchymal transformation by Rho GTPase-dependent activation of ERK1/2. J. Biol Chem 281:24575–24587.
- Cho YJ, Cunnick JM, Yi SJ, Kaartinen V, Groffen J, Heisterkamp N. 2007. Abr and Bcr, two homologous Rac GTPase-activating proteins, control multiple cellular functions of murine macrophages. Mol Cell Biol 27:899–911.
- Citi S, Paschoud S, Pulimeno P, Timolati F, De Robertis F, Jond L, Guillemot L. 2009. The tight junction protein cingulin regulates gene expression and RhoA signalling. Ann NY Acad Sci 1165:88–98.
- Citi S, Sabanay H, Jakes R, Geiger B, Kendrick-Jones J. 1988. Cingulin, a new peripheral component of tight junctions. Nature 333:272–276.
- Citi S, Sabanay H, Kendrick-Jones J, Geiger B. 1989. Cingulin: Characterization and localization. J Cell Sci 93:107–122.
- Coleman ML, Marshall CJ, Olson MF. 2004. RAS and RHO GTPases in G1-phase cell-cycle regulation. Nat Rev Mol Cell Biol 5:355–366.
- Cordenonsi M, D'atri F, Hammar E, Parry DA, Kendrick-Jones J, Shore D, Citi S. 1999. Cingulin contains globular and coiled-coil domains and interacts with ZO-1, ZO-2, ZO-3, and myosin. J Cell Biol 147:1569–1582.
- Cottonham CL, Kaneko S, Xu L. 2010. miR-21 and miR-31 converge on TIAM1 to regulate migration and invasion of colon carcinoma cells. J Biol Chem 285:35293–35302.
- Cozzolino M, Stagni V, Spinardi L, Campioni N, Fiorentini C, Salvati E, Alema S, Salvatore AM. 2003. p120 Catenin is required for growth factor-dependent cell motility and scattering in epithelial cells. Mol Biol Cell 14:1964–1977.
- Daub H, Gevaert K, Vandekerckhove J, Sobel A, Hall A. 2001. Rac/Cdc42 and p65PAK regulate the microtubule-destabilizing protein stathmin through phosphorylation at serine 16. J Biol Chem 276:1677–1680.
- Davis MA, Reynolds AB. 2006. Blocked acinar development, E-cadherin reduction, and intraepithelial neoplasia upon ablation of p120-catenin in the mouse salivary gland. Dev Cell 10:21–31.
- Dermardirossian C, Bokoch GM. 2005. GDIs: Central regulatory molecules in Rho GTPase activation. Trends Cell Biol 15:356–363.
- Diamond JM. 1977. The epithelial junction: Bridge, gate and fence. Physiologist 20:10–18.
- Dobrosotskaya IY, James GLL. 2000. MAGI-1 interacts with beta-catenin and is associated with cell-cell adhesion structures. Biochem Biophys Res Commun 270:903–909.
- Dohn MR, Brown MV, Reynolds AB. 2009. An essential role for p120-catenin in Src- and Rac1-mediated anchorage-independent cell growth. J Cell Biol 184:437–450.
- Drees F, Pokutta S, Yamada S, Nelson WJ, Weis WI. 2005. Alpha-catenin is a molecular switch that binds E-cadherin-beta-catenin and regulates actin-filament assembly. Cell 123:903–915.
- Dube N, Kooistra MR, Pannekoek WJ, Vliem MJ, Oorschot V, Klumperman J, Rehmann H, Bos JL. 2008. The RapGEF PDZ-GEF2 is required for maturation of cell-cell junctions. Cell Signal 20:1608–1615.
- Dupont S, Morsut L, Aragona M, Enzo E, Giulitti S, Cordenonsi M, Zanconato F, Le Digabel J, Forcato M, Bicciato S, Elvassore N, Piccolo S. 2011. Role of YAP/TAZ in mechanotransduction. Nature 474:179–183.
- Ebnet K, Schulz CU, Meyer Zu Brickwedde MK, Pendl GG, Vestweber D. 2000. Junctional Adhesion Molecule (JAM) interacts with the PDZ domain containing proteins AF-6 and ZO-1. J Biol Chem 275:27979–27988.
- Ebnet K, Suzuki A, Horikoshi Y, Hirose T, Meyer Zu Brickwedde MK, Ohno S, Vestweber D. 2001. The cell polarity protein ASIP/PAR-3 directly associates with junctional adhesion molecule (JAM). Embo J 20:3738–3748.
- Edwards DC, Sanders LC, Bokoch GM, Gill GN. 1999. Activation of LIM-kinase by Pak1 couples Rac/Cdc42 GTPase signalling to actin cytoskeletal dynamics. Nat Cell Biol 1:253–259.
- Ehrlich JS, Hansen MD, Nelson WJ. 2002. Spatio-temporal regulation of Rac1 localization and lamellipodia dynamics during epithelial cell-cell adhesion. Dev Cell 3:259–270.
- Erasmus J, Aresta S, Nola S, Caron E, Braga VM. 2010. Newly formed E-cadherin contacts do not activate Cdc42 or induce filopodia protrusion in human keratinocytes. Biol Cell 102:13–24.
- Ernkvist M, Luna Persson N, Audebert S, Lecine P, Sinha I, Liu M, Schlueter M, Horowitz A, Aase K, Weide T, Borg JP, Majumdar A, Holmgren L. 2009. The Amot/Patj/Syx signaling complex spatially controls RhoA GTPase activity in migrating endothelial cells. Blood 113:244–253.
- Espejo R, Rengifo-Cam W, Schaller MD, Evers BM, Sastry SK. 2010. PTP-PEST controls motility, adherens junction assembly, and Rho GTPase activity in colon cancer cells. Am J Physiol Cell Physiol 299:C454–463.
- Fanning AS, Anderson JM. 2009. Zonula occludens-1 and -2 are cytosolic scaffolds that regulate the assembly of cellular junctions. Ann NY Acad Sci 1165:113–120.
- Fanning AS, Jameson BJ, Jesaitis LA, Anderson JM. 1998. The tight junction protein ZO-1 establishes a link between the transmembrane protein occludin and the actin cytoskeleton. J Biol Chem 273:29745–29753.
- Farquhar MG, Palade GE. 1963. Junctional complexes in various epithelia. J Cell Biol 17:375–412.
- Fukata M, Kaibuchi K. 2001. Rho-family GTPases in cadherin-mediated cell-cell adhesion. Nat Rev Mol Cell Biol 2:887–897.
- Fukata M, Kuroda S, Nakagawa M, Kawajiri A, Itoh N, Shoji I, Matsuura Y, Yonehara S, Fujisawa H, Kikuchi A, Kaibuchi K. 1999. Cdc42 and Rac1 regulate the interaction of IQGAP1 with beta-catenin. J Biol Chem 274:26044–26050.
- Fukata M, Watanabe T, Noritake J, Nakagawa M, Yamaga M, Kuroda S, Matsuura Y, Iwamatsu A, Perez F, Kaibuchi K. 2002. Rac1 and Cdc42 capture microtubules through IQGAP1 and CLIP-170. Cell 109:873–885.
- Fukuhara A, Shimizu K, Kawakatsu T, Fukuhara T, Takai Y. 2003. Involvement of nectin-activated Cdc42 small G protein in organization of adherens and tight junctions in Madin-Darby canine kidney cells. J Biol Chem 278:51885–51893.
- Fukuhara T, Shimizu K, Kawakatsu T, Fukuyama T, Minami Y, Honda T, Hoshino T, Yamada T, Ogita H, Okada M, Takai Y. 2004. Activation of Cdc42 by trans interactions of the cell adhesion molecules nectins through c-Src and Cdc42-GEF FRG. J Cell Biol 166:393–405.
- Fukumoto Y, Kaibuchi K, Hori Y, Fujioka H, Araki S, Ueda T, Kikuchi A, Takai Y. 1990. Molecular cloning and characterization of a novel type of regulatory protein (GDI) for the rho proteins, ras p21-like small GTP-binding proteins. Oncogene 5:1321–1328.
- Fukuyama T, Ogita H, Kawakatsu T, Fukuhara T, Yamada T, Sato T, Shimizu K, Nakamura T, Matsuda M, Takai Y. 2005. Involvement of the c-Src-Crk-C3G-Rap1 signaling in the nectin-induced activation of Cdc42 and formation of adherens junctions. J Biol Chem 280:815–825.
- Fukuyama T, Ogita H, Kawakatsu T, Inagaki M, Takai Y. 2006. Activation of Rac by cadherin through the c-Src-Rap1-phosphatidylinositol 3-kinase-Vav2 pathway. Oncogene 25:8–19.
- Furuse M. 2009. Knockout animals and natural mutations as experimental and diagnostic tool for studying tight junction functions in vivo. Biochim Biophys Acta 1788:813–819.
- Garrod D, Chidgey M. 2008. Desmosome structure, composition and function. Biochim Biophys Acta 1778:572–587.
- Glaven JA, Whitehead I, Bagrodia S, Kay R, Cerione RA. 1999. The Dbl-related protein, Lfc, localizes to microtubules and mediates the activation of Rac signaling pathways in cells. J Biol Chem 274:2279–2285.
- Godsel LM, Dubash AD, Bass-Zubek AE, Amargo EV, Klessner JL, Hobbs RP, Chen X, Green KJ. 2010. Plakophilin 2 couples actomyosin remodeling to desmosomal plaque assembly via RhoA. Mol Biol Cell 21:2844–2859.
- Gonzalez-Mariscal L, Betanzos A, Nava P, Jaramillo BE. 2003. Tight junction proteins. Prog Biophys Mol Biol 81:1–44.
- Goodwin M, Kovacs EM, Thoreson MA, Reynolds AB, Yap AS. 2003. Minimal mutation of the cytoplasmic tail inhibits the ability of E-cadherin to activate Rac but not phosphatidylinositol 3-kinase: Direct evidence of a role for cadherin-activated Rac signaling in adhesion and contact formation. J Biol Chem 278:20533–20539.
- Gopalakrishnan S, Dunn KW, Marrs JA. 2002. Rac1, but not RhoA, signaling protects epithelial adherens junction assembly during ATP depletion. Am J Physiol Cell Physiol 283:C261–272.
- Gopalakrishnan S, Raman N, Atkinson SJ, Marrs JA. 1998. Rho GTPase signaling regulates tight junction assembly and protects tight junctions during ATP depletion. Am J Physiol 275:C798–809.
- Green KJ, Getsios S, Troyanovsky S, Godsel LM. 2010. Intercellular junction assembly, dynamics, and homeostasis. Cold Spring Harb Perspect Biol 2:a000125.
- Grosheva I, Shtutman M, Elbaum M, Bershadsky AD. 2001. p120 catenin affects cell motility via modulation of activity of Rho-family GTPases: A link between cell-cell contact formation and regulation of cell locomotion. J Cell Sci 114:695–707.
- Guillemot L, Citi S. 2006a. Cingulin regulates claudin-2 expression and cell proliferation through the small GTPase RhoA. Mol Biol Cell 17:3569–3577.
- Guillemot L, Citi S. 2006b. Cingulin, a cytoskeleton-associated protein of the tight junction. In: Gonzalez-Mariscal L, editor. Tight junctions. New York: Landes Bioscience-Springer Science.
- Guillemot L, Hammar E, Kaister C, Ritz J, Caille D, Jond L, Bauer C, Meda P, Citi S. 2004. Disruption of the cingulin gene does not prevent tight junction formation but alters gene expression. J Cell Sci 117:5245–5256.
- Guillemot L, Paschoud S, Jond L, Foglia A, Citi S. 2008a. Paracingulin regulates the activity of Rac1 and RhoA GTPases by recruiting Tiam1 and GEF-H1 to epithelial junctions. Mol Biol Cell 19:4442–4453.
- Guillemot L, Paschoud S, Pulimeno P, Foglia A, Citi S. 2008b. The cytoplasmic plaque of tight junctions: A scaffolding and signalling center. Biochim Biophys Acta 1778:601–613.
- Gumbiner BM. 2005. Regulation of cadherin-mediated adhesion in morphogenesis. Nat Rev Mol Cell Biol 6:622–634.
- Haegel H, Larue L, Ohsugi M, Fedorov L, Herrenknecht K, Kemler R. 1995. Lack of beta-catenin affects mouse development at gastrulation. Development 121:3529–3537.
- Heuberger J, Birchmeier W. 2010. Interplay of cadherin-mediated cell adhesion and canonical Wnt signaling. Cold Spring Harb Perspect Biol 2:a002915.
- Hirabayashi S, Hata Y. 2006. JAM family proteins: Tight junction proteins that belong to the immunoglobulin superfamily. In: Gonzalez-Mariscal L, editor. Tight junctions. Georgetown, TX: Landes Biosciences.
- Hirase T, Kawashima S, Wong EY, Ueyama T, Rikitake Y, Tsukita S, Yokoyama M, Staddon JM. 2001. Regulation of tight junction permeability and occludin phosphorylation by Rhoa-p160ROCK-dependent and -independent mechanisms. J Biol Chem 276:10423–10431.
- Hogan C, Serpente N, Cogram P, Hosking CR, Bialucha CU, Feller SM, Braga VM, Birchmeier W, Fujita Y. 2004. Rap1 regulates the formation of E-cadherin-based cell-cell contacts. Mol Cell Biol 24:6690–6700.
- Hordijk PL, Ten Klooster JP, Van Der Kammen RA, Michiels F, Oomen LC, Collard JG. 1997. Inhibition of invasion of epithelial cells by Tiam1-Rac signaling. Science 278:1464–1466.
- Ide N, Hata Y, Nishioka H, Hirao K, Yao I, Deguchi M, Mizoguchi A, Nishimori H, Tokino T, Nakamura Y, Takai Y. 1999. Localization of membrane-associated guanylate kinase (MAGI)-1/BAI-associated protein (BAP) 1 at tight junctions of epithelial cells. Oncogene 18:7810–7815.
- Ikeda W, Nakanishi H, Miyoshi J, Mandai K, Ishizaki H, Tanaka M, Togawa A, Takahashi K, Nishioka H, Yoshida H, Mizoguchi A, Nishikawa S, Takai Y. 1999. Afadin: A key molecule essential for structural organization of cell-cell junctions of polarized epithelia during embryogenesis. J Cell Biol 146:1117–1132.
- Ikenouchi J, Umeda K, Tsukita S, Furuse M, Tsukita S. 2007. Requirement of ZO-1 for the formation of belt-like adherens junctions during epithelial cell polarization. J Cell Biol 176:779–786.
- Ishizaki T, Morishima Y, Okamoto M, Furuyashiki T, Kato T, Narumiya S. 2001. Coordination of microtubules and the actin cytoskeleton by the Rho effector mDia1. Nat Cell Biol 3:8–14.
- Itoh M, Sasaki H, Furuse M, Ozaki H, Kita T, Tsukita S. 2001. Junctional adhesion molecule (JAM) binds to PAR-3: A possible mechanism for the recruitment of PAR-3 to tight junctions. J Cell Biol 154:491–497.
- Izumi Y, Hirose T, Tamai Y, Hirai S, Nagashima Y, Fujimoto T, Tabuse Y, Kemphues KJ, Ohno S. 1998. An atypical PKC directly associates and colocalizes at the epithelial tight junction with ASIP, a mammalian homologue of Caenorhabditis elegans polarity protein PAR-3. J Cell Biol 143:95–106.
- Jaffe AB, Hall A. 2005. Rho GTPases: Biochemistry and biology. Annu Rev Cell Dev Biol 21:247–269.
- Joberty G, Petersen C, Gao L, Macara IG. 2000. The cell-polarity protein Par6 links Par3 and atypical protein kinase C to Cdc42. Nat Cell Biol 2:531–539.
- Johansson A, Driessens M, Aspenstrom P. 2000. The mammalian homologue of the Caenorhabditis elegans polarity protein PAR-6 is a binding partner for the Rho GTPases Cdc42 and Rac1. J Cell Sci 113:3267–3275.
- Jou TS, Nelson WJ. 1998. Effects of regulated expression of mutant RhoA and Rac1 small GTPases on the development of epithelial (MDCK) cell polarity. J Cell Biol 142:85–100.
- Jou TS, Schneeberger EE, Nelson WJ. 1998. Structural and functional regulation of tight junctions by RhoA and Rac1 small GTPases. J Cell Biol 142:101–115.
- Katsuno T, Umeda K, Matsui T, Hata M, Tamura A, Itoh M, Takeuchi K, Fujimori T, Nabeshima YI, Noda T, Tsukita S, Tsukita S. 2008. Deficiency of zonula occludens-1 causes embryonic lethal phenotype associated with defected yolk sac angiogenesis and apoptosis of embryonic cells. Mol Biol Cell 19:2465–2475.
- Katzav S, Cleveland JL, Heslop HE, Pulido D. 1991. Loss of the amino-terminal helix-loop-helix domain of the vav proto-oncogene activates its transforming potential. Mol Cell Biol 11:1912–1920.
- Kawakatsu T, Shimizu K, Honda T, Fukuhara T, Hoshino T, Takai Y. 2002. Trans-interactions of nectins induce formation of filopodia and Lamellipodia through the respective activation of Cdc42 and Rac small G proteins. J Biol Chem 277:50749–50755.
- Kemphues KJ, Priess JR, Morton DG, Cheng NS. 1988. Identification of genes required for cytoplasmic localization in early C. elegans embryos. Cell 52:311–320.
- Kim SH, Li Z, Sacks DB. 2000. E-cadherin-mediated cell-cell attachment activates Cdc42. J Biol Chem 275:36999–7005.
- Knox AL, Brown NH. 2002. Rap1 GTPase regulation of adherens junction positioning and cell adhesion. Science 295:1285–1288.
- Kodama A, Takaishi K, Nakano K, Nishioka H, Takai Y. 1999. Involvement of Cdc42 small G protein in cell-cell adhesion, migration and morphology of MDCK cells. Oncogene 18:3996–4006.
- Kooistra MR, Dube N, Bos JL. 2007. Rap1: A key regulator in cell-cell junction formation. J Cell Sci 120:17–22.
- Kovacs EM, Ali RG, McCormack AJ, Yap AS. 2002. E-cadherin homophilic ligation directly signals through Rac and phosphatidylinositol 3-kinase to regulate adhesive contacts. J Biol Chem 277:6708–6718.
- Krendel M, Zenke FT, Bokoch GM. 2002. Nucleotide exchange factor GEF-H1 mediates cross-talk between microtubules and the actin cytoskeleton. Nat Cell Biol 4:294–301.
- Kroschewski R, Hall A, Mellman I. 1999. Cdc42 controls secretory and endocytic transport to the basolateral plasma membrane of MDCK cells. Nat Cell Biol 1:8–13.
- Kuriyama M, Harada N, Kuroda S, Yamamoto T, Nakafuku M, Iwamatsu A, Yamamoto D, Prasad R, Croce C, Canaani E, Kaibuchi K. 1996. Identification of AF-6 and canoe as putative targets for Ras. J Biol Chem 271:607–610.
- Kuroda S, Fukata M, Nakagawa M, Fujii K, Nakamura T, Ookubo T, Izawa I, Nagase T, Nomura N, Tani H, Shoji I, Matsuura Y, Yonehara S, Kaibuchi K. 1998. Role of IQGAP1, a target of the small GTPases Cdc42 and Rac1, in regulation of E-cadherin- mediated cell-cell adhesion. Science 281:832–835.
- Lambert JM, Lambert QT, Reuther GW, Malliri A, Siderovski DP, Sondek J, Collard JG, Der CJ. 2002. Tiam1 mediates Ras activation of Rac by a PI(3)K-independent mechanism. Nat Cell Biol 4:621–625.
- Larue L, Ohsugi M, Hirchenhain J, Kemler R. 1994. E-cadherin null mutant embryos fail to form a trophectoderm epithelium. Proc Natl Acad Sci USA 91:8263–8267.
- Laukoetter MG, Nava P, Lee WY, Severson EA, Capaldo CT, Babbin BA, Williams IR, Koval M, Peatman E, Campbell JA, Dermody TS, Nusrat A, Parkos CA. 2007. JAM-A regulates permeability and inflammation in the intestine in vivo. J Exp Med 204:3067–3076.
- Lin D, Edwards AS, Fawcett JP, Mbamalu G, Scott JD, Pawson T. 2000. A mammalian PAR-3-PAR-6 complex implicated in Cdc42/Rac1 and aPKC signalling and cell polarity. Nat Cell Biol 2:540–547.
- Liu BP, Chrzanowska-Wodnicka M, Burridge K. 1998. Microtubule depolymerization induces stress fibers, focal adhesions, and DNA synthesis via the GTP-binding protein Rho. Cell Adhes Commun 5:249–255.
- Liu Y, Nusrat A, Schnell FJ, Reaves TA, Walsh S, Pochet M, Parkos CA. 2000. Human junction adhesion molecule regulates tight junction resealing in epithelia. J Cell Sci 113:2363–2374.
- Maekawa M, Ishizaki T, Boku S, Watanabe N, Fujita A, Iwamatsu A, Obinata T, Ohashi K, Mizuno K, Narumiya S. 1999. Signaling from Rho to the actin cytoskeleton through protein kinases ROCK and LIM-kinase. Science 285:895–898.
- Malliri A, Van Es S, Huveneers S, Collard JG. 2004. The Rac exchange factor Tiam1 is required for the establishment and maintenance of cadherin-based adhesions. J Biol Chem 279:30092–30098.
- Mandai K, Nakanishi H, Satoh A, Obaishi H, Wada M, Nishioka H, Itoh M, Mizoguchi A, Aoki T, Fujimoto T, Matsuda Y, Tsukita S, Takai Y. 1997. Afadin: A novel actin filament-binding protein with one PDZ domain localized at cadherin-based cell-to-cell adherens junction. J Cell Biol 139:517–528.
- Mandell KJ, Babbin BA, Nusrat A, Parkos CA. 2005. Junctional adhesion molecule 1 regulates epithelial cell morphology through effects on beta1 integrins and Rap1 activity. J Biol Chem 280:11665–11674.
- Marciano DK, Brakeman PR, Lee CZ, Spivak N, Eastburn DJ, Bryant DM, Beaudoin GM 3rd, Hofmann I, Mostov KE, Reichardt LF. 2011. p120 catenin is required for normal renal tubulogenesis and glomerulogenesis. Development 138:2099–2109.
- Martin-Padura I, Lostaglio S, Schneemann M, Williams L, Romano M, Fruscella P, Panzeri C, Stoppacciaro A, Ruco L, Villa A, Simmons D, Dejana E. 1998. Junctional adhesion molecule: A novel member of the immunoglobulin superfamily that distributes at intercellular junctions and modulates monocyte transmigration. J Cell Biol 142:117–127.
- Masuda S, Oda Y, Sasaki H, Ikenouchi J, Higashi T, Akashi M, Nishi E, Furuse M. 2011. LSR defines cell corners for tricellular tight junction formation in epithelial cells. J Cell Sci 124:548–555.
- Matsuda M, Kobayashi Y, Masuda S, Adachi M, Watanabe T, Yamashita JK, Nishi E, Tsukita S, Furuse M. 2008. Identification of adherens junction-associated GTPase activating proteins by the fluorescence localization-based expression cloning. Exp Cell Res 314:939–949.
- McCrea PD, Gu D, Balda MS. 2009. Junctional music that the nucleus hears: Cell-cell contact signaling and the modulation of gene activity. Cold Spring Harb Perspect Biol. 1:a002923.
- Mege RM, Gavard J, Lambert M. 2006. Regulation of cell-cell junctions by the cytoskeleton. Curr Opin Cell Biol 18:541–548.
- Meller N, Merlot S, Guda C. 2005. CZH proteins: A new family of Rho-GEFs. J Cell Sci 118:4937–4946.
- Meng W, Takeichi M.2009. Adherens junction: Molecular architecture and regulation. Cold Spring Harbor Perspect Biol (DOI:10.1101/cshperspect.a002899).
- Mertens AE, Rygiel TP, Olivo C, Van Der Kammen R, Collard JG. 2005. The Rac activator Tiam1 controls tight junction biogenesis in keratinocytes through binding to and activation of the Par polarity complex. J Cell Biol 170:1029–1037.
- Michiels F, Stam JC, Hordijk PL, Van Der Kammen RA, Ruuls-Van Stalle L, Feltkamp CA, Collard JG. 1997. Regulated membrane localization of Tiam1, mediated by the NH2-terminal pleckstrin homology domain, is required for Rac-dependent membrane ruffling and C-Jun NH2-terminal kinase activation. J Cell Biol 137:387–398.
- Mino A, Ohtsuka T, Inoue E, Takai Y. 2000. Membrane-associated guanylate kinase with inverted orientation (MAGI)-1/brain angiogenesis inhibitor 1-associated protein (BAP1) as a scaffolding molecule for Rap small G protein GDP/GTP exchange protein at tight junctions. Genes Cells 5:1009–1016.
- Miralles F, Posern G, Zaromytidou AI, Treisman R. 2003. Actin dynamics control SRF activity by regulation of its coactivator MAL. Cell 113:329–342.
- Mitic LL, Anderson JM. 1998. Molecular architecture of tight junctions. Annu Rev Physiol 60:121–142.
- Moon SY, Zheng Y. 2003. Rho GTPase-activating proteins in cell regulation. Trends Cell Biol 13:13–22.
- Muroya K, Kawasaki Y, Hayashi T, Ohwada S, Akiyama T. 2007. PH domain-mediated membrane targeting of Asef. Biochem Biophys Res Commun 355:85–88.
- Nakagawa M, Fukata M, Yamaga M, Itoh N, Kaibuchi K. 2001. Recruitment and activation of Rac1 by the formation of E-cadherin-mediated cell-cell adhesion sites. J Cell Sci 114:1829–1838.
- Nakayama M, Goto TM, Sugimoto M, Nishimura T, Shinagawa T, Ohno S, Amano M, Kaibuchi K. 2008. Rho-kinase phosphorylates PAR-3 and disrupts PAR complex formation. Dev Cell 14:205–215.
- Nimnual AS, Taylor LJ, Bar-Sagi D. 2003. Redox-dependent downregulation of Rho by Rac. Nat Cell Biol 5:236–241.
- Nishimura T, Yamaguchi T, Kato K, Yoshizawa M, Nabeshima Y, Ohno S, Hoshino M, Kaibuchi K. 2005. PAR-6-PAR-3 mediates Cdc42-induced Rac activation through the Rac GEFs STEF/Tiam1. Nat Cell Biol 7:270–277.
- Noren NK, Liu BP, Burridge K, Kreft B. 2000. p120 catenin regulates the actin cytoskeleton via Rho family GTPases. J Cell Biol 150:567–580.
- Nusrat A, Giry M, Turner JR, Colgan SP, Parkos CA, Carmes D, Lemichez E, Boquet P, Madara JL. 1995. Rho protein regulates tight junctions and perijunctional actin organization in polarized epithelia. Proc Natl Acad Sci USA 92:10629–10633.
- Ohnishi H, Nakahara T, Furuse K, Sasaki H, Tsukita S, Furuse M. 2004. JACOP, a novel plaque protein localizing at the apical junctional complex with sequence similarity to cingulin. J Biol Chem 279:46014–46022.
- Ohno S. 2001. Intercellular junctions and cellular polarity: The PAR-aPKC complex, a conserved core cassette playing fundamental roles in cell polarity. Curr Opin Cell Biol 13:641–648.
- Olofsson B. 1999. Rho guanine dissociation inhibitors: Pivotal molecules in cellular signalling. Cell Signal 11:545–554.
- Otani T, Ichii T, Aono S, Takeichi M. 2006. Cdc42 GEF Tuba regulates the junctional configuration of simple epithelial cells. J Cell Biol 175:135–146.
- Paschoud S, Yu D, Pulimeno P, Jond L, Turner JR, Citi S. 2010. Cingulin and paracingulin show similar dynamic behaviour, but are recruited independently to junctions. Mol Membr Biol 28:123–135.
- Pasdar M, Li Z. 1993. Disorganization of microfilaments and intermediate filaments interferes with the assembly and stability of desmosomes in MDCK epithelial cells. Cell Motil Cytoskel 26:163–180.
- Patel S, Takagi KI, Suzuki J, Imaizumi A, Kimura T, Mason RM, Kamimura T, Zhang Z. 2005. RhoGTPase activation is a key step in renal epithelial mesenchymal transdifferentiation. J Am Soc Nephrol 16:1977–1984.
- Pece S, Chiariello M, Murga C, Gutkind JS. 1999. Activation of the protein kinase Akt/PKB by the formation of E-cadherin-mediated cell-cell junctions. Evidence for the association of phosphatidylinositol 3-kinase with the E-cadherin adhesion complex. J Biol Chem 274:19347–19351.
- Perez-Moreno M, Davis MA, Wong E, Pasolli HA, Reynolds AB, Fuchs E. 2006. p120-catenin mediates inflammatory responses in the skin. Cell 124:631–644.
- Potempa S, Ridley AJ. 1998. Activation of both MAP kinase and phosphatidylinositide 3-kinase by Ras is required for hepatocyte growth factor/scatter factor-induced adherens junction disassembly. Mol Biol Cell 9:2185–2200.
- Price LS, Hajdo-Milasinovic A, Zhao J, Zwartkruis FJ, Collard JG, Bos JL. 2004. Rap1 regulates E-cadherin-mediated cell-cell adhesion. J Biol Chem 279:35127–35132.
- Pulimeno P, Paschoud S, Citi S. 2011. A role of ZO-1 and PLEKHA7 in recruiting paracingulin to epithelial junctions. J Biol Chem 286:16743-16750.
- Qin Y, Meisen WH, Hao Y, Macara IG. 2010. Tuba, a Cdc42 GEF, is required for polarized spindle orientation during epithelial cyst formation. J Cell Biol 189:661–669.
- Ren Y, Li R, Zheng Y, Busch H. 1998. Cloning and characterization of GEF-H1, a microtubule-associated guanine nucleotide exchange factor for Rac and Rho GTPases. J Biol Chem 273:34954–34960.
- Reynolds AB. 2007. p120-catenin: Past and present. Biochim Biophys Acta 1773:2–7.
- Reynolds AB, Daniel J, McCrea PD, Wheelock MJ, Wu J, Zhang Z. 1994. Identification of a new catenin: The tyrosine kinase substrate p120cas associates with E-cadherin complexes. Mol Cell Biol 14:8333–8342.
- Ridley AJ. 2001. Rho proteins: Linking signaling with membrane trafficking. Traffic 2:303–310.
- Ridley AJ, Comoglio PM, Hall A. 1995. Regulation of scatter factor/hepatocyte growth factor responses by Ras, Rac, and Rho in MDCK cells. Mol Cell Biol 15:1110–1122.
- Ridley AJ, Self AJ, Kasmi F, Paterson HF, Hall A, Marshall CJ, Ellis C. 1993. rho family GTPase activating proteins p190, bcr and rhoGAP show distinct specificities in vitro and in vivo. EMBO J 12:5151–5160.
- Rojas R, Ruiz WG, Leung SM, Jou TS, Apodaca G. 2001. Cdc42-dependent modulation of tight junctions and membrane protein traffic in polarized Madin-Darby canine kidney cells. Mol Biol Cell 12:2257–2274.
- Rossman KL, Der CJ, Sondek J. 2005. GEF means go: Turning on RHO GTPases with guanine nucleotide-exchange factors. Nat Rev Mol Cell Biol 6:167–180.
- Sahai E, Marshall CJ. 2002a. RHO-GTPases and cancer. Nat Rev Cancer 2:133–142.
- Sahai E, Marshall CJ. 2002b. ROCK and Dia have opposing effects on adherens junctions downstream of Rho. Nat Cell Biol 4:408–415.
- Sakurai A, Fukuhara S, Yamagishi A, Sako K, Kamioka Y, Masuda M, Nakaoka Y, Mochizuki N. 2006. MAGI-1 is required for Rap1 activation upon cell-cell contact and for enhancement of vascular endothelial cadherin-mediated cell adhesion. Mol Biol Cell 17:966–976.
- Samarin S, Nusrat A. 2009. Regulation of epithelial apical junctional complex by Rho family GTPases. Front Biosci 14:1129–1142.
- Samarin SN, Ivanov AI, Flatau G, Parkos CA, Nusrat A. 2007. Rho/Rho-associated kinase-II signaling mediates disassembly of epithelial apical junctions. Mol Biol Cell 18:3429–3439.
- Sander EE, Van Delft S, Ten Klooster JP, Reid T, Van Der Kammen RA, Michiels F, Collard JG. 1998. Matrix-dependent Tiam1/Rac signaling in epithelial cells promotes either cell-cell adhesion or cell migration and is regulated by phosphatidylinositol 3-kinase. J Cell Biol 143:1385–1398.
- Sato T, Fujita N, Yamada A, Ooshio T, Okamoto R, Irie K, Takai Y. 2006. Regulation of the assembly and adhesion activity of E-cadherin by nectin and afadin for the formation of adherens junctions in Madin-Darby canine kidney cells. J Biol Chem 281:5288–5299.
- Scherle P, Behrens T, Staudt LM. 1993. Ly-GDI, a GDP-dissociation inhibitor of the RhoA GTP-binding protein, is expressed preferentially in lymphocytes. Proc Natl Acad Sci USA 90:7568–7572.
- Schlegelmilch K, Mohseni M, Kirak O, Pruszak J, Rodriguez JR, Zhou D, Kreger BT, Vasioukhin V, Avruch J, Brummelkamp TR, Camargo FD. 2011. Yap1 acts downstream of alpha-catenin to control epidermal proliferation. Cell 144:782–795.
- Schmidt A, Hall A. 2002. Guanine nucleotide exchange factors for Rho GTPases: Turning on the switch. Genes Dev 16:1587–1609.
- Schneeberger EE, Lynch RD. 2004. The tight junction: A multifunctional complex. Am J Physiol Cell Physiol 286:C1213–1228.
- Severson EA, Lee WY, Capaldo CT, Nusrat A, Parkos CA. 2009. Junctional adhesion molecule A interacts with Afadin and PDZ-GEF2 to activate Rap1A, regulate beta1 integrin levels, and enhance cell migration. Mol Biol Cell 20:1916–1925.
- Shen L, Weber CR, Turner JR. 2008a. The tight junction protein complex undergoes rapid and continuous molecular remodeling at steady state. J Cell Biol 181:683–695.
- Shen Y, Hirsch DS, Sasiela CA, Wu WJ. 2008b. Cdc42 regulates E-cadherin ubiquitination and degradation through an epidermal growth factor receptor to Src-mediated pathway. J Biol Chem 283:5127–5137.
- Shewan AM, Maddugoda M, Kraemer A, Stehbens SJ, Verma S, Kovacs EM, Yap AS. 2005. Myosin 2 is a key Rho kinase target necessary for the local concentration of E-cadherin at cell-cell contacts. Mol Biol Cell 16:4531–4542.
- Shin K, Fogg VC, Margolis B. 2006. Tight junctions and cell polarity. Annu Rev Cell Dev Biol 22:207–235.
- Silvis MR, Kreger BT, Lien WH, Klezovitch O, Rudakova GM, Camargo FD, Lantz DM, Seykora JT, Vasioukhin V. 2011. {alpha}-catenin is a tumor suppressor that controls cell accumulation by regulating the localization and activity of the transcriptional coactivator Yap1. Sci Signal 4:ra33.
- Sousa S, Cabanes D, Archambaud C, Colland F, Lemichez E, Popoff M, Boisson-Dupuis S, Gouin E, Lecuit M, Legrain P, Cossart P. 2005. ARHGAP10 is necessary for alpha-catenin recruitment at adherens junctions and for Listeria invasion. Nat Cell Biol 7:954–960.
- Stepniak E, Radice GL, Vasioukhin V. 2009. Adhesive and signaling functions of cadherins and catenins in vertebrate development. Cold Spring Harb Perspect Biol 1:a002949.
- Su L, Hattori M, Moriyama M, Murata N, Harazaki M, Kaibuchi K, Minato N. 2003. AF-6 controls integrin-mediated cell adhesion by regulating Rap1 activation through the specific recruitment of Rap1GTP and SPA-1. J Biol Chem 278:15232–15238.
- Sugihara-Mizuno Y, Adachi M, Kobayashi Y, Hamazaki Y, Nishimura M, Imai T, Furuse M, Tsukita S. 2007. Molecular characterization of angiomotin/JEAP family proteins: Interaction with MUPP1/Patj and their endogenous properties. Genes Cells 12:473–486.
- Takai Y, Ikeda W, Ogita H, Rikitake Y. 2008. The immunoglobulin-like cell adhesion molecule nectin and its associated protein afadin. Annu Rev Cell Dev Biol 24:309–342.
- Takai Y, Nakanishi H. 2003. Nectin and afadin: Novel organizers of intercellular junctions. J Cell Sci 116:17–27.
- Takaishi K, Sasaki T, Kotani H, Nishioka H, Takai Y. 1997. Regulation of cell-cell adhesion by rac and rho small G proteins in MDCK cells. J Cell Biol 139:1047–1059.
- Tan EC, Leung T, Manser E, Lim L. 1993. The human active breakpoint cluster region-related gene encodes a brain protein with homology to guanine nucleotide exchange proteins and GTPase-activating proteins. J Biol Chem 268:27291–27298.
- Tanaka-Okamoto M, Hori K, Ishizaki H, Itoh Y, Onishi S, Yonemura S, Takai Y, Miyoshi J. 2011. Involvement of afadin in barrier function and homeostasis of mouse intestinal epithelia. J Cell Sci 124:2231–2240.
- Tcherkezian J, Lamarche-Vane N. 2007. Current knowledge of the large RhoGAP family of proteins. Biol Cell 99:67–86.
- Terry SJ, Zihni C, Elbediwy A, Vitiello E, Leefa Chong San IV, Balda MS, Matter K. 2011. Spatially restricted activation of RhoA signalling at epithelial junctions by p114RhoGEF drives junction formation and morphogenesis. Nat Cell Biol 13:159–166.
- Torres M, Stoykova A, Huber O, Chowdhury K, Bonaldo P, Mansouri A, Butz S, Kemler R, Gruss P. 1997. An alpha-E-catenin gene trap mutation defines its function in preimplantation development. Proc Natl Acad Sci USA 94:901–906.
- Troyanovsky B, Levchenko T, Mansson G, Matvijenko O, Holmgren L. 2001. Angiomotin: An angiostatin binding protein that regulates endothelial cell migration and tube formation. J Cell Biol 152:1247–1254.
- Tsukita S, Furuse M. 2002. Claudin-based barrier in simple and stratified cellular sheets. Curr Opin Cell Biol 14:531–536.
- Umeda K, Ikenouchi J, Katahira-Tayama S, Furuse K, Sasaki H, Nakayama M, Matsui T, Tsukita S, Furuse M, Tsukita S. 2006. ZO-1 and ZO-2 independently determine where claudins are polymerized in tight-junction strand formation. Cell 126:741–754.
- Utech M, Ivanov AI, Samarin SN, Bruewer M, Turner JR, Mrsny RJ, Parkos CA, Nusrat A. 2005. Mechanism of IFN-gamma-induced endocytosis of tight junction proteins: Myosin II-dependent vacuolarization of the apical plasma membrane. Mol Biol Cell 16:5040–5052.
- Van Aelst L, D'Souza-Schorey C. 1997. Rho GTPases and signaling networks. Genes Dev 11:2295–2322.
- Van Itallie CM, Anderson JM. 2006. Claudins and epithelial paracellular transport. Ann Rev Physiol 68:403–429.
- Van Itallie CM, Fanning AS, Bridges A, Anderson JM. 2009. ZO-1 stabilizes the tight junction solute barrier through coupling to the perijunctional cytoskeleton. Mol Biol Cell 20:3930–3940.
- Vasioukhin V, Bauer C, Yin M, Fuchs E. 2000. Directed actin polymerization is the driving force for epithelial cell-cell adhesion. Cell 100:209–219.
- Vaughan EM, Miller AL, Yu HY, Bement WM. 2011. Control of Local Rho GTPase Crosstalk by Abr. Curr Biol 21:270–277.
- Vigil D, Cherfils J, Rossman KL, Der CJ. 2010. Ras superfamily GEFs and GAPs: Validated and tractable targets for cancer therapy? Nat Rev Cancer 10:842–857.
- Walsh SV, Hopkins AM, Chen J, Narumiya S, Parkos CA, Nusrat A. 2001. Rho kinase regulates tight junction function and is necessary for tight junction assembly in polarized intestinal epithelia. Gastroenterology 121:566–579.
- Wang HR, Zhang Y, Ozdamar B, Ogunjimi AA, Alexandrova E, Thomsen GH, Wrana JL. 2003. Regulation of cell polarity and protrusion formation by targeting RhoA for degradation. Science 302:1775–1779.
- Watanabe T, Sato K, Kaibuchi K. 2009. Cadherin-mediated intercellular adhesion and signaling cascades involving small GTPases. Cold Spring Harb Perspect Biol 1:a003020.
- Wells CD, Fawcett JP, Traweger A, Yamanaka Y, Goudreault M, Elder K, Kulkarni S, Gish G, Virag C, Lim C, Colwill K, Starostine A, Metalnikov P, Pawson T. 2006. A Rich1/Amot complex regulates the Cdc42 GTPase and apical-polarity proteins in epithelial cells. Cell 125:535–548.
- Wennerberg K, Der CJ. 2004. Rho-family GTPases: It's not only Rac and Rho (and I like it). J Cell Sci 117:1301–1312.
- Wheelock MJ, Johnson KR. 2003. Cadherins as modulators of cellular phenotype. Annu Rev Cell Dev Biol 19:207–235.
- Wildenberg GA, Dohn MR, Carnahan RH, Davis MA, Lobdell NA, Settleman J, Reynolds AB. 2006. p120-catenin and p190RhoGAP regulate cell-cell adhesion by coordinating antagonism between Rac and Rho. Cell 127:1027–1039.
- Wittchen ES, Haskins J, Stevenson BR. 1999. Protein interactions at the tight junction. Actin has multiple binding partners, and zo-1 forms independent complexes with zo-2 and zo-3. J Biol Chem 274:35179–35185.
- Wittchen ES, Haskins J, Stevenson BR. 2003. NZO-3 expression causes global changes to actin cytoskeleton in Madin-Darby canine kidney cells: Linking a tight junction protein to Rho GTPases. Mol Biol Cell 14:1757–1768.
- Wittmann T, Bokoch GM, Waterman-Storer CM. 2004. Regulation of microtubule destabilizing activity of Op18/stathmin downstream of Rac1. J Biol Chem 279:6196–6203.
- Woodcock SA, Rooney C, Liontos M, Connolly Y, Zoumpourlis V, Whetton AD, Gorgoulis VG, Malliri A. 2009. SRC-induced disassembly of adherens junctions requires localized phosphorylation and degradation of the rac activator tiam1. Mol Cell 33:639–653.
- Xu J, Anuar F, Ali SM, Ng MY, Phua DC, Hunziker W. 2009. Zona occludens-2 is critical for blood-testis barrier integrity and male fertility. Mol Biol Cell 20:4268–4277.
- Xu J, Kausalya PJ, Phua DC, Ali SM, Hossain Z, Hunziker W. 2008. Early embryonic lethality of mice lacking ZO-2, but Not ZO-3, reveals critical and nonredundant roles for individual zonula occludens proteins in mammalian development. Mol Cell Biol 28:1669–1678.
- Yamada S, Nelson WJ. 2007. Localized zones of Rho and Rac activities drive initiation and expansion of epithelial cell-cell adhesion. J Cell Biol 178:517–527.
- Yamanaka T, Horikoshi Y, Suzuki A, Sugiyama Y, Kitamura K, Maniwa R, Nagai Y, Yamashita A, Hirose T, Ishikawa H, Ohno S. 2001. PAR-6 regulates aPKC activity in a novel way and mediates cell-cell contact-induced formation of the epithelial junctional complex. Genes Cells 6:721–731.
- Yanagisawa M, Anastasiadis PZ. 2006. p120 catenin is essential for mesenchymal cadherin-mediated regulation of cell motility and invasiveness. J Cell Biol 174:1087–1096.
- Yanagisawa M, Huveldt D, Kreinest P, Lohse CM, Cheville JC, Parker AS, Copland JA, Anastasiadis PZ. 2008. A p120 catenin isoform switch affects Rho activity, induces tumor cell invasion, and predicts metastatic disease. J Biol Chem 283:18344–18354.
- Zalcman G, Closson V, Camonis J, Honore N, Rousseau-Merck MF, Tavitian A, Olofsson B. 1996. RhoGDI-3 is a new GDP dissociation inhibitor (GDI). Identification of a non-cytosolic GDI protein interacting with the small GTP-binding proteins RhoB and RhoG. J Biol Chem 271:30366–30374.
- Zhadanov AB, Provance DW, Speer CA, Coffin JD, Goss D, Blixt JA, Reichert CM, Mercer JA. 1999. Absence of the tight junctional protein AF-6 disrupts epithelial cell-cell junctions and cell polarity during mouse development. Curr Biol 9:880–888.
- Zhang H, Macara IG. 2008. The PAR-6 polarity protein regulates dendritic spine morphogenesis through p190 RhoGAP and the Rho GTPase. Dev Cell 14:216–226.