Abstract
We evaluated the effects of early maternal deprivation (MD; age 7–14 days) alone or in combination with unpredictable chronic stress (UCS; MDUN; 28–84 days) on anxiety and learning in 90 days old adult rats. We hypothesized that exposure to both stressors (MDUN) would be more detrimental than exposure to one or neither. Unexpectedly, adult rats from the MDUN group did not differ from control animals, whereas adult MD animals exhibited impaired avoidance learning. We next investigated the effect of juvenile-onset (30–90 days) versus adult-onset (60–90 days) stress on avoidance learning in adulthood (90 days). We found that adult-onset chronic stress impaired avoidance learning and memory whereas juvenile-onset stress did not. Thus, the results again indicate that juvenile exposure to UCS induces resilience rather than impairment.
Introduction
Deprivation of critical experiences during development may be destructive to the development of higher level abilities. Child maltreatment is considered to have significant negative effects on the development of the child's coping abilities (Mash and Wolfe Citation2005). Neglect during infancy is sometimes characterized by early deprivation followed by reasonable growing conditions, although frequent neglect during infancy is usually followed by unstable and stressful childhood conditions (Perry Citation1997). Nevertheless, it is difficult to establish a causal relationship between a trauma/stress and specific cognitive impairments in humans because of variability in the nature and intensity of maltreatment (Kerr et al. Citation2000).
In rats, maternal deprivation (MD) can lead to long-term cognitive deficits (Benetti et al. Citation2009) and has life-long consequences for behaviour (Lévy et al. Citation2003). Animal research has focused mainly on MD during the first postnatal days. The presence of the dam is crucial during this period, and her absence has been shown to cause wide-ranging effects (Ellenbroek and Cools Citation2000; Oitzl et al. Citation2000; Barna et al. Citation2003). Postnatal maternal separation makes rats more vulnerable to the development of abnormal feeding behaviour (Vázquez et al. Citation2006) and has long-lasting consequences for susceptibility to inflammatory autoimmune diseases (Iwasaki et al. Citation2000). Rats reared without mother and siblings also show consistent deficits in learning involving social interactions (Teunis et al. Citation2002).
MD and other stressors encountered during juvenility affect the coping abilities of rats in adulthood (Tsoory and Richter-Levin Citation2006; Tsoory et al. Citation2007; Toth et al. 2008a,b). These stressors adversely affect exploratory behaviour and avoidance learning in adult rats (Tsoory and Richter-Levin Citation2006; Tsoory et al. Citation2007; although see Toth et al. Citation2008a for a contrasting view). Furthermore, such stressors have sex-dependent effects on explorative and social behaviours and brain morphology (Ferdman et al. Citation2007).
For further understanding of the long-term effects of stress, Experiment A evaluated the effects of early MD (7–14 days postnatal) alone, or in combination with unpredictable chronic stress (UCS; 28–84 days), on anxiety and learning in adult rats (90 days). Experiment B investigated the effect of age at onset of UCS (juvenility of 30 days versus adulthood of 60 days) on avoidance learning in adulthood (90 days).
Rationale
Experiment A
Aim
To determine the effect of exposure to juvenile-onset UCS following exposure to a single infantile stressor on coping in adulthood using a battery of anxiety and learning tests.
Experiment B
Aim
To investigate whether the unexpected results of Experiment A could be explained by the age of the rat when UCS exposure began, Experiment B investigated the effect of juvenile-onset stress versus adult-onset stress on shuttle avoidance learning in adulthood.
Materials and methods
Animals
The experiments used male Sprague–Dawley rat pups, raised by their dams with their siblings in the Haifa University vivarium. At 28 days of age, the pups were separated from their dams and housed 4–5 per cage in 75 × 55 × 18 cm Plexiglas cages on sawdust bedding in temperature-controlled (22–23°C) animal quarters, on a 12:12 light–dark cycle (lights on from 07:00 to 19:00). The animals had ad libitum access to Teklad Global Diet 2018S solid food pellets (Harlan Teklad, Madison, WI) and water except during the food deprivation stressor and during the learning and testing sessions in the complex maze. The mean weight range of all animals at weaning was 87 ± 12 (75–99) g (mean ± SE (range)), and at adulthood 356 ± 6 (350–363) g. The body weight of the experimental (i.e. stress-exposed) and control groups did not differ significantly.
Stress, anxiety and learning protocols
See , for a detailed description of the group protocols.
Stress protocol
(1) | MD
| ||||||||||
(3) | UCS
|
(5) | Elevated platform (EP): Rats were placed individually for 30 min on a black platform of (12 × 12 cm) 70 cm above the floor. This was repeated three times with an inter-trial interval of 20 min in a resting cage. | ||||
(6) | Acute swim stress (ASS): Individual rats were placed for 15 min in a circular water tank (diameter: 50 cm and height: 50 cm). Water depth was 40 cm, with temperature maintained at 23–25°C. Following exposure to the ASS, rats were allowed to dry in a resting cage. | ||||
(7) | Food deprivation: Food was not provided for 23 h except water. |
Anxiety tests
(8) | The open-field test
|
Learning tests
(10) | (1) The two-way avoidance shuttle box |
(11) | Active avoidance testing was conducted in a 60 × 26 × 28 cm two-way sound attenuated shuttle-box divided into two equal compartments by a Plexiglas wall, with an opening to allow passage between the compartments. | ||||
(12) | Each rat received a 5-min familiarization period in the shuttle-box before the first trial began. The trials began with a 10-s tone immediately followed by a 0.8-mA shock delivered through the grid floor for a maximum of 10 s. If the rat moved to the opposite compartment during the 10-s tone, the tone ceased, no foot shock was delivered, and the experimenter recorded the event as an ‘avoidance’ response. Moving to the opposite compartment only after the onset of the shock immediately terminated both the tone and the shock and was scored as an ‘escape’ response. Failure to move to the opposite compartment caused the rat to experience the full 10-s shock and was recorded as a “no-escape” response. | ||||
(13) | Each rat went through a single session of 100 or 75 trials (Experiments A and B, respectively) separated by an inter-trial fixed interval of 1 min. In Experiment B, a memory retention test was carried out 15 days after the learning session. In the retention test, each rat went through a single session of 50 trials, separated by an inter-trial fixed interval of 1 min. Scoring was done by computer. |
(14) | (2) Water maze |
The water maze (Avital et al. Citation2001) was 170 cm in diameter with a black rim of 50 cm high. Water depth was 30 cm and temperature was maintained at 23 ± 1°C. A hidden black platform (12 × 12 cm) submerged 3 cm beneath the surface provided the only means to escape swimming. The maze itself was featureless and the only obvious landmarks were those outside the water maze, in the surrounding environment (Morris Citation1984). Rats were placed in the maze and were allowed to swim freely until they reached the submerged platform or for a maximum of 1 min. At the end of each of the 12 trials, rats were either led to the platform or left on it for 15 s. Each trial began from a different starting point selected at random. The experimenter measured the time it took the rat to mount the platform. All trials were conducted by the same experimenter and on the same day between 8:30 and 16:00 h with an alternating inter-trial rest interval of 1 or 4 min.
(15) | (3) The modified complex maze |
The maze consisted of five rectangular boxes (20 × 30 cm) connected linearly to each other by short corridors. Each box had two doors that could be pushed open only in the forward direction. The doors divided each box into a Y shape. Rats were placed in the start box and which navigated to the target box containing saccharine (0.606%; Lévy et al. Citation2003). The experimenter measured the time the rat required to navigate the maze until it crossed the last door in the fifth (the target) box.
(16) | Shaping phase: Rats were given at least 4 days of shaping (3 trials per day) during which all doors were closed but unlocked, such that they could be pushed open. Rats were trained until they were able to move through the maze from the launching box to the saccharine in ≤ 3 min. | ||||
(17) | Learning phase: Each rat was expected to learn its own randomly set route through the maze. One of the doors in each box was locked; therefore, the rat learned which door could be opened through a process of trial and error. The rat practised its route for 6 consecutive days until it reached the ≤ 3 min speed criterion. | ||||
(18) | Test phase: All the doors were closed but unlocked. The experimenter awarded one point each time the rat passed through the correct door in each pair up to a maximum possible score of five points. |
During learning and testing sessions, the rat was water deprived. The rat was rewarded by a drop of saccharine upon reaching the target box. Upon completion of each session, the rat was allowed to drink for half an hour. Each rat was tested at the same time of day by the same experimenter.
Experiments
In Experiment A, rats were randomly assigned to control, MD and MDUN groups that underwent the combination of stress and testing protocols described in . In Experiment B, rats were randomly assigned to control, juvenile-onset stress and adult-onset stress groups that underwent the combination of stress and testing protocols described in .
Statistical analyses
All analyses were performed using the SPSS 11.5 software package. Results are presented as means ± SE of the means (). For analysis, trials in the two-way shuttle avoidance box were collapsed into blocks of five. In Experiment A, the data were not normally distributed and, therefore, we used non-parametrical analysis (two-tailed Kruskal–Wallis (KW) and Mann–Whitney U, as appropriate). In Experiment B, the data were normally distributed and were analysed by one-way ANOVA. For all tests, a p-value of < 0.05 was considered statistically significant.
Approval
The experiments were approved by the institutional Animal Care and Use Committee and were carried out in accordance with the National Institute of Health Guide for the Care and Use of Laboratory Animals.
Results
Experiment A
Experiment A examined the effect of exposure to juvenile-onset UCS following exposure to a single infantile stressor on coping in adulthood using the open-field test, the shuttle box, the Morris water maze and the modified complex maze.
The open-field test
(19) | Significant differences (KW = 6.22, p = 0.001) were found between the total activity levels of the groups (crossings in the periphery plus in the centre of the open field). Further analysis using the Mann–Whitney test revealed a significant difference between the MD and MDUN groups (U = 57.50, p = 0.001). The MDUN group showed a higher level of total activity ( |
Learning tests
The two-way shuttle avoidance task
(20) | Avoidance responses: Significant differences were found between the groups in the avoidance response (KW = 5.90, p = 0.001). The control group avoided the shock significantly more frequently than the MD group (U = 72.50, p = 0.001). No significant differences were found between the MD and MDUN groups and between the control and MDUN groups (). | ||||
(21) | Escape responses: There were no significant between-group differences in escape responses (data not shown). | ||||
(22) | No-escape responses: There were significant differences between the groups (KW = 17.49, p = 0.001). The control group performed significantly better (i.e. showed fewer no-escape responses) than the MD group (U = 43.00, p = 0.001). In addition, a significant difference was found between the MDUN and MD groups (U = 16.50, p = 0.001), with the MDUN group exhibiting fewer no-escape responses. No significant differences were found between the control and MDUN groups (). |
Figure 2. Activity in the open field (Experiment A). (A) Number of times the rats crossed grid lines on the floor of the open field. A larger number indicates less anxiety. (B) Ratio of central to peripheral line crossings in the open field. A higher ratio indicates less anxiety. Three groups of adult rats were tested: control, maternal deprivation (MD) and maternal deprivation followed by juvenile-onset uncontrollable chronic stress (MDUN). ** 0.01, *** 0.001.
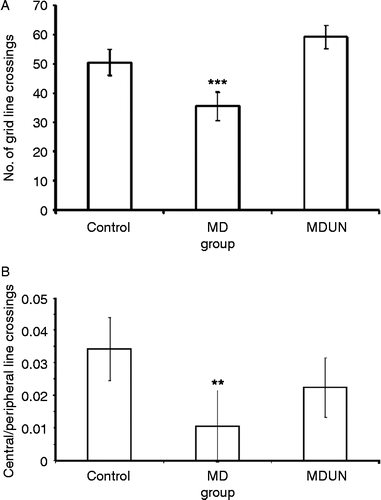
Figure 3. The number of (A) avoidance responses and (B) no-escape responses in the shuttle box to the conditioned stimulus (tone) that immediately preceded a 10-s 0.8-mA foot shock during 20 blocks of five trials in the two-way shuttle avoidance box in Experiment A. Three groups of adult rats were tested: control, maternal deprivation (MD) and maternal deprivation followed by juvenile-onset uncontrollable chronic stress (MDUN). In figure (A), asterisks (***) indicate that the MD group differed from the control group, p = 0.001. In figure (B), asterisks (***) indicate that the MD group differed from the MDUN and hashes (###) indicate the control groups, p = 0.001.
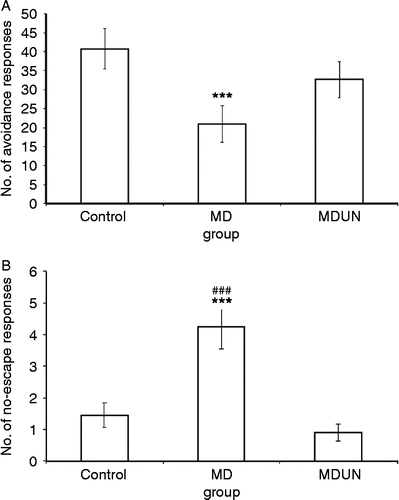
Water maze. There were no statistically significant differences in performance in the water maze. However, as seen in , the control and MDUN groups exhibited a smooth reduction in escape latency to the submerged platform with repeated exposure to the maze, whereas the MD group produced a different, fluctuating learning curve. This led to a significant difference in escape latencies in the last trial. Mean percentages were found to differ significantly (KW = 20.58, p = 0.001). Further analysis using the Mann–Whitney test showed that the control and MNUN groups performed significantly better than the MD group in finding the submerged platform (U = 19.50, p = 0.001 and U = 10.50, p = 0.001, for control vs. MD group, and MDUN vs. MD group, respectively). This suggests that, in contrast to the other groups, the MD group did not develop an effective spatial map for the location of the platform.
Figure 4. Learning in the water maze (Experiment A). The time (s) required for the control, maternal deprivation (MD) and maternal deprivation followed by juvenile-onset uncontrollable chronic stress (MDUN) groups to find a hidden platform submerged at a fixed location. Data for each of the 12 trials (T1–T12) are given.
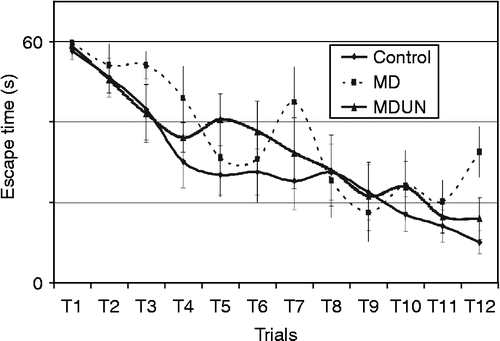
The modified complex maze. There were significant differences between the groups (KW = 8.73, p = 0.001). The control group performed significantly better than the MD group (U = 61.00, p = 0.001) in remembering the correct route through the doors of the maze to the saccharine target. In addition, a significant difference was found between the control group and MDUN group (U = 136.00, p = 0.001), with the control group being more successful. No significant differences were found between the MD and MDUN groups ().
Figure 5. Memory of a learned route through the modified complex maze (Experiment A). Mean percentage of correct door openings (traverses) by the control, MD and MD followed by juvenile-onset uncontrollable chronic stress (MDUN) groups in the modified complex maze. Data for the five trials conducted during the test phase of the experiment are given. Asterisks (**) indicate that the MD and MDUN groups differ from the control group, p < 0.01.
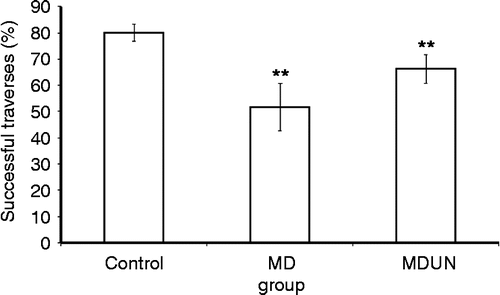
Experiment B
Experiment B examined whether exposure to chronic unpredictable stress results in different outcomes for adult rats depending on their age at onset of exposure (juvenility compared with adulthood). The control, juvenile-onset stress and adult-onset stress groups were tested in adulthood in the two-way shuttle avoidance task used in Experiment A.
One-way ANOVA showed between-group differences in the number of avoidance responses [F(2,27) = 3.842, p < 0.05]. The adult-onset stress group made fewer avoidance responses than the control (p < 0.05) and juvenile-onset stress (p < 0.05) groups ().
Figure 6. Number of avoidance and no-escape responses during training in the shuttle box (Experiment B). Rats that were exposed to stress during adulthood (the adult-onset stress group) and then trained in a two-way shuttle avoidance task made (A) fewer avoidance responses and (B) more no-escape responses during training than rats whose exposure to the same stress protocol began during juvenility (the juvenile-onset stress group) and control animals. The learning phase involved 75 sessions in the apparatus (analysed as 15 blocks of five trials). A group effect was observed both for the number of avoidance and the number of no-escape responses, with the adult-onset stress group making fewer avoidance responses than the control (p < 0.01) and juvenile-onset stress (p < 0.05) groups.
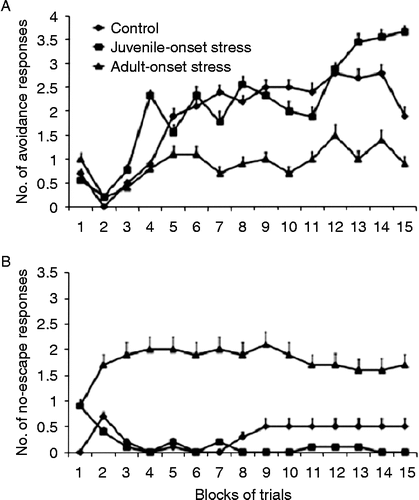
One-way ANOVA indicated that the number of no-escape responses differed between the groups [F(2,27) = 3.925, p < 0.05], with animals from the adult-onset stress group making a higher number of no-escape responses than the control (p < 0.05) and juvenile-onset stress animals (p < 0.05) (). The number of no-escape responses by the juvenile-onset stress and control groups did not differ significantly. No significant differences were found between groups in the number of escape responses.
In the memory retention test 15 days later, there was a group effect both for the number of avoidance [F(2,27) = 4.415, p < 0.05] and no-escape responses [F(2,27) = 5.055, p < 0.05]. As in the training session, the adult-onset stress group made fewer avoidance responses than the control (p < 0.01) and juvenile-onset stress (p < 0.05) groups () and a significantly higher number of no-escape responses than the control (p < 0.05) and juvenile-onset stress (p < 0.01) groups (). The number of no-escape responses by the juvenile-onset stress group and control group did not differ significantly. No significant differences were found between groups in the number of escape responses.
Figure 7. Number of avoidance and no-escape responses during memory testing in the shuttle box (Experiment B). Fifteen days after the learning session, the groups underwent a memory retention test consisting of 10 blocks of five trials in the two-way shuttle avoidance task. The memory retention test revealed that (A) the number of avoidance responses did not differ significantly between the juvenile-onset and adult-onset stress groups. However, (B) there were significant differences between the number of no-escape responses made by the groups. The adult-onset stress group made more no-escape responses than the control (p < 0.05) and juvenile-onset stress groups (p < 0.05). The number of no-escape responses by the juvenile-onset stress and control groups did not differ significantly.
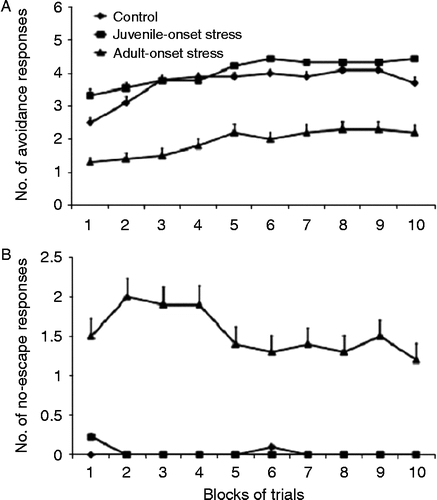
Discussion
UCS has been shown to have significant effects on a range of coping abilities. A growing body of evidence suggests that exposure to UCS affects the developing brain by altering normal neurodevelopmental processes (Lauder Citation1988; McAllister et al. Citation1999; Avital et al. Citation2006).
Animal models of stress-induced depression have identified a bimodal reactivity to stress, namely ‘resilience’ and ‘vulnerability’. Early exposure to consistent moderate stress can result in resilience, whereas exposure to unpredictable or chronic stress can result in functional deficits and vulnerability to future stressors (Plotsky and Meaney Citation1993; Vaid et al. Citation1997; Liu et al. Citation2000).
The most surprising finding in the current study is the absence of vulnerability in rats exposed to our juvenile-onset UCS protocol in addition to MD during infancy. Experiment A investigated the ‘costs’, in terms of anxiety levels and cognitive abilities, of MD alone or followed by prolonged exposure to UCS (MDUN). Our hypothesis, based on our earlier findings (Avital and Richter-Levin Citation2005) and those of others (Shors et al. Citation1990; Roceri et al. Citation2002; Karst and Joels Citation2003), was that there would be a linear relationship between the degree of stress in the juvenile environment (MDUN>MD>control) and the intensity of the adult rats' vulnerability to stress and cognitive impairment. Contrary to these expectations, we found no significant differences between the groups in their overall anxiety levels. Furthermore, the MDUN group consistently outperformed the MD group and often performed as well as the control group in the learning tests (which were in themselves stressful, and for the shuttle avoidance and water maze tests, also aversive). This result was particularly surprising in light of reports that UCS exposure impairs coping behaviour in adult animals (Yann et al. Citation2006).
We, therefore, undertook Experiment B to investigate whether the age at onset of UCS exposure (juvenile versus adult) was a factor in these results, as this appeared to be one of the important differences between the current study and most other UCS studies. Testing of the animals at the same adult age (90 days) necessitated that the two groups experience stress exposures of different durations. Previous studies have reported that stress exposure of more than 21 days in adulthood impairs learning (e.g. Luine et al. Citation1994). Therefore, we used a long exposure paradigm of at least 30 days of stress that could be expected to result in impairment for both experimental groups, and compared the effects of stress as a function of age at onset.
We found that juvenile-onset stress, in contrast to adult-onset stress, does not adversely affect performance in the shuttle-box learning test, despite the juvenile-onset stress group experiencing stress for twice the duration of the adult-onset stress group (60 days vs. 30 days, respectively). The results of Experiment B confirm previous findings (Toth et al. Citation2008a) that exposure to UCS in adulthood leads to significant deficits in learning and memory in the two-way shuttle avoidance task. They also indicate that juvenile-onset exposure to the UCS stress protocol has no effect on learning or retention of the shuttle-avoidance task in adulthood, in line with our Experiment A results. This is significant because it suggests that the juvenile-onset stress group became accustomed to chronic stress, whereas for the adult-onset stress group, the UCS paradigm contrasted sharply with previous life experience. These findings strengthen the argument that early exposure to long-term but mildly stressful experiences may promote the ability to successfully cope with and solve aversive situations in adulthood (Ossowska et al. Citation2004). Our findings also support the recent match–mismatch hypothesis (Raftogianni et al. Citation2010), which proposes an increased coping ability if early and later life experiences match.
Taken together, Experiments A and B show that exposing rats to UCS results in long-lasting alterations in learning, memory and behaviour. However, the main effect of chronic stress throughout juvenility is a reduction in the vulnerability of this group when faced with stressful learning in adulthood. Moreover, it appears that UCS starting in juvenility protects against the vulnerability induced by MD and its consequent cognitive cost in adulthood. Oomen et al. (Citation2010) suggest that adverse experiences during development promote resilience in an adverse or stressful adult environment. Similarly, our results indicate that juvenile-onset exposure to UCS results in the development of resilience to some of the effects of early life stress, with this resilience persisting into adulthood.
We note that, to achieve conditions similar to those of childhood neglect, the UCS procedure used here emphasized unpredictability rather than severity. Consequently, we developed a paradigm in which the stressors were not presented every day or in a predictable order. We have previously demonstrated that exposure to a brief stressor during juvenility has long-term detrimental effects on coping abilities in adulthood (Yann et al. Citation2006). Moreover, Jacobson-Pick and Richter-Levin (Citation2010) recently demonstrated that exposure to current stressors in juvenility causes elevation in CORT levels similar to the elevation observed following exposure to the same stressors in adulthood. Thus, the UCS stressors used (platform, forced swim and food deprivation), although mild compared with those adopted in other chronic stress protocols (e.g. Bondi et al. Citation2007) and repeated (which may have further attenuated their effects over time; Broadhurst Citation1975), are indeed stressful to both age groups. However, the behavioural responses differ with age. Those results, together with the results presented here, suggest that short-term stress at an early age has different effects from chronic deprivation and uncertainty over a long period, with the former apparently inducing vulnerability, whereas the latter induces resilience.
Overall, the current results suggest that, unlike the accepted view (Huang et al. Citation2002), a period of UCS during juvenility does not impair the ability to improve coping, but instead affects the type of coping strategies adopted. It is suggested that rats that experience chronic stress from juvenility develop coping abilities, but these differ from those developed by animals growing under more comfortable conditions.
Limitations
One must take care not to be too literal in interpreting animal models. They are not to be taken to comprehensively reflect the human disorder (childhood neglect), but rather to approximate certain aspects of it.
There were several differences between the procedures used in Experiments A and B that stemmed from Experiment B having been planned only after Experiment A yielded unexpected results. Thus, in the current study, Experiment A did not include a group of animals exposed only to UCS, as in Experiment B. Such a group would have been advantageous for a full comparison. Also, the two experiments did not use identical post-stress tests: Experiment B focused on a single learning test out of the battery of three anxiety and learning tests used in Experiment A. As mentioned, this is because Experiment A's results had indicated that this particular test (the shuttle box) provided sufficient information regarding the impact of the stressors.
It should also be noted that, in Experiment B, the groups differed not only with respect to age at stress onset (juvenile vs. adult) but also with respect to the duration of stress exposure (60 days vs. 30 days, respectively). Further studies are required to illuminate the relative contributions of age-at-onset and duration of exposure to the observed differences in post-stress learning in adulthood. In addition, the shuttle-box data from Experiments A and B exhibited different distributions (probably because of the differences between the two independent studies outlined above) and consequently they had to be analysed differently.
The findings suggest that the effects of UCS during juvenility may differ qualitatively from a similar experience in adulthood, with the former leading to cognitive resilience, whereas the latter induces vulnerability and impaired cognitive functioning in adulthood. Whether more severe chronic stress conditions during juvenility would lead to impairments, and identification of the critical variables that determine whether impairment or resilience will develop, remains to be tested.
Declaration of interest: The authors report no conflicts of interest. The authors alone are responsible for the content and writing of the paper.
References
- Avital A, Richter-Levin G. 2005. Exposure to juvenile stress exacerbates the behavioral consequences of exposure to stress in the adult rat. Int J Neuropsychopharmacol. 8 2: 163–173.
- Avital A, Richter-Levin G, Leschiner S, Spanier I, Veenman L, Weizman A, Gavish M. 2001. Acute and repeated swim stress effects on peripheral benzodiazepine receptors in the rat hippocampus, adrenal, and kidney. Neuropsychopharmacology. 25 5: 669–678.
- Avital A, Ram E, Maayan R, Weizman A, Richter-Levin G. 2006. Effects of early-life stress on behavior and neurosteroid levels in the rat hypothalamus and entorhinal cortex. Brain Res Bull. 68 6: 419–424.
- Barna I, Bálint E, Baranyi J, Bakos N, Makara GB, Haller J. 2003. Gender-specific effect of maternal deprivation on anxiety and corticotropin-releasing hormone mRNA expression in rats. Brain Res Bull. 15:85–91.
- Benetti F, Mello PB, Bonini JS, Monteiro S, Cammarota M, Izquierdo I. 2009. Early postnatal maternal deprivation in rats induces memory deficits in adult life that can be reversed by donepezil and galantamine. Int J Dev Neurosci. 27 1: 59–64.
- Bondi CO, Rodriguez G, Gould GG, Frazer A, Morilak DA. 2007. Chronic unpredictable stress induces a cognitive deficit and anxiety-like behavior in rats that is prevented by chronic antidepressant drug treatment. Neuropsychopharmacology. 33 2: 320–331.
- Broadhurst PL. 1975. The Maudsley reactive and nonreactive strains of rats: A survey. Behav Gen. 5 4: 299–319.
- Ellenbroek BA, Cools AR. 2000. The long-term effects of maternal deprivation depend on the genetic background. Neuropsychopharmacology. 23:99–106.
- Ferdman N, Murmu RP, Bock J, Braun K, Leshem M. 2007. Weaning age, social isolation, and gender, interact to determine adult explorative and social behavior and dendritic spine morphology in prefrontal cortex of rats. Behav Brain Res. 180:174–182.
- Huang LT, Holmes GL, Lai MC, Hung PL, Wang CL, Wang TJ, Yang CH, Liou CW, Yang SN. 2002. Maternal deprivation stress exacerbates cognitive deficits in immature rats with recurrent seizures. Epilepsia. 43 10: 1141–1148.
- Iwasaki S, Inoue K, Kiriike N, Hikiji K. 2000. Effect of maternal separation on feeding behavior of rats in later life. Phys Behav. 70:551–556.
- Jacobson-Pick S, Richter-Levin G. 2010. Differential impact of juvenile stress and corticosterone in juvenility and in adulthood in male and female rats. Behav Brain Res. 214:268–276.
- Karst H, Joels M. 2003. Effect of chronic stress on synaptic currents in rat hippocampal dentate gyrus neurons. J Neurophysiol. 89 1: 625–633.
- Kerr MA, Black MM, Krishnakumar A. 2000. Failure to thrive, maltreatment and the behaviour and development of 6-year-old children from low income, urban families: A cumulative risk model. Child Abuse Negl. 24 5: 587–598.
- Lauder JM. 1988. Neurotransmitters as morphogens. Prog Brain Res. 73:365–387.
- Lévy F, Melo AI, Galef BGJr, Madden M, Fleming AS. 2003. Complete maternal deprivation affects social, but not spatial, learning in adult rats. Dev Psychobiol. 43 3: 177–191.
- Liu D, Caldji C, Sharma S, Plotsky PM, Meaney MJ. 2000. Influence of neonatal rearing conditions on stress-induced adrenocorticotropin responses and norepinepherine release in the hypothalamic paraventricular nucleus. J Neuroendocrinol. 12 1: 5–12.
- Luine V, Villegas M, Martinez C, McEwen BS. 1994. Repeated stress causes reversible impairments of spatial memory performance. Brain Res. 639 1: 167–170.
- Mash EJ, Wolfe DA. 2005. Abnormal child psychology. Belmont, CA: Thomson Learning, Wadsworth Publishing.
- McAllister TW, Saykin AJ, Flashman LA, Sparling MB, Johnson SC, Guerin SJ, Mamourian AC, Weaver JB, Yanofsky N. 1999. Brain activation during working memory one month after mild traumatic brain injury: A functional MRI study. Neurology. 53 6: 1300–1308.
- Morris RG. 1984. Development of water maze procedure for studying spatial learning in the rat. J Neurosci Meth. 11:47–60.
- Oitzl MS, Workel JO, Fluttert M, Frösch F, De Kloet ER. 2000. Maternal deprivation affects behaviour from youth to senescence: Amplification of individual differences in spatial learning and memory in senescent Brown Norway rats. Eur J Neurosci. 12 10: 3771–3780.
- Oomen CA, Soeters H, Audureau N, Vermunt L, van Hasselt FN, Manders EM, Joëls M, Lucassen PJ, Krugers H. 2010. Severe early life stress hampers spatial learning and neurogenesis, but improves hippocampal synaptic plasticity and emotional learning under high-stress conditions in adulthood. J Neurosci. 30 19: 6635–6645.
- Ossowska G, Danilczuk Z, Klenk-Majewska B, Czajkowski L, Zebrowska-Łupina I. 2004. Antidepressants in chronic unpredictable mild stress (cums)-induced deficit of fighting behavior. Pol J Pharmacol. 56 3: 305–311.
- Perry BD. 1997. Incubated in terror: Neurodevelopmental factors in the ‘cycle of violence’. In: Osofsky J. editors. Children, youth and violence: The search for solutions. New York: Guilford Press124–148.
- Plotsky PM, Meaney MJ. 1993. Early, postnatal experience alters hypothalamic corticotropin-releasing factor (CRF) mRNA, median eminence CRF content and stress-induced release in adult rats. Brain Res Mol Brain Res. 18 3: 195–200.
- Raftogianni A, Diamantopoulou A, Miltiadous P, Stamatakis A, Stylianopoulou F. 2010. Neonatal and adolescent experiences interact in determining adult stress coping behaviour. FENS Abstr. 5:113.25.
- Roceri M, Hendriks W, Racagni G, Ellenbroek BA, Riva MA. 2002. Early maternal deprivation reduces the expression of BDNF and N MDA receptor subunits in rat hippocampus. Mol Psychiatry. 7:609–616.
- Shors TJ, Foy MR, Levine S, Thompson RF. 1990. Unpredictable and uncontrollable stress impairs neuronal plasticity in the rat hippocampus. Brain Res Bull. 24 5: 663–667.
- Teunis MA, Heijnen CJ, Sluyter F, Bakker JM, Van Dam AM, Hof M, Cools AR, Kavelaars A. 2002. Maternal deprivation of rat pups increases clinical symptoms of experimental autoimmune encephalomyelitis at adult age. J Neuroimmunol. 133 2: 30–38.
- Toth E, Avital A, Leshem M, Richter-Levin G, Braun K. Neonatal and juvenile stress induces changes in adult social behavior without affecting cognitive function. Behav Brain Res. 2008a; 190:135–139.
- Toth E, Gersner R, Wilf-Yarkoni A, Raizel H, Dar DE, Richter-Levin G, Levit O, Zangen A. Age-dependent effects of chronic stress on brain plasticity and depressive behavior. J Neurochem. 2008b; 107 2: 522–532.
- Tsoory M, Richter-Levin G. 2006. Learning under stress in the adult rat is differentially affected by ‘juvenile’ or ‘adolescent’ stress. Int J Neuropsychopharmacol. 9 6: 713–728.
- Tsoory M, Cohen H, Richter-Levin G. 2007. Juvenile stress induces a predisposition to either anxiety or depressive-like symptoms following stress in adulthood. Eur Neuropsychopharmacol. 17:245–256.
- Vaid RR, Yee BK, Shalev U, Rawlins JN, Weiner I, Feldon J, Totterdell S. 1997. Neonatal nonhandling and in utero prenatal stress reduce the density of NADPH-diaphorase-reactive neurons in the fascia dentata and Ammon's horn of rats. J Neurosci. 17 14: 5599–5609.
- Vázquez DM, Bailey C, Dent GW, Okimoto DK, Steffek A, López JF, Levine S. 2006. Brain corticotropin releasing hormone circuits in the developing rat: Effect of maternal deprivation. Brain Res. 1121:83–94.
- Yann SM, Belzung C, Wim EC. 2006. Effects of unpredictable chronic mild stress on anxiety and depression like behavior in mice. Behav Brain Res. 175:43–50.