Abstract
Corticotropin-releasing factor (CRF) is a major regulatory peptide in the hypothalamic–pituitary–adrenal (HPA) axis under stress conditions. In response to stress, CRF is produced in the hypothalamic paraventricular nucleus. Forskolin- or pituitary adenylate cyclase-activating polypeptide-stimulated CRF gene transcription is mediated by the cyclic AMP (cAMP) response element on the CRF 5′-promoter region. Estrogens enhance activation of the CRF gene in stress, while inducible cAMP-early repressor suppresses the stress response via inhibition of the cAMP-dependent CRF gene. Glucocorticoid-dependent repression of cAMP-stimulated CRF promoter activity is mediated by both the negative glucocorticoid-response element and the serum-response element, while interleukin-6 (IL-6) stimulates the CRF gene. Suppressor of cytokine signaling-3, stimulated by IL-6 and cAMP, is involved in the negative regulation of CRF gene expression. Such complex mechanisms contribute to stress responses and homeostasis in the hypothalamus. Moreover, disruption of the HPA axis may cause a number of diseases related to stress. For example, CRF-induced p21-activated kinase 3 mRNA expression may be related to the proliferation of corticotrophs in Nelson's syndrome. A higher molecular weight form of immunoreactive β-endorphin, putative proopiomelanocortin (POMC), is increased in CRF-knockout mice, suggesting the important role of CRF in the processing of POMC through changes in prohormone convertase type-1 expression levels.
Introduction
The hypothalamic–pituitary–adrenal (HPA) axis is activated by various stressors. Corticotropin-releasing factor (CRF) plays a central role in controlling the stress response, and regulates the HPA axis (Vale et al. Citation1981) by stimulating the synthesis of adrenocorticotropic hormone (ACTH) from the anterior pituitary (AP) (Vale et al. Citation1981; ). Impaired adrenal responses in CRF-knockout mice (CRF KO) were observed in response to various acute stressors such as restraint, ether, and fasting (Muglia et al. Citation1994, Citation1995, Citation1997). In addition, the ACTH response to pain stress in CRF KO was also smaller than that in wild-type mice (WT; ), suggesting the effects of CRF on the augmented release of ACTH from the pituitary in response to stress (Fukuda et al. Citation2004).
Figure 1. A schematic model of the hypothalamic–pituitary–adrenal (HPA) axis. CRF plays a central role in controlling stress responses. CRF, produced in the hypothalamic PVN, stimulates ACTH production from the corticotrophs of the anterior pituitary (AP). ACTH then stimulates glucocorticoid release from the adrenal glands. Glucocorticoids, in turn, inhibits both CRF production in hypothalamus and the ACTH production in the AP.
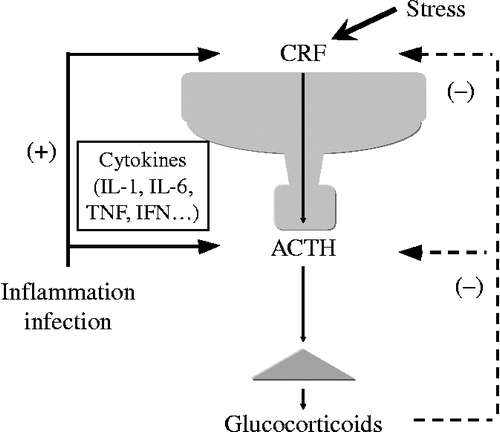
Figure 2. Plasma ACTH concentrations of WT and CRF KO. The basal ACTH concentrations were determined under pentobarbital anesthesia (n = 6–7). ACTH concentrations under pain stress were determined after tail-pinched stress for 15 s (n = 7–8); NS, not significant; *P < 0.05 and **P < 0.01 (compared between the two values indicated). [Reproduced with permission from Fukuda et al. (Citation2004). Copyright © Elsevier.]
![Figure 2. Plasma ACTH concentrations of WT and CRF KO. The basal ACTH concentrations were determined under pentobarbital anesthesia (n = 6–7). ACTH concentrations under pain stress were determined after tail-pinched stress for 15 s (n = 7–8); NS, not significant; *P < 0.05 and **P < 0.01 (compared between the two values indicated). [Reproduced with permission from Fukuda et al. (Citation2004). Copyright © Elsevier.]](/cms/asset/f19ac492-eae1-43d4-9b2c-334875525781/ists_a_536279_f0002_b.gif)
Limbic structures, such as the extended amygdala and the bed nuclei of the stria terminalis, have an important role in stress responses. In the hypothalamic paraventricular nucleus (PVN) of the brain, CRF is produced in response to stress. CRF and arginine vasopressin (AVP) neurons in the parvocellular region of the PVN project to the external zone of the median eminence. CRF and AVP in parvocellular PVN neurons exert synergistic effects on ACTH secretion from the AP (Gillies et al. Citation1982; Mouri et al. Citation1993). On the other hand, ACTH stimulates the release of glucocorticoids from the adrenal glands (). Circulating glucocorticoids are critical for recovery from stress conditions because they inhibit the hypothalamic PVN production of CRF and the pituitary secretion of ACTH, thereby ensuring that serum levels of glucocorticoids are appropriate to the stress experienced ().
Stimulation of the CRF gene in the hypothalamus
Several candidates have been implicated in the activation of CRF neurons. For example, pituitary adenylate cyclase-activating polypeptide (PACAP), a member of the secretin/glucagon/vasoactive intestinal peptide family, is one putative hormone. The role of PACAP in the PVN of the hypothalamus has not been clearly determined. The extended amygdala and the bed nuclei of the stria terminalis are identified as innervation sites of PACAP neurons, suggesting that it may play an important role in stress responses. PACAP may contribute to the response to stress through stimulation of CRF and AVP neurons of the hypothalamus. Both PACAP and the PACAP-selective PACAP receptor type 1 are highly expressed in the hypothalamus, including the parvocellular and magnocellular subdivisions of the PVN, and the supraoptic nucleus (SON; Nomura et al. Citation1996; Shioda et al. Citation1997). PACAP has been shown to stimulate cyclic AMP (cAMP) production in the AP (Miyata et al. Citation1989) and also increases CRF mRNA levels in the parvocellular region of the PVN, suggesting its involvement in the positive regulation of CRF gene expression (Grinevich et al. Citation1997). The cAMP protein kinase A (PKA) pathway plays a main role in stimulating CRF synthesis by PACAP (Kageyama et al. Citation2007).
Computational analysis of the proximal CRF promoter reveals several putative-binding sites for transcriptional factors such as cAMP-response element (CRE), activator protein 1 (AP-1) protein (Fos/Jun)-binding sites, half glucocorticoid regulatory element (GRE), and half estrogen-responsive element (; Yao and Denver Citation2007). Forskolin or PACAP stimulates adenylate cyclase and then intracellular cAMP levels in hypothalamic 4B cells (; Kageyama et al. Citation2007). Forskolin increases CRF transcriptional activity in hypothalamic cells (), which corresponds with the previous studies using other cells (Seasholtz et al. Citation1988; Spengler et al. Citation1992; Yamamori et al. Citation2004). The forskolin-stimulated activity of CRF gene transcription is reduced in 4B cells transfected with a mutant construct, CRF-233Mtluc, in which the CRE element is mutated, and in a deletion mutant construct of the CRF gene promoter CRF-220luc (; Kageyama et al. Citation2008). This suggests that forskolin-induced CRF gene transcription is mainly mediated by CRE, which includes − 220 to − 233 bp, on the CRF 5′-promoter region in hypothalamic cells.
Figure 4. Effects of PACAP on cAMP production in hypothalamic 4B cells. Cells were pre-incubated for 20 min with the medium containing 0.1 mM 3-isobutyl-1-methylxanthine, followed by the addition of forskolin (Fsk) or PACAP. The level of intracellular cAMP was measured by cAMP EIA; *P < 0.05 and **P < 0.005 (compared with control (C)). [Reproduced with permission from Kageyama et al. (Citation2007). Copyright © Society for Endocrinology.]
![Figure 4. Effects of PACAP on cAMP production in hypothalamic 4B cells. Cells were pre-incubated for 20 min with the medium containing 0.1 mM 3-isobutyl-1-methylxanthine, followed by the addition of forskolin (Fsk) or PACAP. The level of intracellular cAMP was measured by cAMP EIA; *P < 0.05 and **P < 0.005 (compared with control (C)). [Reproduced with permission from Kageyama et al. (Citation2007). Copyright © Society for Endocrinology.]](/cms/asset/aab83192-5800-47c9-bb39-8e45de327412/ists_a_536279_f0004_b.gif)
Figure 5. Effects of CRE deletion on forskolin-induced CRF 5′-promoter activity in 4B cells. (A) Schematic representation of CRE in the CRF promoter. (B) Cells were transfected with full-length (CRF-907luc), deleted (CRF-220luc or CRF-233luc), or mutant (CRF-233Mtluc) promoter constructs then incubated for 2 h with 10 μM forskolin alone (Fsk) or vehicle (C); *P < 0.05 (compared with forskolin alone [Fsk] in CRF-907luc transfected cells) and +P < 0.05 (compared with each control). [Reproduced with permission from Kageyama et al. (Citation2008). Copyright © Editrice Kurtis srl.]
![Figure 5. Effects of CRE deletion on forskolin-induced CRF 5′-promoter activity in 4B cells. (A) Schematic representation of CRE in the CRF promoter. (B) Cells were transfected with full-length (CRF-907luc), deleted (CRF-220luc or CRF-233luc), or mutant (CRF-233Mtluc) promoter constructs then incubated for 2 h with 10 μM forskolin alone (Fsk) or vehicle (C); *P < 0.05 (compared with forskolin alone [Fsk] in CRF-907luc transfected cells) and +P < 0.05 (compared with each control). [Reproduced with permission from Kageyama et al. (Citation2008). Copyright © Editrice Kurtis srl.]](/cms/asset/1a08b529-388a-4a0f-8f43-a8c476043a76/ists_a_536279_f0005_b.gif)
The CRE on the promoter is also regulated both by glucocorticoids and glucocorticoid withdrawal. We observed that the intracellular cAMP production levels were transiently increased 30 min after the removal of dexamethasone, while they were also increased 2.5 h after incubation with dexamethasone without the removal (Kageyama et al. Citation2010). Phosphorylated-CREB/CREB protein levels were rapidly increased via an adenylate cyclase pathway after the deprivation of glucocorticoids. Therefore, the phosphorylation of CREB may contribute to the activation of CRF gene transcription after the deprivation of glucocorticoids in hypothalamic cells.
The PKA pathway is mainly involved in cAMP-dependent CRF gene regulation in hypothalamic cells, since a PKA inhibitor strongly blocks forskolin-induced CRF promoter activity (Agarwal et al. Citation2005). This result supports our previous report on the involvement of the PKA pathway in CRF synthesis and release in hypothalamic explants (Suda et al. Citation1985). Activation of the PKA pathway leads to the binding of CRE-binding protein (CREB) to the CRE on the CRF promoter in hypothalamic cells as well as in human placental cells (Cheng et al. Citation2000). Protein kinase C (PKC) and p38 mitogen-activated protein (MAP) kinase are also partially involved in the positive regulation of forskolin-induced CRF gene expression in rat hypothalamic cells (Kageyama et al. Citation2008). Thus, PKA, PKC, and p38 MAP kinase pathways are all involved in forskolin-induced activation of CRF gene transcription in hypothalamic 4B cells.
Estrogen regulates the HPA axis by stimulating CRF gene expression in the hypothalamus in vivo, since high levels of estrogen replacement increased basal levels of CRF mRNA in the PVN of ovariectomized rats (Ochedalski et al. Citation2007). A physiologically relevant dose (10 nM) of estradiol (E2) stimulates both CRF gene transcription and mRNA expression in hypothalamic 4B cells (Ogura et al. Citation2008). These results suggest that the direct effect of E2 increasing the CRF in PVN, may contribute to the enhanced ACTH and cortisol levels observed in the mid-luteal phase. Activation of CRF neurons, in turn, may affect gonadal function in a bidirectional manner, because estrogens activate the HPA axis, while the estrogen-induced activation of CRF neurons would suppress the release of gonadotropin-releasing hormone. Moreover, E2 and diarylpropionitrile, an estrogen receptor β agonist, increases CRF gene transcriptional activity in hypothalamic cells. Furthermore, treatment with both E2 and forskolin shows an additive effect on CRF promoter activity (Ogura et al. Citation2008) and estrogens enhance activation of the CRF gene by cAMP under stress.
Suppression of the CRF gene in the hypothalamus
The HPA axis is regulated by a negative feedback mechanism. Hypothalamic parvocellular neurons express glucocorticoid receptors (GRs), and glucocorticoids regulate CRF gene expression directly in the hypothalamus. Repression by glucocorticoids was shown to occur through inhibition of CRF gene transcription in a subclone of AtT-20 cells (Malkoski and Dorin Citation1999). The CRF promoter does not contain a classical GRE consensus site, but rather there are a number of regions in the sequence where GRs are able to bind (Guardiola-Diaz et al. Citation1996). Malkoski and Dorin (Citation1999) found glucocorticoid regulatory regions on the CRF promoter, and demonstrated that a specific region of the CRF promoter contributed to the inhibition of CRF promoter activity by glucocorticoids as a negative GRE (nGRE). High-affinity binding of the GR DNA-binding domain to this promoter region was observed with an electrophoretic mobility shift assay.
Other regions of the promoter are also involved in the inhibitory regulation of CRF gene expression in hypothalamic 4B cells (). The glucocorticoid suppression of cAMP-stimulated CRF promoter activity is mediated by binding between − 248 and − 233 bp of the CRF promoter in hypothalamic cells (). A serum-response element (SRE) is included in this region and thus may contribute to the negative response of glucocorticoids, as the GR is able to bind to SREs and inhibit promoter activation by antagonizing the function of positive transcription (Karagianni and Tsawdaroglou Citation1994). Therefore, in addition to an nGRE, the glucocorticoid suppression of cAMP-stimulated CRF promoter activity may also be caused by SRE in hypothalamic 4B cells.
Figure 6. Effects of nGRE or SRE deletion on the dexamethasone suppression of CRF 5′-promoter activity in 4B cells. (A) Schematic representation of nGRE or SRE in the CRF promoter. (B) Cells transfected with a full-length (CRF-907luc), deleted (CRF-233luc, CRF-248luc, or CRF-278luc), or mutant (CRF-278Mtluc or CRF-248Mtluc) promoter construct, were pre-incubated for 30 min with the medium containing dexamethasone (Dex, 100 nM) or vehicle (C), then incubated for 2 h with the medium containing 10 μM forskolin. Data are presented as relative activity; luciferase activity in response to forskolin alone (C) was set at 100% in all transfected cells; *P < 0.05 (compared with forskolin alone [C] in all transfected cells), +P < 0.05 (compared with Dex in CRF-907luc), ++P < 0.05 (compared with Dex in CRF-907luc and CRF-278luc), and +++P < 0.05 (compared with Dex in CRF-907luc, CRF-278luc, and CRF-248luc). [Reproduced with permission from Kageyama et al. (Citation2008). Copyright © Editrice Kurtis srl.]
![Figure 6. Effects of nGRE or SRE deletion on the dexamethasone suppression of CRF 5′-promoter activity in 4B cells. (A) Schematic representation of nGRE or SRE in the CRF promoter. (B) Cells transfected with a full-length (CRF-907luc), deleted (CRF-233luc, CRF-248luc, or CRF-278luc), or mutant (CRF-278Mtluc or CRF-248Mtluc) promoter construct, were pre-incubated for 30 min with the medium containing dexamethasone (Dex, 100 nM) or vehicle (C), then incubated for 2 h with the medium containing 10 μM forskolin. Data are presented as relative activity; luciferase activity in response to forskolin alone (C) was set at 100% in all transfected cells; *P < 0.05 (compared with forskolin alone [C] in all transfected cells), +P < 0.05 (compared with Dex in CRF-907luc), ++P < 0.05 (compared with Dex in CRF-907luc and CRF-278luc), and +++P < 0.05 (compared with Dex in CRF-907luc, CRF-278luc, and CRF-248luc). [Reproduced with permission from Kageyama et al. (Citation2008). Copyright © Editrice Kurtis srl.]](/cms/asset/77d30593-a877-46ea-ae39-8f4397f64005/ists_a_536279_f0006_b.gif)
Binding sites for both GR and AP-1 nucleoproteins have been shown at adjacent elements within the nGRE (Malkoski and Dorin Citation1999), with mutations that disrupt either GR- or AP-1-binding activity causing a similar loss of glucocorticoid-dependent repression. These results suggest that the nGRE works as a composite regulatory element, involving direct DNA-binding of GR and AP-1 nucleoproteins. King et al. (Citation2002) proposed that transcription factor differences among cells may cause negative or positive regulation, because CREB and Fos were detected in AtT-20 cells while CREB and cJun were found in placental cells (King et al. Citation2002). Furthermore, glucocorticoids can inhibit CREB and cFos in the PVN (Jacobson et al. Citation1990; Legradi et al. Citation1997). Modified expression of the transcription factors in cells changes the binding of transcription factors to nGRE and/or CRE, and subsequently alters promoter activity, resulting in the suppression of CRF gene transcription. A recent study showed that the steroid receptor coactivator 1a increases GR-mediated repression, whereas the corepressors, nuclear corepressor and silencing mediator of the retinoid and thyroid hormone receptor, inhibit CREB-dependent induction of the CRF gene (van der Laan et al. Citation2008).
Factors other than glucocorticoids may be involved in limiting the stimulation of CRF during stress, because treatment with glucocorticoids in adrenalectomized rats does not prevent the increase in stress-induced CRF heteronuclear RNA (Ma and Aguilera Citation1999). Inducible cAMP-early repressor (ICER) is a cAMP-inducible member of the CRE modulator (CREM) family and a repressor isoform of CREM (Foulkes et al. Citation1991; Molina et al. Citation1993). CREM, CREB, and activating transcription factor 1 bind to CRE promoter elements (Lalli and Sassone-Corsi Citation1994), while ICER acts as a competitive inhibitor of CRE-dependent transcription (Foulkes et al. Citation1991). ICER suppresses the stress response via inhibition of the cAMP-dependent CRF gene ().
Figure 7. Effects of ICER siRNA on forskolin-induced CRF 5′-promoter activity in 4B cells. Cells were incubated for 24 h in culture medium containing siRNA for either control (siControl) or ICER (siICER). After the incubation, the cells were transfected with CRF promoter constructs, then incubated with 1 μM forskolin alone or vehicle for the durations shown. Forskolin-induced CRF 5′-promoter activity in cells transfected with siControl is shown as open circles, while that in cells transfected with siICER is shown as closed circles; *P < 0.05 (compared with control).
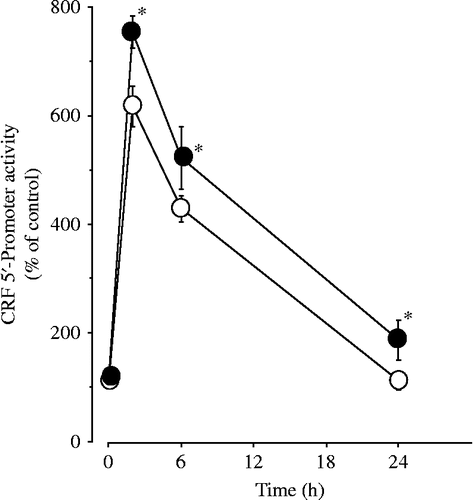
Involvement of interleukin-6 in CRF gene transcription
Interleukin-6 (IL-6) shows a variety of biological activities. Plasma IL-6 levels increase in response to both immune activation and non-immune stress (Zhou et al. Citation1993; Takaki et al. Citation1994). IL-6 promotes lymphocytic proliferation and differentiation (Schimpl and Wecker Citation1972) and induces production of acute-phase proteins in the liver (Castell et al. Citation1989). In addition, IL-6 stimulates the HPA axis (Venihaki et al. Citation2001), resulting in increased glucocorticoid levels, which in turn suppress IL-6 production (Karalis et al. Citation1997; Turnbull and Rivier Citation1999). Therefore, IL-6 is an important mediator of the interaction between neuroendocrine and immune systems.
IL-6 is coexpressed with CRF and AVP in the SON and PVN neurons (Ghorbel et al. Citation2003), and plays an important role in regulating both CRF and AVP in the hypothalamus. For example, IL-6 increases CRF gene expression and secretion in the PVN (Navarra et al. Citation1991; Vallieres and Rivest Citation1999). Forskolin or PACAP stimulates IL-6 protein levels in the hypothalamic cells (). In addition, the stimulatory effects of PACAP on CRF promoter activity are significantly inhibited by treatment with the anti-IL-6 monoclonal antibody in hypothalamic cells (). Therefore, endogenous IL-6 production is also involved in the PACAP-induced CRF gene transcription in an autocrine manner in hypothalamic 4B cells. Considering the delayed response to IL-6, and the partial inhibition of PACAP effects by anti-IL-6 antibody, IL-6 may be important in sustaining the activity of CRF and AVP genes. E2 stimulated IL-6 transcriptional activity, and subsequently the levels of IL-6 mRNA and protein. Thus, it is possible that IL-6, which is produced in hypothalamus, both, directly and indirectly, stimulates CRF and AVP gene expression.
Figure 8. Effects of IL-6 on PACAP-induced CRF 5′-promoter activity in 4B cells. (A) Effects of PACAP on IL-6 production in 4B cells. Cells were incubated for 48 h with medium alone (control), or with medium containing forskolin (F) or PACAP. The titers of IL-6 in the culture supernatants were measured by IL-6 ELISA; **P < 0.005 (compared with control (C)). (B) Effects of anti-IL-6 Ab on PACAP-induced CRF 5′-promoter activity in 4B cells. Cells were pre-incubated with medium containing anti-IL-6 Ab or control IgG for 30 min and then incubated for 2 h with medium containing 10 or 100 nM PACAP or vehicle. Cells treated with control IgG are indicated as C; **P < 0.005 (compared with control (C)), ++P < 0.005 (compared with 100 nM PACAP), and #P < 0.05 (compared with 10 nM PACAP). [Reproduced with permission from Kageyama et al. (Citation2007). Copyright © Society for Endocrinology.]
![Figure 8. Effects of IL-6 on PACAP-induced CRF 5′-promoter activity in 4B cells. (A) Effects of PACAP on IL-6 production in 4B cells. Cells were incubated for 48 h with medium alone (control), or with medium containing forskolin (F) or PACAP. The titers of IL-6 in the culture supernatants were measured by IL-6 ELISA; **P < 0.005 (compared with control (C)). (B) Effects of anti-IL-6 Ab on PACAP-induced CRF 5′-promoter activity in 4B cells. Cells were pre-incubated with medium containing anti-IL-6 Ab or control IgG for 30 min and then incubated for 2 h with medium containing 10 or 100 nM PACAP or vehicle. Cells treated with control IgG are indicated as C; **P < 0.005 (compared with control (C)), ++P < 0.005 (compared with 100 nM PACAP), and #P < 0.05 (compared with 10 nM PACAP). [Reproduced with permission from Kageyama et al. (Citation2007). Copyright © Society for Endocrinology.]](/cms/asset/4b0d0b2f-a573-4348-8d2a-4bf14e3e0941/ists_a_536279_f0008_b.gif)
Suppressor of cytokine signaling-3 (SOCS-3) acts as a potent negative regulator of cytokine signaling (Krebs and Hilton Citation2000), and suppresses cytokine-induced proopiomelanocortin (POMC) gene transcription and ACTH secretion in corticotrophs (Auernhammer et al. Citation1999). IL-6 stimulates the Janus kinase/signal transducers and activators of the transcription (JAK/STAT) signaling pathway, while IL-6-induced SOCS-3 acts as a negative regulator and inhibits STAT phosphorylation by JAK at the receptor complex (Ram and Waxman Citation1999; Schmitz et al. Citation2000). SOCS-3, stimulated by IL-6 and cAMP, was also shown to be involved in the negative regulation of CRF gene expression in hypothalamus ().
Figure 9. Effects of SOCS-3 on the regulation of CRF 5′-promoter activity in 4B cells. Cells were incubated for 24 h in culture medium containing siRNA for either control (siControl) or SOCS (siSOCS). After incubation, the cells were transfected with a CRF promoter construct and then incubated with vehicle (A), 10 μM forskolin (Fsk) for 2 h (B) or 100 ng/mL IL-6 for 24 h (C); *P < 0.05 (compared with control [siControl]). [Reproduced with permission from Kageyama et al. (Citation2009a). Copyright © Society for Endocrinology.]
![Figure 9. Effects of SOCS-3 on the regulation of CRF 5′-promoter activity in 4B cells. Cells were incubated for 24 h in culture medium containing siRNA for either control (siControl) or SOCS (siSOCS). After incubation, the cells were transfected with a CRF promoter construct and then incubated with vehicle (A), 10 μM forskolin (Fsk) for 2 h (B) or 100 ng/mL IL-6 for 24 h (C); *P < 0.05 (compared with control [siControl]). [Reproduced with permission from Kageyama et al. (Citation2009a). Copyright © Society for Endocrinology.]](/cms/asset/9295ff38-de2d-491e-8a6e-2138184bc42d/ists_a_536279_f0009_b.gif)
Role of hypothalamic CRF in regulating pituitary corticotrophs
CRF KO showed severe impairment of the HPA axis, indicating that CRF is required for its regulation. We applied oligonucleotide microarray analysis to the AP of CRF KO to identify gene expression induced by CRF. Twenty-four genes showed less than 60% expression in CRF KO compared with normal mice (). Real-time PCR analysis revealed that p21-activated kinase 3 (Pak3), prohormone convertase type 1 (PC-1), and CRF-binding protein (BP) mRNA expression levels were increased by CRF in AP cells (Kageyama et al. 2009b).
Table I. Microarray analysis in the AP of CRF KO.
The effects of CRF on the proliferation of corticotrophs remain controversial. CRF increased cell viability in AtT-20 cells (Kageyama et al. 2009). This result is consistent with those of other studies showing that CRF induces proliferation of AtT-20 cells (van Wijk et al. Citation1995). However, another report demonstrated that long-term treatment with CRF inhibits the proliferation of AtT-20 cells (Melzig et al. Citation1998), whereas it acts as a mitogen in rat AP cells (Childs et al. Citation1995). The cell cycle is regulated by a specific kinase in pituitary cells (Al-Gahtany et al. Citation2003), for example, p21 is an activator of the cell cycle, while the kinases p27 and p57 inhibit it (Al-Gahtany et al. Citation2003). Paks are serine/threonine kinases implicated in a number of different intracellular processes, including cytoskeletal regulation (Manser et al. Citation1997) and the activation of MAP kinases (Zhang et al. Citation1995). For example, Pak1 signaling mediates cyclin D1 expression, and is up-regulated in human breast tumors (Balasenthil et al. Citation2004). Pak3 mediates both apoptosis and DNA synthesis in neurons, since the mutant Pak3 inhibits neuronal apoptosis and DNA synthesis (McPhie et al. Citation2003). Activators of cellular Paks include the small GTP-BPs Rac and Cdc42, and a variety of external stimuli that act through cell surface receptors, such as G protein-coupled receptors (Knaus et al. Citation1995). Therefore, our findings provide important information with respect to the regulation of Pak3 mRNA levels by CRF in AP (). We also demonstrated that Pak3 knockdown inhibited CRF-induced cell viability (). Although the precise mechanism is still undetermined, CRF-induced Pak3 mRNA expression could be related to the proliferation of corticotrophs in Nelson's syndrome. Nelson's syndrome results from the development of an aggressive ACTH-secreting pituitary adenoma in patients who have undergone bilateral adrenalectomy for Cushing's disease. CRF would be up-regulated in this case (). Pak3 levels in the pituitary of Nelson's syndrome, compared with that of Cushing's disease, should therefore be examined to help confirm the role of Pak3 in corticotrophs.
Figure 10. Effects of CRF on Pak3 mRNA levels. (A) Time-dependent changes in CRF-induced Pak3 mRNA levels. Cells were incubated with medium containing 100 nM CRF for the times indicated. (B) Effects of a CRF receptor antagonist on CRF-induced Pak3 mRNA levels. Cells were pre-incubated with medium containing 1 μM astressin (AST) or vehicle for 30 min and then incubated for 6 h with medium containing 100 nM CRF or vehicle. (C) Effects of Pak3 on CRF-induced AtT-20 cell viability. The AtT-20 cells were incubated for 24 h in culture medium containing siRNA for either control (siCont) or Pak3 (siPak). After incubation, AtT-20 cells were incubated for 48 h in culture medium containing either CRF (10 or 100 nM) or the vehicle (Veh). Cell viability was measured by WST-1 colorimetric assay; *P < 0.005 (compared with control (0 h or C)). [Reproduced with permission from Kageyama et al. (Citation2009b). Copyright © Elsevier.]
![Figure 10. Effects of CRF on Pak3 mRNA levels. (A) Time-dependent changes in CRF-induced Pak3 mRNA levels. Cells were incubated with medium containing 100 nM CRF for the times indicated. (B) Effects of a CRF receptor antagonist on CRF-induced Pak3 mRNA levels. Cells were pre-incubated with medium containing 1 μM astressin (AST) or vehicle for 30 min and then incubated for 6 h with medium containing 100 nM CRF or vehicle. (C) Effects of Pak3 on CRF-induced AtT-20 cell viability. The AtT-20 cells were incubated for 24 h in culture medium containing siRNA for either control (siCont) or Pak3 (siPak). After incubation, AtT-20 cells were incubated for 48 h in culture medium containing either CRF (10 or 100 nM) or the vehicle (Veh). Cell viability was measured by WST-1 colorimetric assay; *P < 0.005 (compared with control (0 h or C)). [Reproduced with permission from Kageyama et al. (Citation2009b). Copyright © Elsevier.]](/cms/asset/e0c7bef3-ef4c-4d60-9729-246fe07cae40/ists_a_536279_f0010_b.gif)
Figure 11. A schematic model of modulation of the HPA axis in Nelson's syndrome. Nelson's syndrome results from the development of an aggressive ACTH-secreting pituitary adenoma in patients who have undergone bilateral adrenalectomy for Cushing's disease.
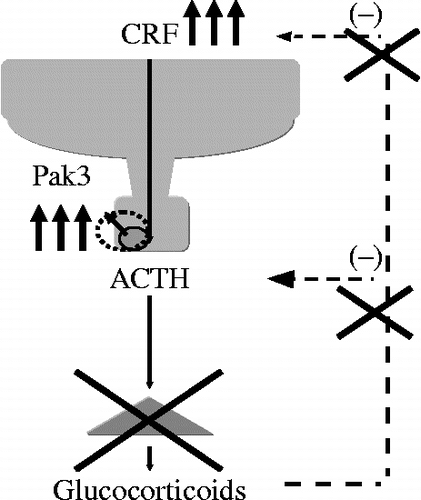
POMC is cleaved to β-lipotropic hormone (β-LPH) and ACTH by the processing enzyme PC-1 (). β-LPH and ACTH are cleaved to β-endorphin (β-EP) and α-melanocyte-stimulating hormone (α-MSH) by PC-2, respectively (). Pituitary POMC mRNA levels are not attenuated (Muglia et al. Citation1995, Citation1997), but ACTH content is decreased in the AP of CRF KO (Muglia et al. Citation2000), suggesting the involvement of CRF in stimulating the processing of POMC. In addition, immunoreactive (ir) β-EP content in the AP of CRF KO is not different from those of WT. Therefore, to determine the different molecular profile of POMC-related peptides between WT and CRF KO, ir β-EP contents extracted from the AP of WT and CRF KO were assayed by gel filtration chromatography. The gel filtration analysis revealed that a higher molecular weight form of ir β-EP, putative POMC (Suda et al. Citation1982), was increased in CRF KO, but the β-EP peak level was small and similar between the two groups (). These results suggest that CRF affects the PC-1 in the AP. In fact, our study shows that PC-1 gene expression levels are dependent on CRF (). Therefore, CRF is able to modulate the processing of POMC through changes in PC-1 expression levels.
Figure 12. Processing of POMC in the AP of CRF KO. (A) Processing of POMC to the POMC-related peptides in the AP. POMC-related peptides are obtained by PC-1 and PC-2 in the AP. In the processing of POMC to NT, JP, ACTH, and β-LPH are mainly produced by the several steps of PC-1, whereas γ-LPH and β-EP are a little produced by PC-2. As β-EP Ab has cross-reactivities with POMC and β-LPH (shown as a dark block square), ir β-EP contains POMC, β-LPH and β-EP, which were previously determined. NT, N-terminal peptide; JP, joining peptide; MSH, melanocyte stimulating hormone; CLIP, corticotropin-like intermediate lobe peptide; LPH, lipotropic hormone; EP, endorphin; PC, prohormone convertase. (B) Representative elution profiles of ir β-EP contents extracted from the AP of WT and CRF KO by Sephadex G-50 gel filtration chromatography. The gel filtration analyses revealed that a higher molecular weight form of ir β-EP, putative POMC, was increased in CRF KO, but the β-EP peak level was small and similar between the two groups. Vo, void volume; LPH, lipotropic hormone; EP, endorphin. (C) POMC/β-LPH ratio in the AP of WT and CRF KO by Sephadex G-50 gel filtration chromatography. POMC/β-LPH ratio of anterior pituitary from individual mice are shown as the mean ± SEM (n = 4); *P < 0.001 (compared with WT). [Reproduced with permission from Fukuda et al. (Citation2004). Copyright © Elsevier.]
![Figure 12. Processing of POMC in the AP of CRF KO. (A) Processing of POMC to the POMC-related peptides in the AP. POMC-related peptides are obtained by PC-1 and PC-2 in the AP. In the processing of POMC to NT, JP, ACTH, and β-LPH are mainly produced by the several steps of PC-1, whereas γ-LPH and β-EP are a little produced by PC-2. As β-EP Ab has cross-reactivities with POMC and β-LPH (shown as a dark block square), ir β-EP contains POMC, β-LPH and β-EP, which were previously determined. NT, N-terminal peptide; JP, joining peptide; MSH, melanocyte stimulating hormone; CLIP, corticotropin-like intermediate lobe peptide; LPH, lipotropic hormone; EP, endorphin; PC, prohormone convertase. (B) Representative elution profiles of ir β-EP contents extracted from the AP of WT and CRF KO by Sephadex G-50 gel filtration chromatography. The gel filtration analyses revealed that a higher molecular weight form of ir β-EP, putative POMC, was increased in CRF KO, but the β-EP peak level was small and similar between the two groups. Vo, void volume; LPH, lipotropic hormone; EP, endorphin. (C) POMC/β-LPH ratio in the AP of WT and CRF KO by Sephadex G-50 gel filtration chromatography. POMC/β-LPH ratio of anterior pituitary from individual mice are shown as the mean ± SEM (n = 4); *P < 0.001 (compared with WT). [Reproduced with permission from Fukuda et al. (Citation2004). Copyright © Elsevier.]](/cms/asset/617506c0-ed87-4c82-b494-a99677356ca4/ists_a_536279_f0012_b.gif)
Figure 13. Effects of CRF on PC-1 mRNA levels. (A) Time-dependent changes in CRF-induced PC-1 mRNA levels. Cells were incubated with the medium containing 100 nM CRF for the times indicated. (B) Effects of a CRF-receptor antagonist on CRF-induced PC-1 mRNA levels. Cells were pre-incubated with the medium containing 1 μM AST or vehicle for 30 min and then incubated for 6 h with the medium containing 100 nM CRF or vehicle; *P < 0.005 (compared with control (0 h or C)). [Reproduced with permission from Kageyama et al. (Citation2009b). Copyright © Elsevier.]
![Figure 13. Effects of CRF on PC-1 mRNA levels. (A) Time-dependent changes in CRF-induced PC-1 mRNA levels. Cells were incubated with the medium containing 100 nM CRF for the times indicated. (B) Effects of a CRF-receptor antagonist on CRF-induced PC-1 mRNA levels. Cells were pre-incubated with the medium containing 1 μM AST or vehicle for 30 min and then incubated for 6 h with the medium containing 100 nM CRF or vehicle; *P < 0.005 (compared with control (0 h or C)). [Reproduced with permission from Kageyama et al. (Citation2009b). Copyright © Elsevier.]](/cms/asset/25c0afb4-3134-4c0b-bd74-b390236a9126/ists_a_536279_f0013_b.gif)
This may have clinical implications, because multiple forms of ir β-EP contents including POMC have been detected in an ectopic ACTH-producing tumor (Suda et al. Citation1982, Citation1986). The synthesis and secretion of both ACTH and β-EP in such an ectopic ACTH-producing tumor may be autonomous, and thus, CRF-independent. In addition, biologically inactive and high-molecular-weight ACTH is produced in some cases of Cushing's disease. Taking into account these findings, multiple forms of ACTH-related peptides including unprocessed ones are present in some ACTH-producing tumors, presumably without the action of PC-1 under the control of CRF.
Summary
In summary, forskolin- or PACAP-stimulated CRF gene transcription is mediated by a CRE in the CRF 5′-promoter region. ICER suppresses the stress response via the inhibition of the cAMP-dependent CRF gene. Both nGRE and SRE are involved in the repression of the CRF gene through glucocorticoids in hypothalamic cells. IL-6 produced in the hypothalamus stimulates the CRF gene, while SOCS-3 is involved in the negative regulation of CRF gene expression. Disruption of the HPA axis may cause a number of diseases related to stress. For example, CRF-induced Pak3 mRNA expression may be related to the proliferation of corticotrophs in Nelson's syndrome. A higher molecular weight form of ir β-EP, putative POMC, is increased in the AP of CRF KO, suggesting the important role of CRF in the processing of POMC through changes in PC-1 expression levels. Multiple forms of ACTH-related peptides including unprocessed ones are present in some cases of Cushing's disease.
Acknowledgements
This work was supported in part by Health and Labour Science Research Grant (Research on Measures for Intractable Diseases) from the Ministry of Health, Labour, and Welfare, Japan.
Declaration of interest: The authors report no conflicts of interest. The authors alone are responsible for the content and writing of the paper.
References
- Agarwal A, Halvorson LM, Legradi G. 2005. Pituitary adenylate cyclase-activating polypeptide (PACAP) mimics neuroendocrine and behavioral manifestations of stress: Evidence for PKA-mediated expression of the corticotropin-releasing hormone (CRH) gene. Brain Res Mol Brain Res. 138:45–57.
- Al-Gahtany M, Horvath E, Kovacs K. 2003. Pituitary hyperplasia. Hormones (Athens). 2:149–158.
- Auernhammer CJ, Bousquet C, Melmed S. 1999. Autoregulation of pituitary corticotroph SOCS-3 expression: Characterization of the murine SOCS-3 promoter. Proc Natl Acad Sci USA. 96:6964–6969.
- Balasenthil S, Sahin AA, Barnes CJ, Wang RA, Pestell RG, Vadlamudi RK, Kumar R. 2004. p21-activated kinase-1 signaling mediates cyclin D1 expression in mammary epithelial and cancer cells. J Biol Chem. 279:1422–1428.
- Castell JV, Gomez-Lechon MJ, David M, Andus T, Geiger T, Trullenque R, Fabra R, Heinrich PC. 1989. Interleukin-6 is the major regulator of acute phase protein synthesis in adult human hepatocytes. FEBS Lett. 242:237–239.
- Cheng YH, Nicholson RC, King B, Chan EC, Fitter JT, Smith R. 2000. Glucocorticoid stimulation of corticotropin-releasing hormone gene expression requires a cyclic adenosine 3′,5′-monophosphate regulatory element in human primary placental cytotrophoblast cells. J Clin Endocrinol Metab. 85:1937–1945.
- Childs GV, Rougeau D, Unabia G. 1995. Corticotropin-releasing hormone and epidermal growth factor: Mitogens for anterior pituitary corticotropes. Endocrinology. 136:1595–1602.
- Foulkes NS, Borrelli E, Sassone-Corsi P. 1991. CREM gene: Use of alternative DNA-binding domains generates multiple antagonists of cAMP-induced transcription. Cell. 64:739–749.
- Fukuda Y, Kageyama K, Nigawara T, Kasagi Y, Suda T. 2004. Effects of corticotropin-releasing hormone (CRH) on the synthesis and secretion of proopiomelanocortin-related peptides in the anterior pituitary: A study using CRH-deficient mice. Neurosci Lett. 367:201–204.
- Ghorbel MT, Sharman G, Leroux M, Barrett T, Donovan DM, Becker KG, Murphy D. 2003. Microarray analysis reveals interleukin-6 as a novel secretory product of the hypothalamo-neurohypophyseal system. J Biol Chem. 278:19280–19285.
- Gillies GE, Linton EA, Lowry PJ. 1982. Corticotropin releasing activity of the new CRF is potentiated several times by vasopressin. Nature. 299:355–357.
- Grinevich V, Fournier A, Pelletier G. 1997. Effects of pituitary adenylate cyclase-activating polypeptide (PACAP) on corticotropin-releasing hormone (CRH) gene expression in the rat hypothalamic paraventricular nucleus. Brain Res. 773:190–196.
- Guardiola-Diaz HM, Kolinske JS, Gates LH, Seasholtz AF. 1996. Negative glucocorticoid regulation of cyclic adenosine 3′,5′-monophosphate-stimulated corticotropin-releasing hormone-reporter expression in AtT-20 cells. Mol Endocrinol. 10:317–329.
- Jacobson L, Sharp FR, Dallman MF. 1990. Induction of fos-like immunoreactivity in hypothalamic corticotropin-releasing factor neurons after adrenalectomy in the rat. Endocrinology. 126:1709–1719.
- Kageyama K, Hanada K, Iwasaki Y, Sakihara S, Nigawara T, Kasckow J, Suda T. 2007. Pituitary adenylate cyclase-activating polypeptide stimulates corticotropin-releasing factor, vasopressin, and interleukin-6 gene transcription in hypothalamic 4B cells. J Encocrinol. 195:199–211.
- Kageyama K, Hanada K, Takayasu S, Iwasaki Y, Sakihara S, Nigawara T, Kasckow J, Suda T. 2008. Involvement of regulatory elements on corticotropin-releasing factor gene promoter in hypothalamic 4B cells. J Endocrinol Invest. 31:1079–1085.
- Kageyama K, Hanada K, Iwasaki Y, Suda T. Regulation and role of suppressor of cytokine signaling-3 in hypothalamic 4B cells. J Endocrinol. 2009a; 201:369–376.
- Kageyama K, Sakihara S, Suda T. Regulation and role of p21-activated kinase 3 by corticotropin-releasing factor in mouse pituitary. Regul Pept. 2009b; 152:88–94.
- Kageyama K, Akimoto K, Suda T. 2010. Corticotropin-releasing factor gene transcription is directly activated after deprivation of glucocorticoids in hypothalamic cells. J Neuroendocrinol. 22:971–978.
- Karagianni N, Tsawdaroglou N. 1994. The c-fos serum response element (SRE) confers negative response to glucocorticoids. Oncogene. 9:2327–2334.
- Karalis K, Muglia LJ, Bae D, Hilderbrand JA. 1997. CRH and the immune system. J Neuroimmunol. 72:131–136.
- King BR, Smith R, Nicholson RC. 2002. Novel glucocorticoid and cAMP interactions on the CRH gene promoter. Mol Cell Endocrinol. 194:19–28.
- Knaus UG, Morris S, Dong HJ, Chernoff J, Bokoch GM. 1995. Regulation of human leukocyte p21-activated kinases through G protein-coupled receptors. Science. 269:221–223.
- Krebs DL, Hilton DJ. 2000. SOCS: Physiological suppressors of cytokine signaling. J Cell Sci. 113:2813–2819.
- Lalli E, Sassone-Corsi P. 1994. Signal transduction and gene regulation: The nuclear response to cAMP. J Biol Chem. 269:17359–17362.
- Legradi G, Holzer D, Kapcala LP, Lechan RM. 1997. Glucocorticoids inhibit stress-induced phosphorylation of CREB in corticotropin-releasing hormone neurons of the hypothalamic paraventricular nucleus. Neuroendocrinology. 66:86–97.
- Ma XM, Aguilera G. 1999. Differential regulation of corticotropin-releasing hormone and vasopressin transcription by glucocorticoids. Endocrinology. 140:5642–5650.
- Malkoski SP, Dorin RI. 1999. Composite glucocorticoid regulation at a functionally defined negative glucocorticoid response element of the human corticotropin-releasing hormone gene. Mol Endocrinol. 13:1629–1644.
- Manser E, Huang HY, Loo TH, Chen XQ, Dong JM, Leung T, Lim L. 1997. Expression of constitutively active alpha-PAK reveals effects of the kinase on actin and focal complexes. Mol Cell Biol. 17:1129–1143.
- McPhie DL, Coopersmith R, Hines-Peralta A, Chen Y, Ivins KJ, Manly SP, Kozlowski MR, Neve KA, Neve RL. 2003. DNA synthesis and neuronal apoptosis caused by familial Alzheimer disease mutants of the amyloid precursor protein are mediated by the p21 activated kinase PAK3. J Neurosci. 23:6914–6927.
- Melzig MF, Papsdorf G, Loose R, Winkler A, Beyermann M, Krause E, Zipper J. 1998. Effects of long-term treatment with corticotropin-releasing factor on corticotropic tumor cells in vitro. Regul Pept. 74:35–40.
- Miyata A, Arimura A, Dahl RR, Minamino N, Uehara A, Jiang L, Culler MD, Coy DH. 1989. Isolation of a novel 38 residue-hypothalamic polypeptide which stimulates adenylate cyclase in pituitary cells. Biochem Biophys Res Commun. 164:567–574.
- Molina CA, Foulkes NS, Lalli E, Sassone-Corsi P. 1993. Inducibility and negative autoregulation of CREM: An alternative promoter directs the expression of ICER, an early response repressor. Cell. 75:875–886.
- Mouri T, Itoi K, Takahashi K, Suda T, Murakami O, Yoshinaga K, Andoh N, Ohtani H, Masuda T, Sasano N. 1993. Colocalization of corticotropin-releasing factor and vasopressin in the paraventricular nucleus of the human hypothalamus. Neuroendocrinology. 57:34–39.
- Muglia LJ, Jenkins NA, Gilbert DJ, Copeland NG, Majzoub JA. 1994. Expression of the mouse corticotropin-releasing hormone gene in vivo and targeted inactivation in embryonic stem cells. J Clin Invest. 93:2066–2072.
- Muglia L, Jacobson L, Dikkes P, Majzoub JA. 1995. Corticotropin-releasing hormone deficiency reveals major fetal but not adult glucocorticoid need. Nature. 373:427–432.
- Muglia LJ, Jacobson L, Weninger SC, Luedke CE, Bae DS, Jeong KH, Majzoub JA. 1997. Impaired diurnal adrenal rhythmicity restored by constant infusion of corticotropin-releasing hormone in corticotropin-releasing hormone-deficient mice. J Clin Invest. 99:2923–2929.
- Muglia LJ, Jacobson L, Luedke C, Vogt SK, Schaefer ML, Dikkes P, Fukuda S, Sakai Y, Suda T, Majzoub JA. 2000. Corticotropin-releasing hormone links pituitary adrenocorticotropin gene expression and release during adrenal insufficiency. J Clin Invest. 105:1269–1277.
- Navarra P, Tsagarakis S, Faria MS, Rees LH, Besser GM, Grossman AB. 1991. Interleukins-1 and -6 stimulate the release of corticotropin-releasing hormone-41 from rat hypothalamus in vitro via the eicosanoid cyclooxygenase pathway. Endocrinology. 128:37–44.
- Nomura M, Ueta Y, Serino R, Kabashima N, Shibuya I, Yamashita H. 1996. PACAP type I receptor gene expression in the paraventricular and supraoptic nuclei of rats. Neuroreport. 8:67–70.
- Ochedalski T, Subburaju S, Wynn PC, Aguilera G. 2007. Interaction between oestrogen and oxytocin on hypothalamic–pituitary–adrenal axis activity. J Neuroendocrinol. 19:189–197.
- Ogura E, Kageyama K, Hanada K, Kasckow J, Suda T. 2008. Effects of estradiol on regulation of corticotropin-releasing factor gene and interleukin-6 production via estrogen receptor type beta in hypothalamic 4B cells. Peptides. 29:456–464.
- Ram PA, Waxman DJ. 1999. SOCS/CIS protein inhibition of growth hormone-stimulated STAT5 signaling by multiple mechanisms. J Biol Chem. 274:35553–35561.
- Schimpl A, Wecker E. 1972. Replacement of T-cell function by a T-cell product. Nat New Biol. 237:15–17.
- Schmitz J, Weissenbach M, Haan S, Heinrich PC, Schaper F. 2000. SOCS3 exerts its inhibitory function on interleukin-6 signal transduction through the SHP2 recruitment site of gp130. J Biol Chem. 275:12848–12856.
- Seasholtz AF, Thompson RC, Douglass JO. 1988. Identification of a cyclic adenosine monophosphate-responsive element in the rat corticotropin-releasing hormone gene. Mol Endocrinol. 2:1311–1319.
- Shioda S, Shuto Y, Somogyvari-Vigh A, Legradi G, Onda H, Coy DH, Nakajo S, Arimura A. 1997. Localization and gene expression of the receptor for pituitary adenylate cyclase-activating polypeptide in the rat brain. Neurosci Res. 28:345–354.
- Spengler D, Rupprecht R, Van LP, Holsboer F. 1992. Identification and characterization of a 3′,5′-cyclic adenosine monophosphate-responsive element in the human corticotropin-releasing hormone gene promoter. Mol Endocrinol. 6:1931–1941.
- Suda T, Tozawa F, Yamaguchi H, Shibasaki T, Demura H, Shizume K. 1982. Multiple forms of immunoreactive ß-endorphin are present in an ectopic adrenocorticotropin-producing tumor but not in normal pituitary or pituitary adenomas. J Clin Endocrinol Metab. 54:167–171.
- Suda T, Yajima F, Tomori N, Demura H, Shizume K. 1985. In vitro study of immunoreactive corticotropin-releasing factor release from the rat hypothalamus. Life Sci. 37:1499–1505.
- Suda T, Kondo M, Totani R, Hashimoto N, Suzuki M, Imaki T, Oba Y, Tomori N, Tozawa F, Sumitomo T, Nakagami Y, Ushiyama T, Demura H, Shizume K. 1986. Ectopic adrenocorticotropin syndrome caused by lung cancer that responded to corticotropin-releasing hormone. J Clin Endocrinol Metab. 63:1047–1051.
- Takaki A, Huang QH, Somogyvari-Vigh A, Arimura A. 1994. Immobilization stress may increase plasma interleukin-6 via central and peripheral catecholamines. Neuroimmunomodulation. 1:335–342.
- Turnbull AV, Rivier CL. 1999. Regulation of the hypothalamic–pituitary–adrenal axis by cytokines: Actions and mechanisms of action. Physiol Rev. 79:1–71.
- Vale W, Spiess J, Rivier C, Rivier J. 1981. Characterization of a 41-residue ovine hypothalamic peptide that stimulates secretion of corticotropin and beta-endorphin. Science. 213:1394–1397.
- Vallieres L, Rivest S. 1999. Interleukin-6 is a needed proinflammatory cytokine in the prolonged neural activity and transcriptional activation of corticotropin-releasing factor during endotoxemia. Endocrinology. 140:3890–3903.
- van der Laan S, Lachize SB, Vreugdenhil E, de Kloet ER, Meijer OC. 2008. Nuclear receptor coregulators differentially modulate induction and glucocorticoid receptor-mediated repression of the corticotropin-releasing hormone gene. Endocrinology. 149:725–732.
- van Wijk PA, van Neck JW, Rijnberk A, Croughs RJ, Mol JA. 1995. Proliferation of the murine corticotropic tumour cell line AtT20 is affected by hypophysiotrophic hormones, growth factors and glucocorticoids. Mol Cell Endocrinol. 111:13–19.
- Venihaki M, Dikkes P, Carrigan A, Karalis KP. 2001. Corticotropin-releasing hormone regulates IL-6 expression during inflammation. J Clin Invest. 108:1159–1166.
- Yamamori E, Asai M, Yoshida M, Takano K, Itoi K, Oiso Y, Iwasaki Y. 2004. Calcium/calmodulin kinase IV pathway is involved in the transcriptional regulation of the corticotropin-releasing hormone gene promoter in neuronal cells. J Mol Endocrinol. 33:639–649.
- Yao M, Denver RJ. 2007. Regulation of vertebrate corticotropin-releasing factor genes. Gen Comp Endocrinol. 153:200–216.
- Zhang S, Han J, Sells MA, Chernoff J, Knaus UG, Ulevitch RJ, Bokoch GM. 1995. Rho family GTPases regulate p38 mitogen-activated protein kinase through the downstream mediator Pak1. J Biol Chem. 270:23934–23936.
- Zhou D, Kusnecov AW, Shurin MR, DePaoli M, Rabin BS. 1993. Exposure to physical and psychological stressors elevates plasma interleukin 6: Relationship to the activation of hypothalamic–pituitary–adrenal axis. Endocrinology. 133:2523–2530.