Abstract
Non-bilayer phospholipid arrangements are three-dimensional structures that can form when anionic phospholipids with an intermediate form of the tubular hexagonal phase II (HII), such as phosphatidic acid, phosphatidylserine or cardiolipin, are present in a bilayer of lipids. The drugs chlorpromazine and procainamide, which trigger a lupus-like disease in humans, can induce the formation of non-bilayer phospholipid arrangements, and we have previously shown that liposomes with non-bilayer arrangements induced by these drugs cause an autoimmune disease resembling human lupus in mice. Here we show that liposomes with non-bilayer phospholipid arrangements induced by Mn2+ cause a similar disease in mice. We extensively characterize the physical properties and immunological reactivity of liposomes made of the zwitterionic lipid phosphatidylcholine and a HII-preferring lipid, in the absence or presence of Mn2+, chlorpromazine or procainamide. We use an hapten inhibition assay to define the epitope recognized by sera of mice with the disease, and by a monoclonal antibody that binds specifically to non-bilayer phospholipid arrangements, and we report that phosphorylcholine and glycerolphosphorylcholine, which form part of the polar region of phosphatidylcholine, are the only haptens that block the binding of the tested antibodies to non-bilayer arrangements. We propose a model in which the negatively charged HII-preferring lipids form an inverted micelle by electrostatic interactions with the positive charge of Mn2+, chlorpromazine or procainamide; the inverted micelle is inserted into the bilayer of phosphatidylcholine, whose polar regions are exposed and become targets for antibody production. This model may be relevant in the pathogenesis of human lupus.
Introduction
Phospholipids are the main structural constituents of all cell membranes; their amphiphatic nature leads to the formation of a smooth bilayer that delimits cells and vesicles and acts as a matrix in which proteins are inserted or anchored. The zwitterionic lipid phosphatidylcholine, which is composed of a choline head group, glycerophosphoric acid, a saturated fatty acid (such as palmitic acid) and an unsaturated fatty acid (such as oleic acid), is the most abundant phospholipid in cell membranes (Voet and Voet Citation2011). However, some anionic phospholipids that have a tubular hexagonal phase II (HII), such as phosphatidic acid, phosphatidylserine or cardiolipin, can lead to the formation of non-bilayer phospholipid arrangements within the lipid bilayer.
Non-bilayer phospholipid arrangements appear as protuberances on the surface of experimental membrane models, such as liposomes; they are transiently formed on the membranes of cells that are participating in membrane fusion process (Hafez and Cullis Citation2001, Wong-Baeza et al. Citation2010), and they have a role in the organization of tight junctions (Wegener and Galla Citation1996) and in the activation of membrane enzymes (van den Brink-van et al. Citation2001). Non-bilayer phospholipid arrangements can be induced and stabilized by some antibiotics, non-polar peptides, Ca2+ (de Kruijff et al. Citation1979, Verkleij et al. Citation1982, Cullis et al. Citation1991) and the drugs chlorpromazine and procainamide (Baeza et al. Citation2004). The structure of these arrangements has been studied using 31P and 2H nuclear magnetic resonance (NMR) spectroscopy, X-ray diffraction, differential scanning calorimetry (de Kruijff et al. Citation1979, Cullis et al. Citation1991, Verkleij Citation1991, Baeza et al. Citation2004, Spink Citation2008) and flow cytometry (Baeza et al. Citation1995, Citation2004, Aguilar et al. Citation1999).
Non-bilayer phospholipid arrangements induced by chlorpromazine or procainamide are immunogenic in mice; polyclonal and monoclonal antibodies that bind non-bilayer phospholipid arrangements have been obtained, and these antibodies do not bind to smooth liposomes or to phospholipids immobilized on a plastic surface (Aguilar et al. Citation1999, Baeza et al. Citation2004). In a previous paper (Baeza et al. Citation2004), we described that liposomes with non-bilayer phospholipid arrangements, induced by chlorpromazine or procainamide, cause the development of an autoimmune disease resembling human lupus in syngeneic BALB/c and non-syngeneic NIH mice. A similar disease was produced by treating mice directly with chlorpromazine or procainamide (which can induce non-bilayer phospholipid arrangements on mice cells) or by injecting the monoclonal antibody H308-AP (which binds specifically to non-bilayer phospholipid arrangements and can stabilize them on murine cells). These results led us to propose that non-bilayer phospholipid arrangements, either on the surface of liposomes or on the surface of cells (induced by chlorpromazine or procainamide, or stabilized by the H308-AP monoclonal antibody) can induce the development of an autoimmune disease. However, it remained possible that other effects of chlorpromazine or procainamide, not related to the induction of non-bilayer phospholipid arrangements, were responsible for the disease induction.
Here, we evaluated if liposomes with non-bilayer phospholipid arrangements induced by Mn2+ caused a similar disease in mice. We extensively characterized the physical properties and immunological reactivity of liposomes made of the zwitterionic lipid phosphatidylcholine and a HII-preferring lipid (phosphatidic acid, phosphatidylserine or cardiolipin), in the absence or presence of Mn2+, chlorpromazine or procainamide; and we report the histological abnormalities and auto-antibodies produced by mice that received non-bilayer phospholipid arrangements induced by Mn2+. Finally, we used a hapten inhibition assay to define the epitope recognized by the monoclonal antibody H308-AP and by the sera of mice with the autoimmune disease, and we propose a model that explains the induction of non-bilayer phospholipid arrangements by Mn2+, chlorpromazine and procainamide.
Methods
Lipids, drugs and antibodies
Egg-yolk L-α-phosphatidic acid, bovine brain L-α-phosphatidylserine, bovine heart cardiolipin, egg-yolk L-α-phosphatidylcholine, dipalmitoyl-L-α-phosphatidylcholine, dipalmitoyl-L-α-phosphatidic acid, phosphate, phosphorylcholine, glycerolphosphorylcholine, phosphorylserine, glycerolphosphorylserine, glycerolphosphate, glycerolphosphorylglycerol, chlorpromazine, procainamide and chloroquine were purchased from Sigma Aldrich (St Louis, MO, USA). All phospholipids were at least 97% pure. The murine IgM H308-AP monoclonal antibody, which binds specifically to non-bilayer phospholipid arrangements, was prepared and affinity purified as described previously (Aguilar et al. Citation1999), and conjugated to fluorescein isothiocyanate (FITC) using the EZ-label FITC protein labelling kit from Pierce Biotechnology (Rockford, IL USA). An IgM monoclonal antibody that binds a protein from Trichinella spiralis (Ts-mAb) was used as isotype control (Baeza et al. Citation2004).
Preparation of liposomes and liposomes with non-bilayer phospholipid arrangements
For most of the experiments, liposomes contained the zwitterionic lipid egg-yolk L-α-phosphatidylcholine and a HII-preferring lipid (egg-yolk L-α-phosphatidic acid, bovine brain L-α-phosphatidylserine or bovine heart cardiolipin) at the following molar ratios: phosphatidylcholine/phosphatidic acid 2:1, phosphatidylcholine/phosphatidylserine 4:1 and phosphatidylcholine/cardiolipin 2:1. In some cases, we also prepared liposomes containing dipalmitoylphosphatidylcholine/phosphatidylcholine/dipalmitoylphosphatidic acid 1.2:0.8:1. Each molar ratio is the optimal for the induction of non-bilayer phospholipid arrangements in the corresponding liposome, as determined experimentally (data not shown). To prepare the liposomes, 9 μmol of phospholipid mixture were dissolved in 1 ml diethyl ether and 330 μl of TS buffer (10 mM Tris-HCl, 1 mM NaCl, pH 7), mixed in a Vortex and sonicated three times (5 sec sonication followed by 30 sec resting period), in a Lab Supply G112SPI sonicator (Laboratory supplies, Hicksville, NY, USA). The diethyl ether was then removed under a stream of oxygen-free dry nitrogen at reduced pressure, using a rotary evaporator at 37°C (Szoka and Papahadjopoulos Citation1978). The liposomes were then filtered through MF-Millipore (Billerica, MA, USA) membranes with 0.45-μm pores to homogenize their size (Ibáñez et al. Citation1996). To induce the formation of non-bilayer phospholipid arrangements, liposomes were incubated 30 min at 37°C in the presence of 5 mM MnCl2, 3 mM chlorpromazine or 8 mM procainamide (Baeza et al. Citation2004).
Characterization of liposomes
Freeze-fracture electron microscopy
Liposomes and liposomes with non-bilayer phospholipid arrangements (0.1 μmol in 100 μl TS buffer) were cooled for 1 h at 5°C; 15% glycerol was added to prevent freeze damage, and liposomes were frozen in Freon 22 previously cooled with liquid nitrogen. The frozen liposomes were fractured in a double replica device and replicated in a Balzers BAF 400D instrument (Balzers AG, Balzers, Liechtenstein) at −120°C, with a vacuum of 3 × 10-7 mbar and an incidence angle of 45° for the platinum electrode. The replicas were cleaned with concentrated chromic mixture and analyzed in a JEOL 2000 EX electron microscope (GFE, Aachen, North Rhine Westphalia, Germany) to evaluate the presence of non-bilayer phospholipid arrangements (Baeza et al. Citation1994).
31P nuclear magnetic resonance (NMR) spectroscopy
Liposomes and liposomes with non-bilayer phospholipid arrangements (0.1 μmol in100 μl TS buffer) were placed in 5 mm (OD) NMR tubes. 31P NMR spectra were obtained with a JEOL FX90Q multinuclear Fourier transform spectrometer (Blue Lion Biotech, Snoqualmie, WA, USA) operating at 36.23 MHz and 37°C. A spectral width of 7.5 kHz was used with 30,000 transients being accumulated with a 7-μsec pulse and 2.8-sec pulse delay. Broadband proton-noise decoupling was used in all measurements, and 2H2O was included in the liposomes samples as a spectrometer lock (Smaal et al. Citation1987, Baeza et al. Citation1994, Corona-Ortega et al. Citation2009).
Flow cytometry
Liposomes and liposomes with non-bilayer phospholipid arrangements were resuspended in TS buffer and analyzed in a FACSCalibur flow cytometer (Becton Dickinson, San Jose, CA, USA) with CellQuest software. Diffraction of the laser beam (forward scatter, FSC) is proportional to liposome size and/or liposome aggregation, while refraction and reflection of the laser beam (side scatter, SSC) are proportional to the complexity of the liposomal surfaces, which increases in the presence of non-bilayer phospholipid arrangements. We have previously shown that the SSC signal of liposomes with non-bilayer phospholipid arrangements is significantly higher (D ≥0.5, p < 0.001) than the SSC signal of smooth liposomes (Aguilar et al. Citation1999, Baeza et al. Citation2004, Campos et al. Citation2006).
Flow cytometry was also used to assess the binding of the H308-AP monoclonal antibody to liposomes. Liposomes and liposomes with non-bilayer phospholipid arrangements (0.1 μmol in 100 μl TS buffer) were incubated at 37°C for 1 h with FITC-labelled H308-AP monoclonal antibody or with isotype control. After the incubation, liposomes were centrifuged at 200,000 g for 50 min at 18°C and washed with TS buffer. Ten thousand events were acquired for each sample.
Mouse model of autoimmune disease resembling human lupus
In a previous paper (Baeza et al. Citation2004), we described that liposomes with non-bilayer phospholipid arrangements, induced by chlorpromazine or procainamide, cause the development of an autoimmune disease resembling human lupus in BALB/c mice. Here, we used liposomes with non-bilayer phospholipid arrangements induced by Mn2+ to cause the disease. Ten specific pathogen-free female 2-month-old BALB/c mice were injected intrasplenically, on days 1 and 15, with phosphatidylcholine/phosphatidic acid (2:1) liposomes (50 nmol of phosphatidic acid in 50 μl TS buffer) that had been incubated with 5 mM MnCl2. Mice received the same amount of liposomes by intraperitoneal injection on day 30, and then every week for six months. As a positive control, two additional groups of female mice were treated in a similar way, but using phosphatidylcholine/phosphatidic acid (2:1) liposomes incubated with 3 mM chlorpromazine or phosphatidylcholine/phosphatidic acid (2:1) liposomes incubated with 8 mM procainamide. The negative control groups consisted of mice treated with phosphatidylcholine/phosphatidic acid (2:1) liposomes alone and mice treated with TS buffer.
Blood was taken from mice previous to liposome injection and each month after the first intraperitoneal injection, for a total of three months. Sera were heated at 56°C for 30 min to inactivate complement. Animals, which received the liposomes by intraperitoneal injection every week, were euthanized after six months and complete autopsies were performed; tissue fragments from different organs were collected, fixed in 10% formaldehyde, embedded in paraffin, sectioned at 6-μm thickness and stained with haematoxylin and eosin for histological evaluation (Baeza et al. Citation2004). All our protocols for animal care and use were reviewed and approved by the Bioethics Committee of our Institution following International rules.
Characterization of the antibodies produced by mice with autoimmune disease
Detection of anti-cardiolipin, anti-histone and lupus anticoagulant antibodies
Anti-cardiolipin, anti-histone and lupus anticoagulant antibodies were determined as previously described (Baeza et al. Citation2004). For anti-cardiolipin and anti-histone antibodies, results are reported as arbitrary units, calculated as: (AsP – AsW)/(AsH – AsW), where AsP is the absorbance of sera from immunized mice, AsH is the absorbance of sera from pre-immune mice and AsW is the absorbance of controls without sera.
Detection of antibodies that bind non-bilayer phospholipid arrangements in sera
Flow cytometry was used to detect antibodies that bind non-bilayer phospholipid arrangements in the sera of mice with autoimmune disease. Liposomes and liposomes with non-bilayer phospholipid arrangements (0.1 μmol in100 μl TS buffer) were incubated at 37°C for 1 h with inactivated sera (1:50 dilution in TS buffer) from mice with the autoimmune disease or from pre-immune mice. After the incubation, liposomes were centrifuged at 200,000 g for 50 min at 18°C and washed with TS buffer. A FITC-labelled goat anti-mouse antibody was added, and after 1 h at 37°C, liposomes were washed again before flow cytometry analysis. Ten thousand events were acquired for each sample. The presence of antibodies that bind non-bilayer phospholipid arrangements in sera was also assessed with the liposomal enzyme-linked immunosorbent assay (ELISA) (Aguilar et al. Citation1999), which is described below.
Hapten inhibition assay
Phosphate, phosphorylcholine, glycerolphosphorylcholine, phosphorylserine, glycerolphosphorylserine, glycerolphosphate and glycerolphosphorylglycerol were used as 0.2, 0.4, 0.6, 0.8 and 1.0 mM solutions. Aliquots of H308-AP monoclonal antibody, or inactivated sera from mice with the autoimmune disease (1:50 dilution in TS buffer), were incubated with each one of the hapten solutions (1:1, v/v) for 30 min at 37°C. The ability of the H308-AP monoclonal antibody and sera to bind liposomes with non-bilayer phospholipid arrangements was assessed using liposomal ELISA (Aguilar et al. Citation1999).
Briefly, microtiter plates with 96 flat-bottom wells (Costar Co, Cambridge, MA, USA) were coated with liposomes or with liposomes with non-bilayer phospholipid arrangements (0.1 μmol in 100 μl TS buffer) at room temperature for 12 h. After this incubation, plates were blocked with 200 μl per well of 8% foetal bovine serum in TS buffer for 1 h at room temperature and washed. 100 μl of H308-AP monoclonal antibody or inactivated sera, with or without previous incubation with hapten solution, were added. After incubation for 1 h at 37°C, the plates were washed 5 times and 100 μl of goat anti-mouse IgM or goat anti-mouse polyvalent antibody conjugated to peroxidase (1:2000 dilution in TS buffer) were added to each well. The plates were incubated for 1 h at 37°C and washed 5 times before adding 100 μl of peroxidase substrate (10 mg o-phenylendiamine and 20 μl of 30% H2O2 in 25 ml TS buffer). After 20 min at 37°C, the enzymatic reaction was stopped by addition of 50 μl of sulphuric acid 2.5 M and absorbances were read at 492 nm in a Labsystems Multiskan MS reader (MTX Labsystems, Vienna, Virginia, USA). Each sample was assayed in duplicate.
Results
Characterization of liposomes with non-bilayer phospholipid arrangements induced by Mn2+, chlorpromazine or procainamide
Liposomes containing phosphatidylcholine and a HII-preferring lipid (phosphatidic acid, phosphatidylserine or cardiolipin) are unilamellar in TS buffer; they are 100–400 nm wide and their lipid bilayer has a smooth surface, similar to that described for bilayers in fluid state (Singh et al. Citation1992, Baeza et al. Citation1994). The top panel of shows a representative liposome containing phosphatidylcholine and cardiolipin; liposomes containing phosphatidylcholine and phosphatidylserine or phosphatidylcholine and phosphatidic acid are similar (data not shown). The addition of Mn2+ ions induced non-bilayer phospholipid arrangements; these arrangements have an average thickness of 3.7 nm and are organized in pentagonal and hexagonal strings that do not modify liposome size. The middle panel of shows a phosphatidylcholine/cardiolipin liposome treated with 5 mM Mn2+. The addition of chlorpromazine induced non-bilayer phospholipid arrangements with an average thickness of 5.5 nm; these arrangements are isolated or form small strings and show fusogenic properties: they cause the fusion of small liposomes into larger liposomes of up to 1 μm radius. A small portion of a fused liposome containing phosphatidylcholine and cardiolipin, and treated with 3 mM chlorpromazine, is shown in the bottom panel of . Procainamide also induced non-bilayer phospholipid arrangements in these liposomes; the arrangements have an average thickness of 4.7 nm and also have fusogenic properties (data not shown).
Figure 1. Detection of non-bilayer phospholipid arrangements on liposomes by freeze-fracture electron microscopy and 31P NMR spectroscopy. Liposomes made of phosphatidylcholine/cardiolipin (2:1 molar ratio) were incubated at 37°C for 30 min with: TS buffer (top panels) or the non-bilayer phospholipid arrangements inducers: 5 mM MnCl2 (middle panels), or 3 mM chlorpromazine (bottom panels). (A) Freeze-fracture electron microscopy. The black arrow in the top panel indicates the shadow direction. Small white arrows show non-bilayer phospholipid arrangements. The solid line represents 100 nm in all micrographs. (B) 31P NMR spectra were attained at 37°C using 70 μmol of liposomes incubated with TS buffer or the same non-bilayer phospholipid arrangements inducers as above. The graph number 1 in the middle panel corresponds to phosphatidylcholine/cardiolipin (2:1) liposomes and the graph number 2 corresponds to phosphatidylcholine liposomes as a control. The broken line indicates the reference values for changes in ppm of NMR. The same liposome preparations were used for these determinations and for the immunological assays.
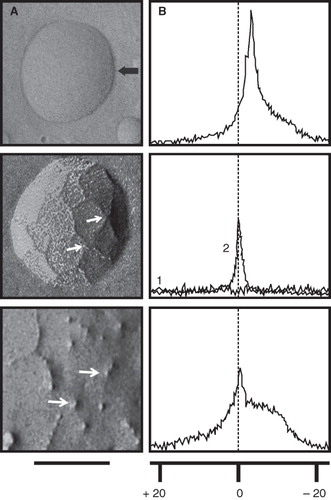
Phospholipids have 31P in their head groups, so 31P NMR spectroscopy can be used to assess the physical properties of liposomes (Smaal et al. Citation1987, Baeza et al. Citation1994). The top panel of shows the 31P NMR spectrum of a liposome containing phosphatidylcholine and cardiolipin; liposomes containing phosphatidylcholine and phosphatidylserine or phosphatidylcholine and phosphatidic acid have similar spectra (data not shown). The addition of 5 mM Mn2+ to phosphatidylcholine/cardiolipin liposomes flattens the 31P NMR (, middle panel, graph 1), because the non-bilayer phospholipid arrangements induced by Mn2+ transport this cation to the inside of the liposome, and its paramagnetic effects (Aguilar et al. Citation1999, Madine et al. Citation2008) on phospholipids on both the outer and the inner side of the bilayer cancel each other. The addition of 5 mM Mn2+ to liposomes made of phosphatidylcholine alone causes only a small decrease in the 31P NMR spectrum (, middle panel, graph 2), which suggests that HII-preferring lipids (such as cardiolipin) are needed to form non-bilayer phospholipid arrangements.
The addition of chlorpromazine to phosphatidylcholine/cardiolipin liposomes produced a 31P NMR spectrum with a sharp peak, which is slightly shifted towards a lower magnetic field showing non-bilayer phospholipid arrangements, and with a broad tail towards a higher magnetic field (, bottom panel), which reflects the presence of larger liposomes. The addition of procainamide to phosphatidylcholine/cardiolipin liposomes produced a 31P NMR spectrum similar to obtained with chlorpromazine (data not shown), and the addition of these drugs did not modify the 31P NMR spectrum of liposomes made of phosphatidylcholine alone (data not shown). The presence of non-bilayer phospholipid arrangements on liposomes can also be detected as an increase in SSC signal by flow cytometry. The addition of Mn2+or chlorpromazine to phosphatidylcholine/phosphatidic acid liposomes or to phosphatidylcholine/phosphatidylserine liposomes, respectively, caused an increase in SSC signal (; values of D ≥0.5 with p < 0.001 were considered significant). The amount of non-bilayer phospholipid arrangements increased with the concentration of Mn2+ or chlorpromazine; 5 mM Mn2+ and 3 mM chlorpromazine, respectively, induce the highest amounts of non-bilayer phospholipid arrangements on liposomes. A similar study was made using procainamide; we found that 8 mM procainamide induces the highest amount of non-bilayer phospholipid arrangements on liposomes. So, chlorpromazine is more efficient than Mn2+ for the induction of non-bilayer phospholipid arrangements, and Mn2+ is more efficient than procainamide.
Figure 2. Detection of non-bilayer phospholipid arrangements on liposomes by flow cytometry. Liposomes made of phosphatidylcholine (PC)/phosphatidic acid (PA) (2:1 molar ratio) or phosphatidylcholine/phosphatidylserine (PS) (4:1) were incubated at 37°C for 30 min with the indicated concentrations of Mn2+ or chlorpromazine. Changes in bilayer complexity (SSC) and liposomal aggregation (FSC) were evaluated. Bilayer complexity (SSC) is represented in histograms (red lines represent liposomes alone; blue lines represent liposomes with Mn2+ or with chlorpromazine; the D value of the Kolmogorov-Smirnov test is indicated); bilayer complexity and liposomal aggregation are represented in density plots.
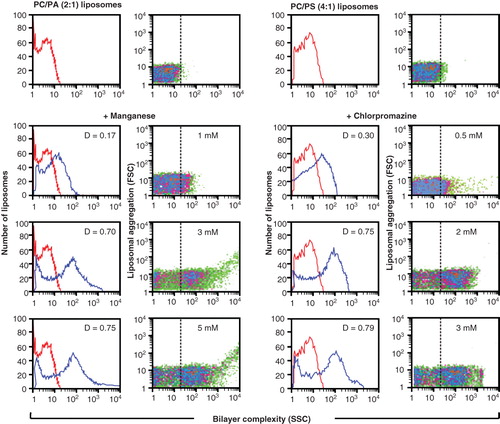
As controls, liposomes containing only phosphatidylcholine, or rigid liposomes made from dipalmitoylphosphatidylcholine/phosphatidylcholine/dipalmitoylphosphatidic acid 1.2:0.8:1, were examined in the presence of Mn2+, chlorpromazine or procainamide. Freeze-fracture electron microscopy revealed smooth surfaces identical to those of liposomes alone (data not shown) in every case, with the exception of chlorpromazine, which induced the formation of non-bilayer phospholipid arrangements in rigid liposomes. These results were confirmed by flow cytometry ().
Figure 3. H308-AP monoclonal antibody binds non-bilayer phospholipid arrangements induced by Mn2+, chlorpromazine and procainamide. Liposomes made of phosphatidylcholine (PC)/phosphatidic acid (PA) (2:1 molar ratio), phosphatidylcholine/cardiolipin (CL) (2:1) or dipalmitoylphosphatidylcholine (DPPC)/phosphatidylcholine/dipalmitoylphosphatidic acid (DPPA) (1.2:0.8:1) were used alone or in the presence of 5 mM MnCl2, 3 mM chlorpromazine (CPZ), 8 mM procainamide (PCM), 5 mM MgCl2, 5 mM BaCl2, 1.5 mM NaCl2 or 5 mM chloroquine (CQ). Liposomes were stained with FITC-labelled H308-AP monoclonal antibody or with an isotype control. Changes in liposomal fluorescence, bilayer complexity (SSC), and liposomal aggregation (FSC) were evaluated. One experiment representative of three is shown.
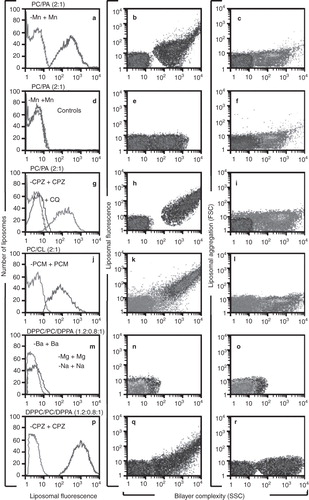
H308-AP monoclonal antibody binds non-bilayer phospholipid arrangements induced by Mn2+, chlorpromazine and procainamide
The H308-AP monoclonal antibody does not bind smooth liposomes made of phosphatidylcholine and phosphatidic acid (, green histogram), but in the presence of Mn2+, H308-AP clearly binds these liposomes (, blue histogram; with a Kolmogorov-Smirnov value of D = 0.85, at p < 0.001 (Young Citation1987)). The isotype control does not bind these same liposomes (). The SSC signal indicates that the pattern of non-bilayer phospholipid arrangements in Mn2+-treated liposomes is altered in the presence of the H308-AP monoclonal antibody (). Liposomal aggregation (FSC), which can produce nonspecific increases in fluorescence, was not evident, since the FSC signal in the presence of H308-AP is similar to the FSC signal in the presence of the isotype control (). The H308-AP monoclonal antibody also binds to phosphatidylcholine/phosphatidic acid liposomes in the presence of chlorpromazine () and to phosphatidylcholine/cardiolipin liposomes in the presence of procainamide (). Furthermore, we demonstrated that chloroquine inhibits the formation of non-bilayer phospholipid arrangements, since the presence of chloroquine prevented the binding of H308-AP to chlorpromazine-treated liposomes (, blue histogram).
To discard the possibility that H308-AP monoclonal antibody recognizes a lipid-cation complex and/or the reduction in the liposomal charge in the presence Mn2+ chlorpromazine or procainamide (as these drugs are monovalent cations), we used rigid liposomes made from dipalmitoylphosphatidylcholine/phosphatidylcholine/dipalmitoylphosphatidic acid. H308-AP did not bind to these rigid liposomes in the presence of divalent (Ba2+ or Mg2+) or monovalent (Na1+) cations (). However, H308-AP bound to these rigid liposomes in the presence of chlorpromazine ().
Phosphorylcholine and glycerolphosphorylcholine prevent the binding of H308-AP to non-bilayer phospholipid arrangements
Haptens that form part of the polar region of the HII-preferring lipids phosphatidic acid (phosphate and glycerolphosphate), phosphatidylserine (phosphorylserine and glycerolphosphorylserine) and cardiolipin (phosphate, glycerolphosphate and glycerolphosphorylglycerol) did not prevent the binding of H308-AP monoclonal antibody to non-bilayer phospholipid arrangements (). Phosphorylcholine and glycerolphosphorylcholine, which form part of the polar region of the zwitterionic lipid phosphatidylcholine, are the only haptens that block the binding of H308-AP to non-bilayer phospholipid arrangements (). Similar results were obtained with non-bilayer phospholipid arrangements induced by Mn2+, chlorpromazine and procainamide.
Figure 4. Phosphorylcholine and glycerolphosphorylcholine prevent the binding of H308-AP to non-bilayer phospholipid arrangements. H308-APmAb was incubated with phosphate, phosphorylcholine, glycerolphosphorylcholine, phosphorylserine, glycerolphosphorylserine, glycerolphosphate or glycerolphosphorylglycerol (the chemical structure of some of these haptens is shown). The ability of the H308-AP monoclonal antibody to bind phosphatidylcholine/cardiolipin liposomes with chlorpromazine-induced non-bilayer phospholipid arrangements was assessed by liposomal ELISA. As a negative control, we used liposomes without non-bilayer phospholipid arrangements.
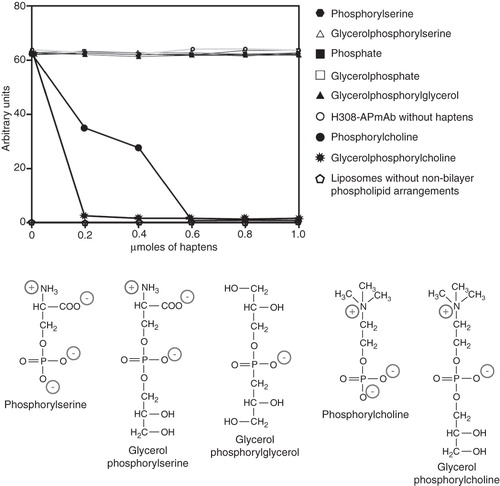
Liposomes with non-bilayer phospholipid arrangements induced by Mn2+ cause an autoimmune disease resembling human lupus in mice
In a previous paper (Baeza et al. Citation2004), we described that liposomes with non-bilayer phospholipid arrangements, induced by chlorpromazine or procainamide, cause the development of an autoimmune disease resembling human lupus in BALB/c mice. Here, we evaluated if liposomes with non-bilayer phospholipid arrangements induced by Mn2+ caused a similar disease. Three months after the initial administration of phosphatidylcholine/phosphatidic acid liposomes that had been incubated with 5 mM MnCl2, 80% of mice developed alopecia, particularly in the face, exhibiting symmetric malar masks (). Six months after the initial administration of liposomes with non-bilayer phospholipid arrangements induced by Mn2+, 80% of these mice had histopathological abnormalities in the skin and 100% had histopathological abnormalities in the kidneys. The kidneys presented a mild expansion of the mesangial matrix with thickened capillary walls in the glomeruli (), while the alopecic skin showed extensive epidermal atrophy, mild chronic inflammatory infiltrate and an accentuated decrease in terminal hair follicles. Many of these follicles had a widened external fibrous sheath with extensive disaggregation of matrical cells, surrounded by a chronic inflammatory infiltrate and haemorrhage ().
Figure 5. Liposomes with non-bilayer phospholipid arrangements induced by Mn2+ cause an autoimmune disease resembling human lupus in mice. (A) and (B) Representative photographs of 6-month-old female BALB/c mice treated with phosphatidylcholine/phosphatidic acid liposomes with non-bilayer phospholipid arrangements induced by Mn2+, showing facial lesions (mice are identified by marks made with a picric acid solution, which show as yellow colouring). (C) Glomeruli with mesangial hypercellularity (asterisks) and thickening of capillary walls (arrows). (D) Skin from alopecic areas showing epidermal atrophy (arrows), and widening of hair bulb fibrous sheath (asterisks) with total disorganization of matrical cells. In contrast, there are not histological abnormalities in kidney (E) or skin (F) from control mice treated with phosphatidylcholine/phosphatidic acid liposomes (H/E, 40× in all panels).
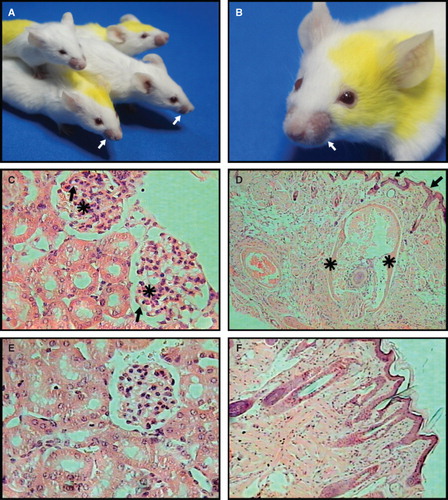
As a positive control, we treated mice with phosphatidylcholine/phosphatidic acid liposomes that had been incubated with 3 mM chlorpromazine or with phosphatidylcholine/phosphatidic acid liposomes that had been incubated with 8 mM procainamide (Baeza et al. Citation2004). Two months after the initial administration of liposomes with non-bilayer phospholipid arrangements induced by chlorpromazine, 80% of these animals developed malar masks; four months later, 80% had histopathological abnormalities in the skin and 100% exhibited glomerular lesions in the kidneys. Three months after the initial administration of liposomes with non-bilayer phospholipid arrangements induced by procainamide, 80% of mice developed malar masks; four months later, 80% had histopathological abnormalities in the skin and 100% showed morphological abnormalities in the kidneys. The histopathological abnormalities in the skin and kidneys developed in these three groups of mice were similar.
The negative control groups consisted of mice treated with phosphatidylcholine/phosphatidic acid liposomes alone and mice treated with TS buffer; none of the mice in the negative control groups showed piloerection or alopecia, or any histopathological abnormalities in the kidneys () or skin ().
Sera of mice with the autoimmune disease bind specifically to non-bilayer phospholipid arrangements, and have anti-cardiolipin, anti-histone and lupus anticoagulant antibodies
The serum of a mouse with the autoimmune disease caused by Mn2+-treated phosphatidylcholine/phosphatidic acid liposomes binds Mn2+-treated phosphatidylcholine/phosphatidic acid liposomes (, D = 0.96, p < 0.001 vs. pre-immune serum). In addition, this serum also binds chlorpromazine-treated phosphatidylcholine/phosphatidic acid liposomes (). Similarly, the serum of a mouse with the autoimmune disease caused by chlorpromazine-treated phosphatidylcholine/phosphatidic acid liposomes binds chlorpromazine-treated phosphatidylcholine/phosphatidic acid liposomes (), and Mn2+-treated phosphatidylcholine/phosphatidic acid liposomes (). The reactivity of these two sera is representative of all the sera in each group. The sera of pre-immune mice or from the negative control groups did not bind non-bilayer phospholipid arrangements (data not shown). The antibodies against non-bilayer phospholipid arrangements were detected as early as the first month after the administration of liposomes with non-bilayer phospholipid arrangements, and their titers remained constant for at least six months.
Figure 6. Sera of mice with the autoimmune disease bind specifically to non-bilayer phospholipid arrangements. The serum of a mouse with the autoimmune disease caused by Mn2+-treated phosphatidylcholine/phosphatidic acid liposomes was assayed by flow cytometry, using Mn2+-treated phosphatidylcholine/phosphatidic acid liposomes (A) or chlorpromazine-treated phosphatidylcholine/phosphatidic acid liposomes (B). The serum of a mouse with the autoimmune disease caused by chlorpromazine-treated phosphatidylcholine/phosphatidic acid liposomes was assayed by flow cytometry, using chlorpromazine-treated phosphatidylcholine/phosphatidic acid liposomes (C), and Mn2+-treated phosphatidylcholine/phosphatidic acid liposomes (D). Changes in liposomal fluorescence were evaluated. Filled histograms represent the serum of a mouse with the disease; empty histograms represent pre-immune serum.
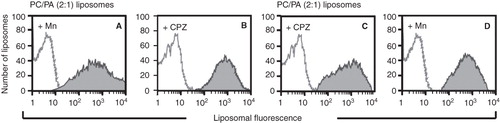
shows the titers of antibodies that bind non-bilayer phospholipid arrangements in mice which received phosphatidylcholine/phosphatidic acid liposomes that had been treated with Mn2+, chlorpromazine or procainamide; the titers of antibodies anti-non-bilayer phospholipid arrangements in these three groups of mice were significantly higher (p < 0.0001, two-way ANOVA with Bonferroni post-tests) than in control mice at the three time points analysed. Moreover, the titers of antibodies induced by chlorpromazine-treated liposomes were significantly higher (p < 0.0001) than those induced by Mn2+-treated or procainamide-treated liposomes at the three time points analysed. The levels of anti-cardiolipin, anti-histone and lupus anticoagulant antibodies in these four groups of mice are shown in . The titers of these antibodies in mice that received liposomes with non-bilayer phospholipid arrangements induced by Mn2+, chlorpromazine or procainamide are significantly higher (p < 0.0001) than in control mice at the second and third months after liposome administration. Chlorpromazine-treated liposomes induced significantly higher titers of anti-cardiolipin (p < 0.0001), anti-histone (p < 0.0001) and lupus anticoagulant antibodies (p < 0.0001) than those induced by Mn2+-treated or procainamide-treated liposomes at the second and third months after liposome administration. Moreover, procainamide-treated liposomes induced higher titers of anti-histone antibodies (p < 0.0001) than Mn2+-treated liposomes at the second and third months after liposome administration, and higher titers of lupus anti-coagulant antibodies (p < 0.0001) than Mn2+-treated liposomes at the third month after liposome administration. The titers of these four antibodies remained constant for at least six months.
Figure 7. Sera of mice with the autoimmune disease have antibodies that bind non-bilayer phospholipid arrangements, as well as anti-cardiolipin, anti-histone and lupus anticoagulant antibodies. Mice (10 per group) received phosphatidylcholine/phosphatidic acid liposomes alone (controls) or treated with Mn2+, chlorpromazine or procainamide. The titers of antibodies that bind non-bilayer phospholipid arrangements (A), anti-cardiolipin antibodies (B), anti-histone antibodies (C) and lupus anticoagulant antibodies (D) were determined before the administration of liposomes, and 1, 2 and 3 months after liposome administration. Data were analysed by two-way ANOVA with Bonferroni post-tests.
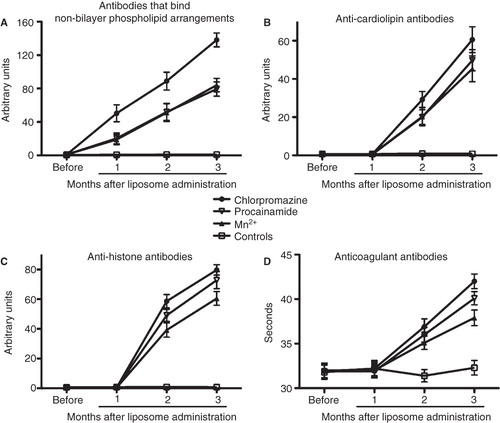
Phosphorylcholine and glycerolphosphorylcholine prevent the binding of sera of mice with the autoimmune disease to non-bilayer phospholipid arrangements
Sera of mice with the autoimmune disease caused by Mn2+-treated or chlorpromazine-treated phosphatidylcholine/phosphatidic acid liposomes were assayed with the same haptens that were used with the H308-AP monoclonal antibody. Only phosphorylcholine and glycerolphosphorylcholine prevented the binding of these sera to non-bilayer phospholipid arrangements induced by Mn2+, chlorpromazine or procainamide in phosphatidylcholine/cardiolipin liposomes (data not shown).
Discussion
In a previous study, we demonstrated that an autoimmune disease resembling human lupus was induced in syngeneic BALB/c and non-syngeneic NIH mice by the administration of liposomes with non-bilayer phospholipid arrangements induced by chlorpromazine or procainamide. A similar illness was produced by injection of chlorpromazine or procainamide, or by injection of the H308-AP monoclonal antibody (Baeza et al. Citation2004). These results led us to propose that non-bilayer phospholipid arrangements, either on the surface of liposomes or on the surface of cells (induced by chlorpromazine or procainamide, or stabilized by the H308-AP monoclonal antibody) can induce the development of an autoimmune disease. However, it remained possible that other effects of chlorpromazine or procainamide, not related to the induction of non-bilayer phospholipid arrangements, were responsible for the disease induction. To address this issue, in the present study we demonstrate that liposomes with non-bilayer phospholipid arrangements induced by Mn2+ can develop a similar disease. Moreover, the physical properties of these liposomes are similar to liposomes with non-bilayer phospholipid arrangements induced by chlorpromazine or procainamide, and their immune reactivity is also similar.
Freeze-fracture electron microscopy and 31P NMR spectroscopy showed that the non-bilayer phospholipid arrangements induced by Mn2+ were smaller (3.7 nm) than those induced by chlorpromazine (5.5 nm), which is in agreement with the bigger size of this drug. The non-bilayer phospholipid arrangements induced by chlorpromazine and procainamide had fusogenic properties, which was not the case of the non-bilayer phospholipid arrangements induced by Mn2+, probably because of their smaller size. 31P NMR spectroscopy also showed evidence of increased transport of Mn2+ to the inside of phosphatidylcholine/cardiolipin liposomes with non-bilayer phospholipid arrangements induced by this cation. Increased transport of Mn2+ was not observed in liposomes made of phosphatidylcholine alone, which suggests that HII-preferring lipids (such as cardiolipin) are needed to form non-bilayer phospholipid arrangements. Similar findings were attained with liposomes containing the HII-preferring lipids phosphatidic acid or phosphatidylserine.
The detection of non-bilayer phospholipid arrangements on liposomes by flow cytometry (as an increase in SSC signal) correlated with the detection of these arrangements by freeze-fracture electron microscopy and 31P NMR spectroscopy. A combination of these three experimental approaches led us to conclude that chlorpromazine is more efficient than Mn2+ for the induction of non-bilayer phospholipid arrangements, and Mn2+ is more efficient than procainamide. This differential efficiency is also reflected in our mouse model of autoimmune disease: Chlorpromazine-treated liposomes induce higher titers of antibodies that bind non-bilayer phospholipid arrangements, and also of anti-cardiolipin, anti-histone and lupus anticoagulant antibodies, than Mn2+-treated or procainamide-treated liposomes. However, procainamide-treated liposomes induce higher titers of anti-histone and lupus anticoagulant antibodies than Mn2+-treated liposomes.
H308-AP is a monoclonal antibody that binds specifically to non-bilayer phospholipid arrangements induced by chlorpromazine or procainamide, but not to smooth liposomes (Baeza et al. Citation2004). Here we show that H308-AP also binds to non-bilayer phospholipid arrangements induced by Mn2+, which suggests the phospholipid re-arrangement induced by these drugs and by Mn2+ is similar.
The ability to form non-bilayer phospholipid arrangements in the presence of Mn2+, chlorpromazine or procainamide, and the increased permeability to Mn2+, reflect the dynamic behaviour of liposomes made of phosphatidylcholine and a HII-preferring lipid (phosphatidic acid, phosphatidylserine or cardiolipin); these phospholipids are among the most abundant in the cell membranes (Voet and Voet Citation2011). In contrast, liposomes made of phospholipids with a higher content of saturated fatty acids (such as dipalmitoylphosphatidylcholine and dipalmitoylphosphatidic acid) are more rigid and do not form non-bilayer phospholipid arrangements in the presence of Mn2+ or procainamide. However, chlorpromazine induces non-bilayer phospholipid arrangements on these rigid liposomes, which could be attributed to its large non-polar region compared with procainamide () and confirms that this drug is the most efficient inducer of non-bilayer phospholipid arrangements, among the inducers that we tested.
Figure 8. Molecular organization of the non-bilayer phospholipid arrangements that induce an autoimmune disease resembling human lupus in mice. Chemical structure and molecular shape of chlorpromazine (A), procainamide (B) and chloroquine (C). (D) Inverted micelle with chlorpromazine. (E) Inverted micelle with Mn2+. (F) Inverted micelle with chlorpromazine inserted in the bilayer arrangement of the liposome, forming the non-bilayer phospholipid arrangement. The bilayer is mainly formed by phosphatidylcholine, whose polar regions are exposed on the zones of the lipid bilayer where the inverted micelle is inserted. The increased exposure facilitates the production of antibodies against these regions.
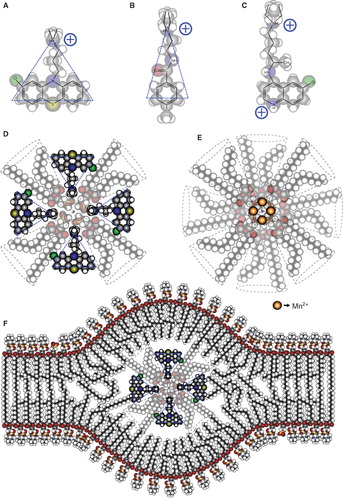
Liposomes made of phosphatidylcholine and a HII-preferring lipid (phosphatidic acid, phosphatidylserine or cardiolipin) have a smooth surface, but they can form non-bilayer phospholipid arrangements in the presence of Mn2+, chlorpromazine or procainamide. As a result, these liposomes are useful to study immune responses against lipids in a particular three-dimensional configuration (i.e., non-bilayer phospholipid arrangements), independently of the phospholipid chemical structures. We observed that liposomes with non-bilayer phospholipid arrangements induced by Mn2+ cause an autoimmune disease in mice, which presents histopathological abnormalities in the skin and kidneys similar to those observed in the disease caused by liposomes with non-bilayer phospholipid arrangements induced by chlorpromazine or procainamide. These results strongly suggest that chlorpromazine and procainamide produce an autoimmune disease by inducing the formation of non-bilayer phospholipid arrangements, and not by some other collateral activity (Zhou and Lu Citation2008, Vedove et al. Citation2009, Chang and Gershwin Citation2010, Tucci et al. Citation2010).
We suggest that liposomes with non-bilayer phospholipid arrangements induce the production of antibodies that specifically bind these arrangements in mice. These antibodies could induce cell damage, which would expose many auto-antigens, such as cardiolipin, histones, nucleus and DNA, with the consequent production of auto-antibodies. Interestingly, we observed that antibodies that bind non-bilayer phospholipid arrangements appear 4 weeks before the antibodies against cardiolipin and histones and lupus anticoagulant antibodies. Antibodies that bind non-bilayer phospholipid arrangements are pathogenic, because we have previously shown that the administration of H308-AP monoclonal antibody causes the development of an autoimmune disease in mice, and this disease is similar to the one caused by administration of liposomes with non-bilayer phospholipid arrangements (Baeza et al. Citation2004).
The sera of mice with the autoimmune disease contained antibodies that bind specifically to non-bilayer phospholipid arrangements. Moreover, haptens that form part of the polar region of the HII-preferring lipids phosphatidic acid, phosphatidylserine and cardiolipin do not block the binding of these sera to non-bilayer phospholipid arrangements. However, haptens that form part of the polar region of the zwitterionic phospholipid phosphatidylcholine block the binding of these sera to non-bilayer phospholipid arrangements; and this is true for the sera of mice that were immunized with liposomes with non-bilayer phospholipid arrangements induced by Mn2+, chlorpromazine or procainamide. These results confirm that the three types of non-bilayer phospholipid arrangements that we analyzed have a similar three-dimensional arrangement. The haptens phosphorylcholine and glycerolphosphorylcholine, which form part of the polar region of the phosphatidylcholine, are the only haptens that also blocked the binding of H308-AP to non-bilayer phospholipid arrangements.
According to these results, we propose a model in which the HII-preferring lipids phosphatidic acid, phosphatidylserine and cardiolipin, which have a negative charge, can form an inverted micelle by electrostatic interactions with the positive charge of Mn2+, chlorpromazine or procainamide (). It is also possible that van der Waals forces between the non-polar groups of the phospholipids and the drugs contribute to the stability of the inverted micelle. The outer layer of this inverted micelle is hydrophobic, so it is immediately inserted into the lipid bilayer of the liposome. The bilayer is mainly formed by phosphatidylcholine, and the polar regions of this phospholipid are exposed on the zones of the lipid bilayer where the inverted micelle is inserted (), facilitating the production of antibodies against them. The formation of non-bilayer phospholipid arrangements had been previously proposed in relation with the fusion of cell membranes, but their molecular association has not been analyzed (Cullis et al. Citation1980, Hope and Cullis Citation1980, Hafez et al. Citation2000). Chloroquine, a divalent cationic drug with a different distribution of its positive charges () than the monovalent cationic drugs chlorpromazine and procainamide inhibits the formation of non-bilayer phospholipid arrangements on liposomes.
Some studies have demonstrated that the tubular hexagonal II phase of phospholipids can induce the formation of antibodies; for example, Rauch and Janoff (Citation1990) showed that phosphatidylethanolamine in hexagonal II phase, but not in bilayer phase, is capable of inducing anti-phospholipid antibodies with lupus anticoagulant activity in BALB/c mice. Further studies demonstrated that human hybridomas (Rauch et al. Citation1986) and lupus anticoagulant antibodies from patients with systemic lupus erythematosus (Rauch et al. Citation1998) recognize hexagonal II associations but not bilayer arrangements of phosphatidylethanolamine. However, extensive areas of phospholipids in HII tubular associations do not occur under physiological conditions, and non-bilayer phospholipid arrangements embedded in the bilayer matrix of liposomes are more representative of the structure and function of cell membranes.
After the administration of liposomes with non-bilayer phospholipid arrangements, mice develop an autoimmune disease that shows histopathological abnormalities and a pattern of auto-antibody production resembling those observed in human lupus. The autoimmune disease in mice is transient and reverts if the administration of liposomes with non-bilayer phospholipid arrangements is discontinued for several months, as is the case with drug-induced human lupus. Three factors support a role of non-bilayer phospholipid arrangments in the pathogenesis of human lupus: (1) Phosphatidylcholine is the most abundant phospholipid in cell membranes, (2) chlorpromazine and procainamide (inducers of non-bilayer phospholipids) induce a lupus like syndrome in humans (Sammaritano Citation2000, Chang and Gershwin Citation2010), and (3) chloroquine (an inhibitor of non-bilayer phospholipids) is beneficial for the treatment of lupus (López et al. Citation2010).
Drug-induced lupus is a complex disease, and several mechanisms have been proposed to explain the production of auto-antibodies characteristic of this disease, including suppression of central and peripheral tolerance, alteration of gene transcription in T and B cells, abnormal cytokine and cytokine receptor balance and function, modification of chromatin structure and modification of self antigens (Chang and Gershwin Citation2010). The results of the present work suggest that the alteration of the membrane phospholipid bilayer of cells by stable non-bilayer phospholipid arrangements (which in a way represents the modification of a self antigen) could be a significant factor in the development of this disease.
Conclusions
The findings of this study demonstrate that non-bilayer phospholipid arrangements induced by Mn2+ have similar physical properties and immune reactivity to non-bilayer phospholipid arrangements induced by chlorpromazine and procainamide, and that all these non-bilayer phospholipid arrangements induce an autoimmune disease resembling human lupus in mice. Our results are consistent with a model in which the HII-preferring lipid (phosphatidic acid, phosphatidylserine or cardiolipin) forms an inverted micelle with Mn2+, chlorpromazine or procainamide, and that this inverted micelle is inserted into the lipid bilayer, causing the exposure of the polar regions of phosphatidylcholine.
The three-dimensional structure of non-bilayer phospholipid arrangements is the same, regardless of the phospholipid composition and the inducer used (Mn2+, chlorpromazine or procainamide), and all the types on non-bilayer phospholipid arrangements that we tested led to the development of similar histopathological abnormalities and auto-antibody production in mice.
Acknowledgements
We thank Isabel Wong-Baeza for discussion and assistance in the preparation of the English manuscript.
Declaration of interest : This study was supported by grants from the National Polytechnic Institute, Mexico (SIP20101117 to I.B., SIP20101111 to C.W. and SIP20100416 to M.I.). I.B, M.I. and C.W. are listed as authors in the following patents: (1) Baeza I, Aguilar L, Wong C, Ibáñez M, Lara M. (2004). US Patent 6,777,193 B1. Methods for diagnostic and/or treatment of antiphospholipid antibodies-related diseases and devices. (2) Baeza I, Aguilar L, Wong C, Ibáñez M, Lara M. (2011). US Patent 7,867,723 B2. Methods for antiphospholipid syndrome. The authors report no conflicts of interest. The authors alone are responsible for the content and writing of the paper.
References
- Aguilar L, Ortega-Pierres G, Campos B, Fonseca R, Ibáñez M, Wong C, 1999. Phospholipid membranes form specific nonbilayer molecular arrangements that are antigenic. J Biol Chem 274:25193–25196.
- Baeza I, Wong C, Ibáñez M, Mondragón R, González S, Farfán N, 1994. Transbilayer diffusion of divalent cations into liposomes mediated by lipidic particles of phosphatidate. J Mol Evol 39:560–568.
- Baeza I, Aguilar L, Alvarado-Aleman F, Escobar-Gutierrez A, Mondragón R, González S, 1995. Identification of phosphatidate nonlamellar phases on liposomes by flow cytometry. Biochem Cell Biol 73:289–297.
- Baeza I, Leyva E, Campos B, Lara M, Ibáñez M, Farfán N, 2004. Antibodies to nonbilayer phospholipid arrangements induce a murine autoimmune disease resembling human lupus. Eur J Immunol 34:1–11.
- Campos B, Chames M, Lantry JM, Bill JP, Eis A, Brockman D, 2006. Determination of non-bilayer phospholipid arrangenments and their antibodies in placentae and sera of patients with hypertensive disorders of pregnancy. Placenta 27:215–224.
- Chang C, Gershwin M. 2010. Drugs and autoimmunity – a contemporary review and mechanism approach. J Autoimmun 34:J266–J275.
- Corona-Ortega T, Rangel-Corona R, Hernández-Jiménez M, Baeza I, Ibáñez M, Weiss-Steider B. 2009. Characterization of cationic liposomes having IL-2 expressed on their external surface, and their affinity to cervical cancer cells expressing the IL-2 receptor. J Drug Target 17:496–501.
- Cullis PR, De Kruijff B, Hope MJ, Nayar R, Schmid SL. 1980. Phospholipids and membrane transport. Can J Biochem 58:1091–1100.
- Cullis PR, Tilcock CP, Hope MJ. 1991. Lipid polymorphism. In: Wilschut J, Hoekstra D, editors. Membrane fusion. New York: Marcel Dekker. pp 35–64.
- de Kruijff B, Verkley AJ, van Echteld CJ, Gerritsen WJ, Mombers C, Noordam PC, 1979. The occurrence of lipidic particles in lipid bilayers as seen by 31P NMR and freeze-fracture electron-microscopy. Biochim Biophys Acta 555:200–209.
- Hafez IM, Ansell S, Cullis PR. 2000. Tunable pH-sensitive liposomes composed of mixtures of cationic and anionic lipids. Biophys J 79:1438–1446.
- Hafez IM, Cullis PR. 2001. Roles of lipid polymorphism in intracelular delivery. Adv Drug Deliv Rev 47:139–148.
- Hope MJ, Cullis PR. 1980. Effects of divalent cations and pH on phosphatidylserine model membranes: A 31P-NMR study. Biochem Biophys Res Commun 92:846–852.
- Ibáñez M, Gariglio P, Chávez P, Santiago R, Wong C, Baeza I. 1996. Spermidine-condensed DNA and cone-shaped lipids improve delivery and expression of exogenous DNA transfer by liposomes. Biochem Cell Biol 74:633–643.
- López P, Gutierrez C, Suárez A. 2010. IL-10 and TNFα genotypes in SLE. J Biomed Biotechnol 2010:838390–838401.
- Madine J, Hughes E, Doig AJ, Middleton DA. 2008. The effects of α-synuclein on phospholipid vesicle integrity: A study using 31P NMR and electron microscopy. Mol Membr Biol 25:518–527.
- Rauch J, Janoff AS. 1990. Phospholipid in the hexagonal II phase is immunogenic: Evidence for immunorecognition of nonnilayer lipid phases in vivo. Proc Natl Acad Sci USA 87:4112–4114.
- Rauch J, Tannenbaum M, Tannenbaum H, Ramelson H, Cullis PR, Tilcock CP, 1986. Human hybridoma lupus anticoagulant distinguish between lamellar and hexagonal phase lipid systems. J Biol Chem 261:9672–9677.
- Rauch J, Tannenbaum M, Neville C, Fortin PR. 1998. Inhibition of lupus anticoagulant activity by hexagonal phase phosphatidylethanolamine in the presence of prothrombin. Thromb Haemost 80:936–941.
- Sammaritano LR. 2000. Drug-induced antiphospholipid antibodies. In: Khamashta MA, editor. Hughes syndrome, Antiphospholipid syndrome. London: Springer-Verlag. pp 144–154.
- Singh D, Jarrel HC, Barber KR, Grant CW. 1992. Glycosphingolipids: 2H NMR study of the influence of ceramide fatty acid characteristics on the carbohydrate headgroup in phospholipid bilayers. Biochemistry 31:2662–2669.
- Smaal EB, Nicolay K, Mandersloot JG, de Gier J, de Kruijff B. 1987. 2H-NMR, 31P-NMR and DSC characterization of a novel lipid organization in calcium-dioleoylphosphatidate membranes. Implications for the mechanism of the phosphatidate calcium transmembrane shuttle. Biochim Biophys Acta 897:453–466.
- Spink CH. 2008. Differential scanning calorimetry. In Correia J, Detrich H, editor. Biophysical tools for biologists. Boston: North-eastern University, 84:115–141.
- Szoka F Jr, Papahadjopoulos D. 1978. Procedure for preparation of liposomes with large internal aqueous space and high capture by reverse-phase evaporation. Proc Natl Acad Sci USA 75:4194–4198.
- Tucci M, Stucci S, Strippoli S, Silvestris F. 2010. Cytokine overproduction, T-Cell activation, and defective T-regulatory functions promote nephritis in systemic lupus erythematosus. J Biomed Biotechnol 2010:457146–457152.
- van den Brink-van der Laan E, Dalbey RE, Demel RA, Killian JA, de Kruijff B. 2001. Effect of nonbilayer lipids on membrane binding and insertion of catalytic domain of leader peptidase. Biochemistry 40:9677–9684.
- Vedove CD, Del Giglio M, Schena D, Girolomoni G. 2009. Drug-induced lupus erythematosus. Arch Dermatol Res 301:99–105.
- Verkleij AJ, de Maagd R, Levnissen-Bijvelt J, de Kruijff B. 1982. Divalent cations and chlorpromazine can induce non-bilayer structures in phosphatidic acid-containing model membranes. Biochim Biophys Acta 684:255–262.
- Verkleij AJ. 1991. Role of non-bilayer lipids in membrane fusion. In: Wilschut J, Hoekstra D, editors. Membrane fusion. New York: Marcel Dekker. pp 155–181.
- Voet D, Voet JG. 2011. Biochemistry. New York, USA: John Wiley & Sons, Inc.
- Wegener J, Galla HJ. 1996. The role of non-lamellar lipid structures in the formation of tight junctions. Chem Phys Lipids 81:229–255.
- Wong-Baeza C, Bustos I, Serna M, Tescucano A, Alcántara-Farfán V, Ibáñez M, 2010. Membrane fusion inducers, chloroquine and spermidine increase lipoplex-mediated gene transfection. Biochem Biophys Res Commun 396:549–554.
- Young IT. 1987. Proof without prejudice: Use of the Kolmogorov-Smirnov test for the analysis of histograms from flow systems and other sources. J Histochem Cytochem 7:935–941.
- Zhou Y, Lu Q. 2008. DNA methylation in T cells from idiopathic lupus and drug-induced lupus patients. Autoimmun Rev 7:376–383.