Abstract
This study investigated glucocorticoid (GC) responses to season and changes in enclosure size and human proximity in the Persian onager (Equus hemionus onager). Enzyme immunoassays were validated to measure GC metabolites in urine and feces (fGCM). Fecal samples were collected from 10 female onagers while in a large pasture, after transport to smaller yards (in greater proximity to people), and 2 months thereafter. Urine samples were collected for 1 year while females were in smaller yards to examine seasonal GC activity. Approximately, 2-fold increases (P < 0.05) were observed in fGCM levels after transport from pasture to yards with increased human exposure, followed by a rapid decline (within ∼17 days) to baseline (pasture) values. However, responses varied among onagers during the 30 days after translocation, with one female failing to acclimate. Mean fGCM concentrations in smaller yards 2 months after transport were comparable to those in pasture. Seasonal GC concentrations were lowest (P < 0.05) during winter, indicating modest seasonal variability. Results demonstrate an acute increase in GC secretion in Persian onagers that moved from large to small enclosures coincident with increased human activities. Most animals acclimated within 3 weeks, suggesting that this rare equid has retained mechanisms to acclimate to marked alterations in an ex situ environment.
Introduction
Stress responses and elevated glucocorticoid (GC) secretion are regulated by the hypothalamic–pituitary–adrenal (HPA) axis in response to a perceived threat or novel stimulus (Hennessy and Levine Citation1979). During acute stress, GC release activates metabolic resources as a component of an adaptive response (Sapolsky et al. Citation2000). However, prolonged or intermittent exposure to stressors can provoke chronically elevated GC secretion and contribute to abnormal physiology, including immune suppression and weight loss (Cassinello and Pieters Citation2000), tissue atrophy (Munck et al. Citation1984), stereotypies (McBride and Cuddelford Citation2001), decreased reproductive success (Liptrap Citation1993; Dobson and Smith Citation1995), and shorter life span (Carlstead et al. 1999). There is surprisingly little information on adrenocortical activity of wildlife species managed ex situ. Ability to maintain these populations requires an environment that promotes health, reproduction, and normative social behaviors. Many ungulates exist in nature in groups (herds) that range over large territories. Thus, it is important to understand the impact of the ex situ environment, including the influence of restricted space and increased exposure to human activities, on biological function.
There is evidence that enclosure size and animal density affect GC responses as well as reproductive capacity and survivability. Cassinello and Pieters (Citation2000) found that dama gazelles (Gazella dama mhorr) maintained in multi-male groups exhibit more aggressive behaviors, injuries, and stereotypies in small compared to large spaces. Similarly, Père David's deer (Elaphurus davidianus) held in large enclosures (200 ha) with low animal density (0.7 deer/ha) also display less aggression and lower GC production than their counterparts in small (0.75 ha) pens with higher density (25.3 deer/ha; Li et al. Citation2007). The latter authors concluded that small living space was likely imposing physiological stress, expressed as conflict behavior, which may affect reproduction and survival (Li et al. Citation2007). Human proximity also influences GC output in ungulates. For example, increased human activity results in higher GC concentrations in elk (Cervus elaphus; Creel et al. Citation2002) and red deer (Huber et al. Citation2003). In the black rhinoceros (Diceros bicornis), the percentage of enclosure periphery exposed to the public has been associated with poorer reproductive success and increased mortality (Carlstead et al. Citation1999).
Wild equids have benefited from intensive ex situ management programs. A classic example is the Przewalski's horse (Equus ferus przewalskii), once declared extinct in the wild (Moehlman Citation2002). However, systematic breeding and husbandry research have assisted species recovery to allow reintroductions into Mongolia and, more recently, China (Moehlman Citation2002). This success is an incentive for creating self-sustaining collections of other wild equids, including the endangered Persian onager (Equus hemionus onager; CitationIUCN 2010). A subspecies of Asiatic wild ass, the onager's historically vast habitat, once extended from Saudi Arabia to Mongolia and China (Feh et al. Citation2002), but is now markedly reduced to only two protected areas in Iran with approximately 600 remaining individuals (Feh et al. Citation2002). There is a lack of fundamental biological knowledge about this animal, although one study in Mongolia found that individuals traveled 15–40 km per day (Kaczensky et al. Citation2007), suggesting that available space (i.e. enclosure size) may be important for maintaining a healthy population.
There are ∼100 onagers maintained in North American and European zoological institutions (Pohle Citation2008; Volz Citation2009). This population is not self-sustaining owing to it being scattered among holding institutions, and there are too few animals in breeding situations (Feh et al. 2002). Increasing animal production will require recruiting more ex situ space, including from smaller-sized zoos. Thus, a priority is to determine the influence of space and human proximity on adrenocortical activity and ability to acclimate to a new environment, especially for this species that normally ranges over vast territories.
Our approach was to first determine the feasibility of measuring GC metabolites excreted in the urine and feces of this equid. These non-invasive technologies have been adapted to a wide range of species (Möstl et al. Citation1999; Brown et al. Citation2002; Monfort Citation2002; Palme et al. Citation2005; Touma and Palme Citation2005). After determining assay validity, our specific objective was to test the hypothesis that moving Persian onagers from a large pasture to smaller enclosures with increased human proximity (more typical of spaces allotted in urban zoos) would result in sustained increases in fecal GC metabolite (fGCM) levels. Because our experimental design was conducted over time, it was also possible to examine the hypothesis that GC metabolite excretion does not vary seasonally in this species.
Methods
Study area, animals, husbandry, and diet
This study was conducted from March 2007 through June 2009 at the Wilds, a ∼4000 hectare conservation and public visitation facility near Cumberland, OH, USA (39°49′29″ N, 81°44′58″ W). Ten female Persian onagers (age, 1–24 years; mean ± SEM, 8.1 ± 2.6 years) were managed in fenced enclosures designated as a large “pasture” (∼48.6 ha) of rolling grasslands with lakes and shrubbery or smaller “yards” (∼0.4 ha each) with seasonally available grass. Initially, all females were maintained on the large pasture. To accommodate population breeding recommendations and exhibit requirements for the public, this study was conducted over 2 years, with five onagers monitored in the first year (27 March 2007 through 30 June 2008) and five in the second (1 April 2008 through 30 June 2009).
While in the large pasture, onagers were maintained as a single sex herd in the presence of other species, including female Bactrian camels (Camelus bactrianus; n = 3), Père David's deer (E. davidianus; n = 20), and Indian rhinoceroses (Rhinoceros unicornis; n = 5). Onagers had access to water and pasture forage ad libitum. Pasture fecal samples were collected during cooler months (November–March) when grasses were dormant, and each onager was supplemented with grass hay (∼4 kg/animal/day) and herbivore pellets (0.45 kg/animal/day; Mazuri 5Z23, St Louis, MO, USA). Urine and fecal samples (see below) were collected from onagers on pasture during the time of year when the facility was closed to the public (November–March). Thus, encounters with humans were generally limited to twice daily (morning and evening), and brief feeding bouts were conducted by animal caregivers who quickly distributed feed from a vehicle.
The smaller yards were adjacent to the veterinary hospital and intensive animal management compound, providing frequent exposure to humans. Here, onagers were maintained as a single species/sex group and supplemented with grass hay (∼4 kg/animal/day) and herbivore pellets (0.45 kg/animal/day). Females were also subjected to other human activities including landscaping, public tours, movement of other species through the area for veterinary management purposes, and urine/fecal sample collection. During the 15 months in smaller yards, onagers were maintained on a seasonally grass-covered yard for ∼22 h per day and then, using part of the daily grain ration as a reward, encouraged to enter an adjacent “dry lot” for ∼2 h to facilitate urine and feces collection. Females were habituated to urinate with grain reward as positive reinforcement. Individual onagers were monitored closely to ensure proper identification of animal source for each urine and fecal sample. The animal care and use committees of the Wilds and the Smithsonian Conservation Biology Institute approved experimental procedures.
Sample collection and laboratory assay validation
Urine collection and GC determination
Urine samples were collected between 09:00 and 11:00 h once daily (5–7 days per week) from the ground immediately after the onager voided using a clean 10 ml plastic syringe. A 5 ml urine aliquot was centrifuged (1500g, 15 min, 22°C) to remove debris, and the supernatant was decanted and stored ( − 20°C) in labeled 12 × 75 mm polypropylene tubes until analysis. Before evaluation, urine was diluted in phosphate-buffered saline (1:50). Immunoreactive cortisol was quantified by enzyme immunoassay (EIA) using an anti-cortisol antiserum (R4866; 1:85,000 dilution) provided by Coralie Munro (University of California, Davis, CA, USA; Munro et al. Citation1991). Serial dilutions of pooled urine from female onagers displayed parallelism with the standard curve (t = 2.14, P = 0.79). Percent recovery averaged 97.5% in diluted urine spiked with 2.50 and 250.0 pg/well standard. Intra- and inter-assay variations were 3.8 and 11.3%, respectively. To correct for water content in urine samples, cortisol was indexed by creatinine (Cr) concentration and expressed as ng mg− 1 Cr (Gronwall and Price Citation1985; Carrieri et al. Citation2001).
Feces collection and FGCM determination
For each sample, four to five freshly voided fecal pellets were collected between 09:00 and 11:00 h from the ground and were placed into a plastic bag, sealed, and homogenized by manually mixing the bag contents. Each bag was labeled with date and animal number and then stored at − 20°C until analyzed. The latter involved freeze-drying while in the original collection bag over a 4-day period using a commercial lyophilizer (VirTis 25EL, SP Scientific; Warminster, PA, USA). For each sample, 0.2 g of lyophilized feces was weighed and used for hormone extraction (as previously described; Merl et al. Citation2000). Briefly, 1 ml of distilled water was added to dried feces and vortexed followed by adding 4 ml of 100% methanol. Samples were shaken vigorously for 30 min and centrifuged (1500g, 15 min). Then 1 ml of supernatant was transferred to a new labeled tube. Five milliliters of diethyl ether and 250 μl of 5% sodium bicarbonate were added to the tube, vortexed for 30 s, centrifuged (1500g, 15 min), and then placed in a freezer at − 80°C for 30 min, allowing the bottom aqueous layer to freeze. The supernatant was poured into a new labeled tube and evaporated under a stream of air. Samples were reconstituted by adding 500 μl of assay buffer into each dry tube and vortexing vigorously for 30 s, followed by storage at − 20°C for not more than 2 months before analysis. Reconstituted samples were further diluted to 1:10 in assay buffer before being assayed.
A group − specific 11-oxoetiocholanolone EIA (Palme and Möstl Citation1997), which measures 11,17-dioxoandrostanes (11,17-DOA), was used to determine fGCM. The latter term will be used hereafter in the manuscript. This EIA has been successfully validated and applied to measuring fGCM in the domestic horse (Möstl et al. Citation1999; Merl et al. Citation2000; Gorgasser et al. Citation2007). Mean fGCM concentrations were expressed as ng g− 1 dry weight (DW). Serially diluted fecal extracts from female onagers displayed parallelism with the standard curve (t crit = − 0.43, P = 0.67). Percent recovery averaged 96.2% in onager's fecal extracts spiked with 12.5, 50, and 100 pg/well of standard. Intra- and inter-assay variations were 4.9 and 10.5%, respectively.
Biological assay validation: Influence of acute animal manipulation on GC excretion in urine versus feces
Matched urine and fecal samples were collected daily from three female onagers periodically exposed to brief physical restraint, thus provoking a significant change in the animal's daily routine. During this 25-day period, each female was manipulated three to five times with at least 48 h between episodes. Specifically, between 09:30 and 11:00 h, each onager was guided from a small yard through a chute system into a padded, hydraulic restraint device (Fauna Research Inc., Red Hook, NY, USA). The walls of the device were closed, and the animal elevated about 15 cm before undergoing transrectal palpation of the reproductive tract, including insertion of a standard 5 MHz linear ultrasound probe (Aloka, Wallingford, CT, USA) to examine the uterus and ovaries. This procedure (from entering the chute to the end of ultrasonography) lasted for about 6 min (range, 3–10 min). Urine and fecal samples were collected between 09:00 and 11:00 h on days in which physical restraint did not occur. On days with restraint, urine and feces were taken after the procedure (between 11:00 and 14:00 h). Samples were stored and analyzed for GC as described above.
depicts the ability of both assays to identify changes in adrenocortical activity reflected in urine and fecal samples caused by the 3–10 min restraint combined with rectal palpation and ultrasonography. Both types of assay revealed a response to the manipulations as reflected by an approximate 2-fold to 8-fold increase in urinary GC or fGCM contents. Although the small sample size prevented statistical analysis, there were indications of individual differences, for example the higher baseline GC and fGCM excreted by the female in compared to its counterparts. In general, there was a spike in urinary GC content on the day of physical manipulations. There was a consistent 18–24 h lag between the observed urinary GC peak and the subsequent maximum fGCM value. Concentrations of both GC and fGCM generally returned to baseline within ∼24 h. Thus, both urinary GC and fGCM patterns evidently accurately reflected the adrenocortical response to a known stressor.
Figure 1. Urinary cortisol (–•–) per mg creatinine (Cr) and fGCM (– ∇ –), specifically 11,17-DOA, concentrations per g DW over 25 days in (A) a 5-year old, (B) a 15-year old and (C) a 17-year old female Persian onager. Day-matched urine and fecal samples were collected from each individual over a 25 day interval when the animals were occasionally (at least 48 h apart) exposed to physical restraint and examination (arrows). DW, dry weight.
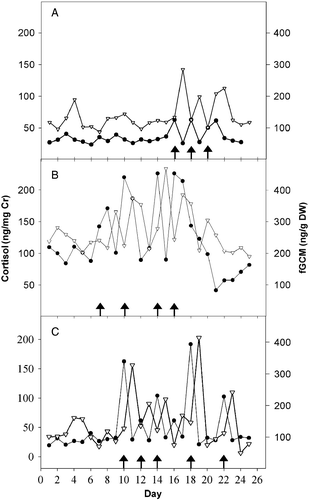
Influence of enclosure size and human proximity on adrenal activity
Initially, all onagers were maintained for at least 1 year in the large pasture. Each female served as its own control. Beginning March 27, 2007, five onagers were transported to a smaller yard while the other animals remained in the large pasture. This first group of five remained in smaller yards for 15 months and then returned to open pasture. Pasture samples for the five females in the second group were collected while the first group was maintained in yards in March 2008. Beginning April 1, 2008, the remaining five were translocated to yards for at least 15 months. During both years, the transfer involved initial herding of the onagers as a group slowly from the pasture into a corral using a vehicle and a grain ration as enticement. Females were then herded by vehicle and on foot through a series of corridors into the smaller yard before being sorted. Females not included in the study were separated from the herd by three animal managers on foot and using a gate between two yards. Sorted females were returned to pasture using a reverse of the above methods. This entire process required ∼1 h.
Because it was not possible to collect urine samples in the tall grasses of the large pasture, only fecal samples were evaluated. Three, 30-day sampling periods were undertaken: (1) while animals were maintained in the large pasture; (2) immediately after moving the group to the smaller yard which was in proximity to significant human activities (see above); and (3) again beginning 60 days after being placed in the yard (presumed post-acclimation). On average, 8–10 fecal samples were collected per individual while on pasture compared to ∼5 days per week from animals during the yard and post-acclimation phases. Samples were collected and processed as described above. One female (during the second year) was prematurely returned to pasture 25 days after moving into the yard because of continued refractory behaviors.
Influence of season on GC production
As nine of the 10 onagers were managed in the yards for at least 13 months, we took the opportunity to evaluate possible seasonal variations in GC patterns. Because the validation trial demonstrated equal ability to measure adrenal function via urine or feces, we chose only to evaluate urine for GC content. Urine samples were collected 5–7 days weekly for 13 months, processed, stored, and later analyzed as described above. Seasons were defined as spring, 21 March–20 June; summer, 21 June–20 September; fall (autumn), 21 September–20 December; and winter, 21 December–20 March. The first 30 days after moving into the smaller yards were excluded from the seasonal analysis.
Statistical analysis
For seasonal assessments, urinary GC concentrations were compared over time using two-way analysis of variance (Sigma Stat 3.5, Systat Software, Inc., Chicago, IL, USA) with GC (ng mg− 1 Cr) as the dependent variable and individual onager with season or month as independent variables. When significant effects were determined, post hoc tests for multiple pair-wise comparisons were performed using the Holm–Sidak method to evaluate differences among individuals within season and month and the converse, differences between season and month among individuals. To identify potentially influential data points (outliers), the procedures DFFITS, Leverage, and Cook's Distance were performed using SigmaStat (Version 3.5). No outliers were identified.
To assess the influence of enclosure size and human proximity on adrenal activity, general linear model analysis (PROC GLM; SAS version 9.1; SAS Institute, Cary, NC, USA) was performed to determine the differences in fGCM concentrations among the three evaluation phases (pasture versus yard versus post-acclimation). Because no differences were observed between females studied in years 1 and 2, fGCM concentration data were pooled for evaluation. Post hoc Tukey's studentized range tests were performed to determine significant differences between phases. Finally, daily changes in fGCM for the 30 days after translocation into smaller yards were analyzed using general linear model analysis and a post hoc Dunnett's test, with mean fGCM in pasture serving as the control. All data sets evaluating group or overall means passed tests for normality using the Kolmogorov–Smirnov test and exhibited skewness and kurtosis values < |1| indicating normal distribution. Mean values are reported as mean ± SEM, and P < 0.05 was considered statistically significant.
Results
Influence of enclosure size and human proximity on adrenal activity
While onagers were managed under spacious pasture conditions, there was little variation within and among animals in fGCM excretion, which did not vary by more than two SDs from the mean. Moving onagers from the pasture to the small yards combined with more exposure to human-related activities increased fGCM excretion by ∼ 2-fold (F(2, 213) = 29.57, P < 0.0001; ). This response was observed in all 10 animals. However, magnitude of the response, as measured by fold increase in metabolite production from the week before to the week after the perturbation, varied among animals (range, 0.5- to 4-fold; F(9, 260) = 6.91, P < 0.0001; for representative individual profiles). Post hoc Tukey's test revealed that mean fGCM values were similar (P>0.05) between the pasture and yard conditions by 60 days after translocation (). However, the temporal patterns for reaching this time point varied among onagers (). In three of 10 females, the fGCM elevation was modest and transient (). Two females exhibited erratic fGCM excretion patterns post-translocation that approached pasture levels only at the end of the 30-day period (). Four of 10 females initially experienced at least a 2-fold fGCM increase compared to levels measured while in the pasture, an elevation that quickly decreased within days of transfer (). Finally, one individual failed to acclimatize to the small yard enclosure (and associated human activities) based on persistently elevated fGCM concentrations () and consistently nervous, erratic behaviors. For humane reasons, this female was returned to the pasture before the end of the sampling period.
Figure 2. Mean ( ± SEM) fGCM concentrations in Persian onagers (n = 10) while maintained in a spacious pasture, in smaller yards with increased human interaction (yard) and 2 months after translocation to yard conditions (acclimation). DW, dry weight. Different letters indicate significant differences (post hoc Tukey's studentized range test for multiple comparisons; P < 0.05).
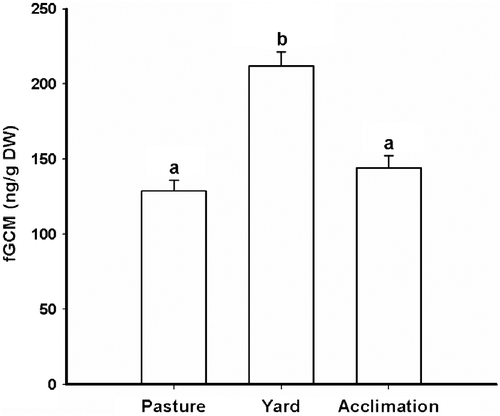
Figure 3. Profiles of fGCM in four representative female Persian onagers (A–D) relative to time of transport from spacious pasture to smaller yards. The dashed vertical line represents day of transport (Day 0). Concentrations depicted prior to Day 0 represent eight samples collected from each onager while in large pasture over 30 days (i.e. not values for consecutive days). The female in profile D failed to acclimate and was transported back to pasture on Day 25 because of continuous refractory behavior while maintained in yards. DW, dry weight.
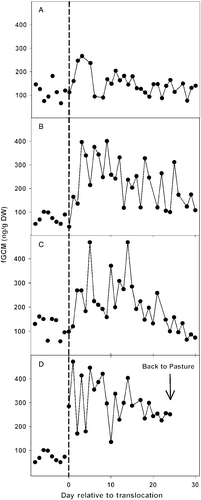
To determine the average time required to acclimatize to translocation and increased human activity, we calculated the mean fGCM concentrations for all onagers while on pasture (129 ± 7 ng g− 1, DW). Taking into account individual variability in the model, an average of 17 days was required for adrenal activity in yard conditions to return to concentrations similar to those observed in pasture (F(38, 260) = 4.62, P < 0.001; ). Once fGCM concentrations reached this “baseline,” the females displayed consistent excretion profiles that were no different from those measured under pasture conditions.
Figure 4. Mean ( ± SEM) concentrations of fGCM over 30 consecutive days after translocation (Day 0) from pasture to smaller yards (n = 10, days 1–25; n = 9, days 25–30). The straight, dashed line represents a single value for the overall mean fGCM; during the entire period females were sampled while in pasture. DW, dry weight. Asterisks indicate days when fGCM concentrations produced by animals in the yard were significantly elevated (post hoc Dunnett's test for comparison to a control, P < 0.05) compared to the overall mean while in the pasture.
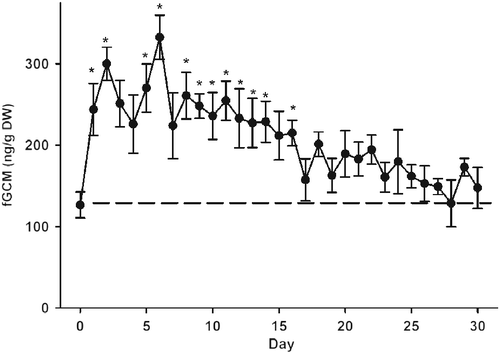
Influence of season on GC production
There was seasonal variation in GC output in female onagers, with lower concentrations in winter compared to spring, summer, and fall (F(3, 2028) = 18.22, P < 0.05; ). More specifically, mean urinary GC content was at nadir during February and March (). There also was an individual female effect (F(8, 2028) = 110.617, P < 0.001) on seasonal GC activity patterns in urine. Post hoc Holm–Sidak tests for multiple comparisons revealed that two of nine females produced higher overall mean urinary GC concentrations than all other females (mean ± SD; 75.1 ± 28.3 and 60.8 ± 18.9, respectively, compared to (mean ± SEM) 40.5 ± 2.3 ng mg− 1 Cr of the other females; P < 0.001). Anecdotally, these two females were the most submissive in the group and quickest to shy away from human proximity.
Figure 5. Mean ( ± SEM) urinary cortisol per milligram of creatinine (Cr) in female Persian onagers (n = 9) by (A) month (JAN, January; FEB, February; MAR, March; APR. April; JUN, June, JUL, July; AUG, August; SEP, September; OCT, October; NOV, November; DEC, December) and (B) season (SPR, spring; SUM, summer; FALL, autumn; WIN, winter). Within each panel, different letters indicate significant differences (post hoc Holm–Sidak test for multiple comparisons, P < 0.05) among months or seasons.
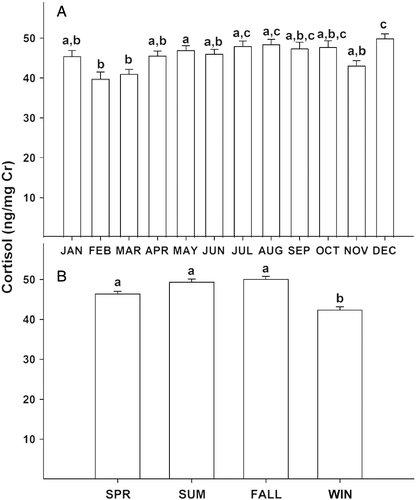
Discussion
The objective of this study was primarily to understand adrenal activity in this species in response to environmental (spatial and human exposure) change and over time. We made three major findings. First, we validated that acute stress was quantifiable by measuring GC metabolites in onager urine or feces. Second, although this equid appeared to produce consistent fGCM concentrations in the more naturalistic conditions of the spacious pasture, there was a marked increase in adrenocortical activity after confinement to small yards and greater exposure to human activities. However, almost all females rapidly acclimated to this environmental change, with fGCM contents returning to baseline within < 3 weeks. Third, there were individual variations in baseline GC concentrations as well as modestly lower levels in at least one season (winter). Collectively, the data demonstrated that non-invasive monitoring tools were useful for beginning to understand the physiology of this rare equid species. Our discovery that the onager had the capacity to acclimate to a radically different environment, at least with respect to GC output, has management implications. Large ungulates ideally should be maintained under spacious conditions. However, our findings suggest that if necessary, the well-being of onagers could be sustained in smaller enclosures typical of traditional zoological institutions.
Handling and restraint were clearly stressors for this non-domestic equid, as demonstrated by marked spikes in GC excretion after brief restraint in the initial validation trial. The observed rise in urinary GC after 4 h and in fGCM ∼20 h after manipulation confirmed a successful biological validation of the non-invasive methods. In two of the three females, GCs remained elevated for more than 24 h post-restraint, suggesting that this species may be less adapted to handling than the domestic horse (Berghold et al. Citation2007; Gorgasser et al. Citation2007). Physical restraint has been reported to result in acute increases in fGCM content in the rhinoceros (Turner et al. Citation2002) as well as in plasma cortisol in wild ungulates (Morton et al. Citation1995; Phillips et al. Citation1998). Measured lag times of GC excretion in onager urine and feces were similar to those reported in the domestic horse (Palme et al. Citation1996; Möstl et al. Citation1999).
Increased fGCM content has been reported in horses after transportation (Schmidt et al. Citation2010). However, another study indicated no increase in fGCM content in response to modest, first-time handing, including exposure to a saddle and rider (Gorgasser et al. Citation2007). The difference between the domestic horse and the onager no doubt is related to the domestication of the former versus the documented (Bush Citation1839; Diamond Citation1997) temperamental and stubborn nature of the latter, in addition to differences in restraint methods. Despite these, an ideal response to such an acute stressor is a quick, but transient activation of the HPA axis, which is present in onagers managed in captivity.
Translocation of onagers from spacious pasture to small yards with increased human proximity markedly increased adrenocortical activity. The movement event itself was likely a stressor, with an almost immediate doubling in fGCM production. This observation was similar to the 2-fold increase in fGCM in Grevy's zebras (Equus grevyi) captured from the wild and then moved by vehicle ∼165 km over ∼4 h (Franceschini et al. Citation2008). The zebras failed to acclimate while held in pens for the next 5–6 weeks, but fGCM concentrations returned to baseline 2–9 weeks after being released into a national park. Linklater et al. (Citation2010) reported a gradual decline of fGCM concentrations after capture and holding to below “pre-capture” levels in black rhinoceros. These investigators suggested that this pattern (along with a parallel decline in gonadal hormones) reflected distress, rather than acclimation. Contrary to the findings of the zebra and rhinoceros studies, we observed onagers rapidly acclimating to the acute stressor of translocation and smaller enclosures with increased human proximity. Our study animals then sustained fGCM concentrations that were similar to (but not below) what was measured under large pasture conditions. Thus, there may be differences among related species in ability to adapt to changes in environment. It is also possible that onagers in this study already had adapted in some way to long-term captivity, despite being managed in expansive enclosures. Interestingly, CitationCoburn et al. (2010) found that wild-caught bighorn sheep had GC responses to repeated handling similar to those of captivity-reared counterparts, which suggests that other ungulates also have rapid acclimation capacity to new environments.
Some wildlife species, however, may not adapt well to captivity. For example, long-term increases in GC production have been recorded in populations of dama gazelle (G. dama mhorr; Cassinello and Pieters Citation2000) and Père David's deer (E. davidianus; Li et al. Citation2007), which failed to fully acclimate to certain spatial restrictions. Of course, it is difficult to separate the influence of other potentially confounding environmental factors. For example, human proximity can provoke increased adrenal activity in wild ungulates, including the pampas deer (Ozotoceros bezarticus bezoarticus; Pereira et al. Citation2006), elephant (Loxodonta africana; Stead et al. Citation2000), and black rhinoceros (Carlstead and Brown Citation2005).
Unlike what has been demonstrated for other wild ungulates, the onager appears to acclimatize relatively quickly (within 3 weeks) to substantive environmental change. However, the response varied among individuals, with at least one female failing to acclimate. As the rise in GC levels was almost always transient, this species seems to be well adapted to alterations in intensive management protocols. A similar situation has been reported by Christofoletti et al. (Citation2010) for marsh deer (Blastocerus dichotomus), but not brown brocket deer (Mazama gouazoubira), indicating that the ability to adapt to changes in captive environments is likely species-specific.
There is a surprising lack of information on circannual GC rhythms in any equid species. Gill et al. (Citation1985) reported inconsistencies in seasonal serum GC production between 2 years of study in domestic mares, and Flisinska-Bojanowska et al. (Citation1991) observed seasonal fluctuations in pregnant, but not barren mares. In one review, seasonal variation in baseline GC level was observed in 88% of 31 free-ranging mammalian species, but the season of peak values was species-specific (Schiml and Rissman Citation1999; Romero Citation2002). Seasonality in GC production appears consistent in amphibians, reptiles, and birds, in which GC release is highest during the breeding season, presumably as a mechanism for helping to mobilize energy stores to improve breeding fitness and to modulate behaviors associated with competitive mating strategies (Romero Citation2002). There were only modest annual variations in GC output in the onager, with nadir values in the winter and comparatively higher GC concentrations from April through September, a period generally recognized as the breeding and foaling season (Feh et al. Citation2002). However, our study discovered no profound circannual fluctuation in GC output. Furthermore, it is worth noting that the period of lowest GC production coincided with the time of year when there were fewest public visitors. Therefore, the significant fluctuation observed in longitudinal corticoid production (lower values in winter) may have been affected by less exposure to people.
Although our study was not designed to quantify specific behaviors, there were no notable differences in social structure, including aggressive or affiliative behavior in onagers while on pasture versus in the smaller yards. There were two subordinate animals that were more nervous and excitable (e.g. pacing, shifting weight between legs [weaving], and head rolling) and simultaneously showed the highest fGCM values when in smaller yards. Among all the study animals, there also were significant variations in baseline GC output as well as short- and long-term responses to environmental perturbations. Social status may also play a role, as subordinate herd members can exhibit elevated GC production in the domestic horse (Alexander and Irvine Citation1998).
Taken together, these results have significant implications for understanding the biology of stress and for the future study and management of onagers and other equids. First, we determined that both urine and feces were suitable and biologically meaningful, non-invasive resources for tracking adrenocortical activity in this species. Second, the results of this study highlight the value of non-invasive GC monitoring for beginning to understand the impacts of various environmental conditions on the biology of non-domestic equids. This study confirms that species-specific variations exist in GC response to acute stressors and environment and highlights the need for continued assessments of the impact of environmental variables in non-domestic species. Additionally, it appears worthwhile that future studies consider evaluating the implications of environmental change on other essential biological functions, for example social and reproductive capacities, namely ovarian cyclicity, and the ability to mate, conceive, and carry young to term (Friend Citation1991; von Borell Citation2001). From a practical perspective, our findings help to affirm the resiliency of this species managed in ex situ collections, and demonstrate that it is prudent to allow at least 30 days between exposing onagers to major change and beginning to assess normative data or the influence of experimental treatments. Nonetheless, finding that the onager readily acclimated to intensive management changes indicates that this species is appropriate for most zoological institutions. This characteristic, in turn, has the potential of increasing availability of space for breeding and production to create a self-sustaining population of this critically endangered species.
Acknowledgments
This study was conducted under a consortium agreement of the Conservation Centers for Species Survival (C2S2), a partnership that shares unique resources to improve the biological understanding and management of endangered species, especially those that require space, natural group sizes, and scientific research. The work was supported by the Sichel Endowment Fund and Philip D. Reed, Jr. The authors thank the Wilds staff for assisting in animal handling; Dr James Dooley (Muskingum University) for student mentorship; Dr Janine Brown (Smithsonian Conservation Biology Institute) for advice on data analysis and interpretation; and interns Shoshana Sommer, Tara Strickler, Natalie Sacks, Emma Corrigan, Elise Gicola, Stacy Price, Amy Flaggs, Travis Smith, and Kate Archibald for assistance with sample collection, processing, and analysis.
Declaration of interest: The authors did not have any conflicts of interest related to the work presented in this manuscript. The authors alone are responsible for the content and writing of the paper.
References
- Alexander SL, Irvine CHG. 1998. The effect of social stress on adrenal axis activity in horses: The importance of monitoring corticosteroid-binding globulin capacity. J Endocrinol. 157:425–432.
- Berghold P, Möstl E, Aurich C. 2007. Effects of reproductive status and management on cortisol secretion and fertility of oestrous horse mares. Anim Reprod Sci. 102:276–285.
- Brown JL, Bellem AC, Fouraker M, Wildt DE, Roth TL. 2002. Comparative analysis of gonadal and adrenal activity in male and female black and white rhinoceroses in North America by non-invasive endocrine monitoring. Zoo Biol. 20:463–486.
- . 1839. Illustrations of the holy scripturesBush RG. Battleboro, VT: Battleboro Typographic Co656.
- Carlstead K, Brown JL. 2005. Relationships between patterns of fecal corticoid excretion and behavior, reproduction and environmental factors in captive black (Diceros bicornis) and white (Ceratotherium simum) rhinoceros. Zoo Biol. 24:215–232.
- Carlstead K, Fraser J, Bennet C, Kleiman D. 1999. Black rhinoceros (Diceros biocornis) in US zoos: II. Behavior, breeding success and mortality in relation to housing facilities. Zoo Biol. 18:35–52.
- Carrieri M, Trevisan A, Bartolucci GB. 2001. Adjustment to concentration–dilution of spot urine samples: Correlation between specific gravity and creatinine. Int Arch Occup Environ Health. 74:63–67.
- Cassinello J, Pieters I. 2000. Multi-male captive groups of endangered dama gazelle: Social rank, aggression and enclosure effects. Zoo Biol. 19:121–129.
- Christofoletti MD, Pereira RJG, Duarte JMB. 2010. Influence of husbandry systems on physiological stress reactions of captive brown brocket (Mazama gouazoubira) and marsh deer (Blastocerus dichotomus): Noninvasive analysis of fecal cortisol metabolites. Eur J Wildl Res. 56:561–568.
- Coburn S, Salman M, Rhyan J, Keefe T, McCollum M, Aune K, Spraker T, Miller L. 2008. Comparison of endocrine response to stress between captive-raised and wild-caught bighorn sheep. J Wildl Manage. 74:532–538.
- Creel S, Fox JE, Hardy A, Sands J, Garrott B, Peterson RO. 2002. Snowmobile activity and glucocorticoid stress responses in wolves and elk. Conserv Biol. 16:809–814.
- Diamond JM. 1997. Guns, germs and steel: The fates of human societies. New York: Norton and Co., Inc p 480.
- Dobson H, Smith RF. 1995. Stress and reproduction in farm animals. J Reprod Fertil Suppl. 49:451–461.
- Feh C, Shah N, Rowen M, Reading R, Goyal SP. 2002. Status and action plan for the Asiatic wild ass. In: Moehlman PD. editors. Zebras, asses and horses: Status survey and conservation action plan. Gland Switzerland and Cambridge: IUCN/SCC Equid Specialist Group, IUCN (The World Conservation Union)62–71.
- Flisinska-Bojanowska A, Komosa M, Gill J. 1991. Influence of pregnancy on diurnal and seasonal changes in cortisol, T3 and T4 levels in the mare blood serum. Comp Biochem Physiol A-Physiol. 98:23–30.
- Franceschini MD, Rubenstein DI, Low B, Romero LM. 2008. Fecal glucocorticoid metabolite analysis as an indicator of stress during translocation and acclimation in an endangered large mammal, the Grevy's zebra. Anim Conserv. 11:263–269.
- Friend TH. 1991. Behavioral aspects of stress. J Dairy Sci. 74:292–303.
- Gill J, Kompanowskajezierska E, Jakubow K, Kott A, Szumska D. 1985. Seasonal changes in the white blood cell system, lyzozyme activity and cortisol level in Arabian brood mares and their foals. Comp Biochem Physiol A-Physiol. 81:511–523.
- Gorgasser I, Tichy A, Palme R. 2007. Faecal cortisol metabolites in Quarter horses during initial training under field conditions. Wien Tierarztl Monatsschr. 94:226–230.
- Gronwall R, Price G. 1985. Estimation of urine flow rate in mares. Am J Vet Res. 46:1107–1110.
- Hennessy JW, Levine S. 1979. Stress, arousal and the pituitary–adrenal system: A psychoendocrine hypothesis. Prog Psychobiol Physiol Psychol. 8:133–178.
- Huber S, Palme R, Zenker W, Möstl E. 2003. Non-invasive monitoring of the adrenocortical response in red deer. J Wildl Manage. 67:258–266.
- IUCN. 2010. IUCN red list of threatened species, Version 2010.1. < www.iucnredlist.org>. Downloaded on 11 June 2010.
- Kaczensky P, Yadamsuren A, Walzer C. 2007. Asiatic wild ass and wild camel collaring mission in the great Gobi: A strictly protected area in Mongolia Landscape Level Research for the Conservation of Asiatic Wild Ass in Mongolia: A Report. Research Institute of Wildlife Ecology. Vienne, Austria: University of Veterinary Medicine14.
- Li CW, Jiang ZG, Tang SH, Zeng Y. 2007. Influence of enclosure size and animal density on fecal cortisol concentration and aggression in Pere David's deer stags. Gen Comp Endocrinol. 151:202–209.
- Linklater WL, MacDonald EA, Flamand JRB, Czekala NM. 2010. Declining and low fecal corticoids are associated with distress, not acclimation to stress, during the translocation of African rhinoceros. Anim Conserv. 13:104–111.
- Liptrap RM. 1993. Stress and reproduction in domestic animals. Ann N Y Acad Sci. 697:275–284.
- McBride SD, Cuddelford D. 2001. The putative welfare reducing effects of preventing equine stereotypic behaviour. Anim Welf. 10:173–189.
- Merl S, Scherzer S, Palme R, Möstl E. 2000. Pain causes increased concentrations of glucocorticoid metabolites in horse feces. J Eq Vet Sci. 20:586–590.
- . 2002. Equids: Zebras, asses and horses: Status survey and conservation action plan. In: Moehlman PD. editors. . Gland Switzerland and Cambridge: IUCN (The World Conservation Union)185.
- Monfort SL. 2002. Non-invasive endocrine measures of reproduction and stress in wild populations. In: Holt WV, Pickard A, Rodger J, Wildt DE. editors. Reproduction and integrated conservation science. Cambridge, UK: Cambridge University Press147–165.
- Morton DJ, Anderson E, Foggin CM, Kock MD, Tiran EP. 1995. Plasma cortisol as an indicator of stress due to capture and translocation in wildlife species. Vet Rec. 136:60–63.
- Möstl E, Messmann S, Bagu E, Robia C, Palme R. 1999. Measurement of glucocorticoid metabolite concentrations in faeces of domestic livestock. Zentralbl Vet A. 46:621–631.
- Munck A, Guyre PM, Holbrook NJ. 1984. Physiological functions of glucocorticoids in stress and their relation to pharmacological actions. Endocr Rev. 5:25–44.
- Munro CJ, Stabenfeldt GH, Cragun JR, Addiego LA, Overstreet JW, Lasley BL. 1991. Relationship of serum estradiol and progesterone concentrations to the excretion profiles of their major urinary metabolites as measured by enzyme immunoassay and radioimmunoassay. Clin Chem. 37:838–844.
- Palme R, Möstl E. 1997. Measurement of cortisol metabolites in faeces of sheep as a parameter of cortisol concentration in blood. Z Saugetierkd Int J Mammal Biol. 62:192–197.
- Palme R, Fischer P, Schildorfer H, Ismail MW. 1996. Excretion of 14C-steroid hormones via feces and urine in domestic livestock. Anim Reprod Sci. 43:43–63.
- Palme R, Rettenbacher S, Touma C, El-Bahr SM, Möstl E. 2005. Stress hormones in mammals and birds: Comparative aspects regarding metabolism, excretion and noninvasive measurement in fecal samples. Ann N Y Acad Sci. 1040:162–171.
- Pereira RJG, Duarte JMB, Negrao JA. 2006. Effects of environmental conditions, human activity, reproduction, antler cycle and grouping on fecal glucocorticoids of free-ranging Pampas deer stags (Ozotoceros bezoarticus bezoarticus). Horm Behav. 49:114–122.
- Phillips M, Grandin T, Graffam W, Irlbeck NA, Cambre RC. 1998. Crate conditioning of bongo (Tragelaphus eurycerus) for veterinary and husbandry procedures at the Denver Zoological Gardens. Zoo Biol. 17:25–32.
- . 2008. Internationales Zuchtbuch für HalbeselPohle C. Berlin, Germany: Tierpark Berlin-Fredrichsfelde23.
- Romero LM. 2002. Seasonal changes in plasma glucocorticoid concentrations in free-living vertebrates. Gen Comp Endocrinol. 128:1–24.
- Sapolsky RM, Romero LM, Munck AU. 2000. How do glucocorticoids influence stress responses? Integrating permissive, suppressive, stimulatory and preparative actions. Endocr Rev. 21:55–89.
- Schiml PA, Rissman EF. 1999. Cortisol facilitates induction of sexual behavior in the female musk shrew (Suncus murinus). Behav Neurosci. 113:166–175.
- Schmidt A, Möstl E, Wehnert C, Aurich J, Müller J, Aurich C. 2010. Cortisol release and heart rate variabilty in horses during road transport of one, 3.5 and 8 hours duration. Horm Behav. 57:209–215.
- Stead SK, Meltzer DGA, Palme R. 2000. The measurement of glucocorticoid concentrations in the serum and faeces of captive African elephants (Loxodonta africana) after ACTH stimulation. J S Afr Vet Assoc. 71:192–196.
- Touma C, Palme R. 2005. Measuring fecal glucocorticoid metabolites in mammals and birds: The importance of validation. Ann N Y Acad Sci. 1046:54–74.
- Turner JWJr, Tolson P, Hamad N. 2002. Remote assessment of stress in white rhinoceros (Ceratotherium simum) and black rhinoceros (Diceros bicornis) by measurement of adrenal steroids in feces. J Zoo Wildl Med. 33:214–221.
- . 2009. North American regional onager studbook (Equus hemionus onager)Volz K. Washington, DC: Smithsonian National Zoological Park34.
- von Borell EH. 2001. The biology of stress and its application to livestock housing and transportation assessment. J Anim Sci. 79 Suppl: E260–E267.