Abstract
This study examined the role of sympathetic nerves in the control of cerebral hemodynamics during air-jet stress. In adult male Sprague-Dawley rats, blood flow velocity (pulsed Doppler) was measured in both internal carotid arteries 1 week after excision of one superior cervical ganglion. Blood pressure (BP) and carotid blood flows (CBFs) were simultaneously recorded during exposure to air-jet stress. In 5 out of 13 rats, stress was applied after β2-adrenoceptor blockade with ICI 118551 (0.4 mg/kg, then 0.2 mg/kg/h, i.v). Stress evoked an immediate rise in BP, CBFs, and vascular conductances. Vasodilatation was much larger on the denervated side than on the intact side (mean ± SEM: 78 ± 7 versus 19 ± 4%; P < 0.02) and lasted about 10 s. Thereafter, blood flows returned to or near normal and showed parallel variations while BP remained elevated. There was, therefore, a net vasoconstriction on both sides. In ICI 118551-treated rats, the initial vasodilatation was not significantly reduced on the denervated side (64 ± 4%), but the subsequent vasoconstriction was enhanced (P < 0.05) on both sides. In conclusion, air-jet stress evokes an immediate, short-lasting vasodilatation through a mechanism unrelated to β2-adrenoceptor stimulation. Sympathetic nerves powerfully limit this phenomenon, and thus contribute to protect the cerebral circulation from stress-induced BP surges.
Introduction
It has long been suggested that the sympathetic nervous system contributes to protecting the cerebral microcirculation by facilitating vasoconstriction of cerebral resistance vessels in response to increases in blood pressure (BP) (Sadoshima et al. Citation1983). This concept was mainly supported by experiments carried out in anesthetized animals in which BP increases were evoked through pharmacological or mechanical interventions. In these studies, it was shown that electrical stimulation of cerebral sympathetic nerves efficiently limited the impact of the BP rises on cerebral blood flow (Busija et al. Citation1980). There have been few attempts to examine the protective role of the sympathetic nervous system under real-life conditions, i.e. in conscious animals during spontaneous increases in BP. In 2005, Loos et al. reported that increases in cerebral blood flow occurring during the natural BP surges of rapid-eye-movement (REM) sleep were enhanced after removal of the superior cervical ganglia (SCG) in lambs. Recently, Cassaglia et al. (Citation2009) have described the fluctuations of cerebral sympathetic nerve activity in sleeping lambs. They observed that sympathetic nerve activity collected from the SCG increased during the transient BP surges of REM sleep. Surprisingly, little is known about the role of sympathetic nerves in the control of cerebral hemodynamics during a most common situation of daily life, i.e. exposure to emotional stressors.
In rats, stress can readily be elicited by blowing a jet of air into the cage (Julien et al. Citation1988; Bajić et al. Citation2010). This procedure has been shown to evoke the whole pattern of cardiovascular changes typical of the defense reaction, namely increases in BP, heart rate, and renal sympathetic nerve activity, vasoconstriction in visceral organs and vasodilatation in skeletal muscles (Zhang et al. Citation1996; Barrès et al. Citation2004). The objective of the present study was to examine the effects of cerebral sympathetic denervation on cerebrovascular responses to air-jet stress in rats, to test the hypothesis that sympathetic denervation would magnify the effects of stress-induced increases in BP on cerebral hemodynamics. For this purpose, blood flow was measured in both internal carotid arteries and was assumed to provide an approximation of cerebral blood flow in each hemibrain (Kolb et al. Citation2007). One SCG was excised at the time of probe implantation. It has been shown that chronic ganglionectomy achieves an almost complete unilateral sympathectomy (Kobayashi et al. Citation1983; Handa et al. Citation1991). When it is carried out in adult animals, denervation has little or no effect on vascular structure (Bevan and Tsuru Citation1981) and, therefore, differences in hemodynamic responses can be safely attributed to the presence or absence of sympathetic nerves. Responses of carotid blood flows (CBFs) to air-jet stress were compared in control rats and in rats under blockade of β2-adrenoceptors. Stimulation of these receptors has indeed been shown to play a major role in hemodynamic adjustments accompanying air-jet stress in rats (Zhang et al. Citation1996).
Materials and methods
Animals and chronic instrumentation
Experiments were carried out on 10–12-week-old male Sprague-Dawley rats (Charles River Laboratories, L'Arbresle, France) and were approved by the local Animal Ethics Committee. Groups of 2–3 rats were housed under controlled conditions (temperature: 21 ± 1°C, light period: 08:00–20:00 h), and had free access to food and water. After the first surgery, rats were housed in individual cages.
One week before the study, rats were anesthetized with a mixture of xylazine (8 mg/kg i.p.), acepromazine maleate (4 mg/kg i.p.), and ketamine hydrochloride (40 mg/kg i.p.), and received prophylactic injections of penicillin G (50,000 IU s.c.) and ketoprofen (5 mg/kg s.c.). Left and right internal carotid arteries were exposed, and the right SCG was excised. Pulsed Doppler flow probes with a 0.8 mm lumen diameter (Iowa Doppler Products, Iowa City, IA, USA) were placed around both internal carotid arteries. Probe wires were tied to the sternohyoideus muscles. Wires and connectors were tunneled subcutaneously to exit at the back of the neck and were fixed to the skull with dental screws and cement. Rats were allowed to recover from surgery for 6 days. On the seventh day after surgery, under isoflurane anesthesia (2% in oxygen), a polyethylene catheter was inserted into the left femoral artery for BP recording. A catheter was also inserted into the left femoral vein for drug administration. Rats were allowed to recover from anesthesia overnight.
Data collection and experimental protocol
CBF velocities (expressed in kHz of Doppler shift) were recorded by connecting the probes via the head plug to a directional pulsed Doppler flowmeter (Department of Bioengineering, University of Iowa, Iowa City, IA, USA). BP was measured using a pressure transducer (model TNF-R; Becton Dickinson, Sandy, UT, USA) coupled to an amplifier (model 13-3615-52, Gould, Cleveland, OH, USA).
After a 1- to 2-h acclimatization period, BP and both CBFs were continuously recorded by a computer equipped with a 12-bit A/D converter (model MIO-16-E-10; National Instruments, Austin, TX, USA) and LabVIEW 5.1 software (National Instruments) at 500-Hz sampling rate. Mild stress was elicited by means of a jet of air blown into the cage for 5 min with an air compressor. Stress trials were always carried out between 14:00 and 15:00 h when rats were awake in a quiet, resting state.
In a separate group of rats, the air-jet stress was applied after β2-adrenoceptor blockade with ICI 118551 (0.4 mg/kg, then 0.2 mg/kg/h i.v). Pilot experiments indicated that this dose was sufficient to almost abolish cardiovascular responses to isoprenaline (0.5 μg/kg i.v).
At the end of the experiment, rats were euthanized with an intravenous overdose (>100 mg/kg) of pentobarbital sodium.
Data analysis
Individual 500-Hz data files were first replayed and carefully examined to eliminate occasionally occurring artifacts. BP and CBF time series were then resampled at 1 Hz by averaging over consecutive 1-s periods, which provided mean arterial BP and mean CBF. For comparison purpose, each CBF and corresponding carotid vascular conductance (CVC, calculated as the ratio of CBF to BP) were normalized by its mean value calculated over the 60-s period preceding application of the air-jet stress. Quantification of responses to stress included calculation of maximum changes as well as overall changes that were estimated as the sum of areas under and over the curves with the 60-s mean value used for normalization being taken as the reference baseline.
Statistics
All statistical analyses were carried out using VassarStats (http://faculty.vassar.edu/lowry/vassarstats.html). Paired comparisons (denervated side versus intact side) were carried out using the Wilcoxon signed-rank test. The Mann–Whitney test was used for unpaired comparisons (control group data versus ICI 118551-treated group data). Results are expressed as means ± SEM. Values of P < 0.05 were considered significant.
Results
Air-jet stress evoked an immediate rise in BP and in both CBFs and CVCs (). Increases in CBF and CVC were much more marked on the denervated side than on the intact side ( and ). This difference peaked at ∼5 s and lasted ∼10 s (). Then, CBFs returned to or near normal levels whereas CVCs became lowered, pointing to a net vasoconstriction on both sides.
Figure 1. Effects of air-jet stress on BP, CBFs, and vascular conductances in rats with unilateral cervical ganglionectomy. Rats were either untreated (control, n = 8) or were receiving an infusion of a β2-adrenoceptor blocker (ICI 118551, n = 5). SE lines have been omitted for legibility. Vertical dashed lines show the onset of the stress trial. The inset graphs show a zoom in of the initial response of CBFs and vascular conductances. Note the much stronger increase in flow and conductance on the denervated side.
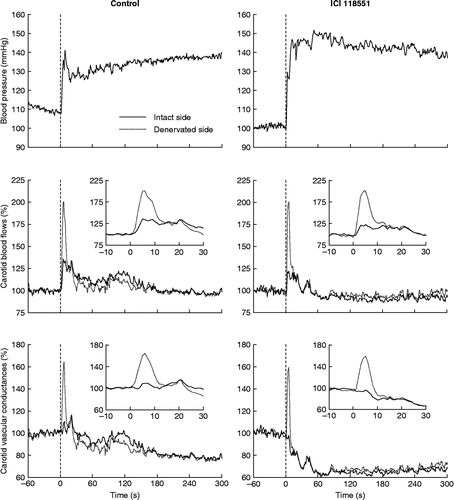
Table I. Immediate cerebrovascular responses to air-jet stress in rats with unilateral cervical ganglionectomy.
Infusion of ICI 118551 did not affect basal BP (1-h mean values of BP were 108 ± 4 and 109 ± 6 mmHg before and during infusion, respectively, P = 0.345 from Wilcoxon signed-rank test). The sequence of stress-induced hemodynamic changes was not altered by β2-adrenoceptor blockade. The BP increase was, however, more progressive and its overall magnitude was significantly enhanced (). On the intact side, the initial increase in CBF was not significantly attenuated (P = 0.079 from Mann–Whitney test) and the vasodilator response was essentially abolished as compared with control rats ( and ). On the denervated side, the initial increases in CBF and CVC were not significantly altered (). On both sides, the late vasoconstrictor responses were enhanced (), which was reflected in the sum of areas under and over the curves ().
Figure 2. Overall responses of BP, CBFs, and vascular conductances to air-jet stress in conscious rats with unilateral cervical ganglionectomy. Rats were either untreated (control, n = 8) or were receiving an infusion of a β2-adrenoceptor blocker (ICI 118551, n = 5). For each variable, stress-induced changes were estimated as the sum of areas under and over the curves that were calculated from the stress onset up to the termination of the jet of air (5 min). Values are means ± SEM. *P < 0.05 versus respective control (Mann–Whitney test).
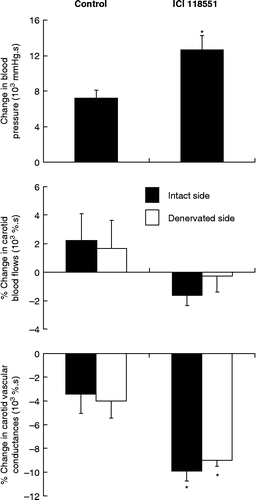
Discussion
The main finding of this study is that the cerebrovascular sympathetic innervation limits cerebral vasodilatation at the very beginning of exposure to an emotional stressor, and thus contributes to protect the cerebral circulation from stress-induced BP surges.
Methodological aspects
Capturing rapid changes in cerebral blood flow requires its continuous recording. In rats, laser Doppler flowmetry can provide a continuous index of cortical cerebral blood flow and this method has been adapted to the conscious freely moving animal (Gu et al. Citation1999). However, because of the size of the connecting device, the method can only measure blood flow on one side of the brain. As the main objective of the present study was to compare overall cerebrovascular responses to stress in intact and sympathetically denervated hemibrains, we chose to chronically implant pulsed Doppler probes on both internal carotid arteries. The pulsed Doppler technique measures blood velocity and thus does not provide absolute measures of flow, unless probes are calibrated in situ, preferably in the living animal, which is not possible for the internal carotid artery of the rat. Furthermore, the strength of the Doppler signal strongly depends on the angle between probe and blood vessel (Haywood et al. Citation1981). Slight differences in this angle may exist from artery to artery and from animal to animal, which will result in marked differences in the signal strength. This is especially true in the chronic situation in which small changes in probe position can occur following implantation. For this reason, only acute changes in blood flow can be assessed with this technique, and these changes must be normalized, i.e. expressed as percentage of a baseline value. An alternative method would have been to measure CBF with transit-time flow probes which measure true volumetric flow rate (Kolb et al. Citation2007). However, the size and rigidity of the transit-time probe and wire limit their use in the chronic setting. The small, soft, and flexible Doppler probes were usually well tolerated and all the rats included in the present study had regained or exceeded their initial bodyweight 6 days after surgery.
Unilateral ganglionectomy was carried out at the same time as probe implantation. The 1-week postoperative recovery time was an ideal duration to ensure complete degeneration of sympathetic nerves before the occurrence of significant reinnervation (Kobayashi et al. Citation1983, Handa et al. Citation1991).
Responses to air-jet stress
Air-jet stress evoked an immediate and short-lasting increase in CBF that was markedly enhanced on the ganglionectomized side. Yu and Blessing (Citation1997) also reported a transient increase in CBF at the onset of various alerting stimuli in rabbits, but they did not investigate the role of adrenergic mechanisms in this response. In the present study, acute β2-adrenoceptor blockade did not significantly attenuate this response on both sides, indicating that the mechanism of CBF increase was not stimulation of β2-adrenoceptors by circulating adrenaline. Calculation of CVCs revealed that the increase in CBF was mostly secondary to the rise in BP on the intact side, whereas a strong vasodilatation occurred on the denervated side. The mechanism of this vasodilatation cannot be inferred from the present study. An attractive working hypothesis would involve a flow-induced mechanism. Discrepant results have been reported regarding the effect of flow on cerebral vascular tone in rats. Flow-induced constriction has been observed in isolated cerebral vessels (Bryan et al. Citation2001), whereas flow-induced dilatation has been observed in vivo (Paravicini et al. Citation2006). The kinetics of the vasodilatation observed in the present study would be compatible with a shear stress-induced release of nitric oxide (Nafz et al. Citation1997). Alternatively, or additionally, passive distension of large cerebral arteries might account for at least part of the observed increase in CVC at the onset of stress. Whatever the mechanism of the early stress-induced vasodilatation, it was efficiently blunted by sympathetic nerves, possibly through a baroreflex mechanism, as suggested by the recent observation that phenylephrine increases sympathetic nerve activity in the SCG of anesthetized lambs (Cassaglia et al. Citation2008). Another possibility that cannot be excluded is that vascular tone was lower in denervated vessels. Consequently, pressure-induced arterial distension would be larger on the denervated side, resulting in an enhanced increase in CVC.
After the initial stress-induced increase in CBFs, flows returned to or near normal and vasoconstriction developed on both sides. This delayed vasoconstriction might be due, at least partly, to the action of increased levels of circulating noradrenaline. Other mechanisms such as the myogenic response are likely to be involved. The kinetics of the myogenic responses of the rat cerebral vasculature has been described (Kolb et al. Citation2007) and would be compatible with the kinetics of stress-induced vasoconstriction. After β2-adrenoceptor blockade, vasoconstrictor responses to stress were increased on both sides. It is of note that the pressor response to stress was also enhanced, most probably due to blunting of vasodilatation in skeletal muscles, as previously reported (Zhang et al. Citation1996). A larger stress-induced increase in BP would in turn evoke a stronger vasoconstrictor, myogenic response. Therefore, β2-adrenoceptors take part in the regulation of cerebrovascular tone during air-jet stress in rats, either directly through their stimulation by circulating adrenaline in the cerebral vasculature, or indirectly through blunting of a pressure-induced myogenic response.
Conclusion and perspectives
Recently, BP variability has been recognized as a strong predictor of stroke, independent of mean BP (Rothwell et al. Citation2010). In rats, pressor responses to stress are positively correlated with spontaneous BP variability (Julien et al. 1988) and thus, probably contribute to this BP variability. These responses are mediated by sympathetic nervous activation (Barron and Van Loon Citation1989). The present study brings direct support to the hypothesis that the sympathetic nervous system plays a protective role against stress-induced BP surges. The apparent paradox is that the same system is responsible for producing BP increases and for protecting the cerebral vasculature during air-jet stress. Our hypothesis is that a common regulatory mechanism, namely the arterial baroreceptor reflex, determines both the amplitude of the pressor response (Zhang et al. Citation1996; Barrès et al. Citation2004) and the strength of the sympathetically mediated cerebral vasoconstriction during stress. This demonstration can provide an explanation for the intriguing observation that baroreflex sensitivity and life expectancy are positively correlated in stroke prone hypertensive rats (Liu et al. Citation2007).
Declaration of interest: The authors report no conflicts of interest. The authors alone are responsible for the content and writing of the paper.
References
- Bajić D, Loncar-Turukalo T, Stojicić S, Sarenac O, Bojić T, Murphy D, Paton JF, Japundzić-Zigon N. 2010. Temporal analysis of the spontaneous baroreceptor reflex during mild emotional stress in the rat. Stress. 13:142–154.
- Barrès C, Cheng Y, Julien C. 2004. Steady-state and dynamic responses of renal sympathetic nerve activity to air-jet stress in sinoaortic denervated rats. Hypertension. 43:629–635.
- Barron BA, Van Loon GR. 1989. Role of sympathoadrenomedullary system in cardiovascular response to stress in rats. J Auton Nerv Syst. 28:179–187.
- Bevan RD, Tsuru H. 1981. Functional and structural changes in the rabbit ear artery after sympathetic denervation. Circ Res. 49:478–485.
- Bryan RM, Marrelli SP, Steenberg ML, Schildmeyer LA, Johnson TD. 2001. Effects of luminal shear stress on cerebral arteries and arterioles. Am J Physiol Heart Circ Physiol. 280:H2011–H2022.
- Busija DW, Heistad DD, Marcus ML. 1980. Effects of sympathetic nerves on cerebral vessels during acute, moderate increases in arterial pressure in dogs and cats. Circ Res. 46:696–702.
- Cassaglia PA, Griffiths RI, Walker AM. 2008. Sympathetic nerve activity in the superior cervical ganglia increases in response to imposed increases in arterial pressure. Am J Physiol Regul Integr Comp Physiol. 294:R1255–R1261.
- Cassaglia PA, Griffiths RI, Walker AM. 2009. Cerebral sympathetic nerve activity has a major regulatory role in the cerebral circulation in REM sleep. J Appl Physiol. 106:1050–1056.
- Gu WG, Jiang W, Brännström T, Wester P. 1999. Long-term cortical CBF recording by laser-Doppler flowmetry in awake freely moving rats subjected to reversible photothrombotic stroke. J Neurosci Methods. 90:23–32.
- Handa Y, Nojyo Y, Hayashi M. 1991. Patterns of reinnervation of denervated cerebral arteries by sympathetic nerve fibers after unilateral ganglionectomy in rats. Exp Brain Res. 86:82–89.
- Haywood JR, Shaffer RA, Fastenow C, Fink GD, Brody MJ. 1981. Regional blood flow measurement with pulsed Doppler flowmeter in conscious rat. Am J Physiol. 241:H273–H278.
- Julien C, Cerutti C, Kandza P, Barrès C, Su D, Vincent M, Sassard J. 1988. Cardiovascular response to emotional stress and spontaneous blood pressure variability in genetically hypertensive rats of the Lyon strain. Clin Exp Pharmacol Physiol. 15:533–538.
- Kobayashi S, Tsukahara S, Tsuji T, Sugita K, Nagata T. 1983. Histochemical studies on the regeneration of aminergic nerves in rat cerebral artery after superior cervical ganglionectomy. Histochemistry. 77:57–62.
- Kolb B, Rotella DL, Stauss HM. 2007. Frequency response characteristics of cerebral blood flow autoregulation in rats. Am J Physiol Heart Circ Physiol. 292:H432–H438.
- Liu AJ, Ma XJ, Shen FM, Liu JG, Chen H, Su DF. 2007. Arterial baroreflex: A novel target for preventing stroke in rat hypertension. Stroke. 38:1916–1923.
- Loos N, Grant DA, Wild J, Paul S, Barfield C, Zoccoli G, Franzini C, Walker AM. 2005. Sympathetic nervous control of the cerebral circulation in sleep. J Sleep Res. 14:275–283.
- Nafz B, Wagner CD, Persson PB. 1997. Endogenous nitric oxide buffers blood pressure variability between 0.2 and 0.6 Hz in the conscious rat. Am J Physiol. 272:H632–H637.
- Paravicini TM, Miller AA, Drummond GR, Sobey CG. 2006. Flow-induced cerebral vasodilatation in vivo involves activation of phosphatidylinositol-3 kinase, NADPH-oxidase, and nitric oxide synthase. J Cereb Blood Flow Metab. 26:836–845.
- Rothwell PM, Howard SC, Dolan E, O'Brien E, Dobson JE, Dahlöf B, Sever PS, Poulter NR. 2010. Prognostic significance of visit-to-visit variability, maximum systolic blood pressure, and episodic hypertension. Lancet. 375:895–905.
- Sadoshima S, Busija DW, Heistad DD. 1983. Mechanisms of protection against stroke in stroke-prone spontaneously hypertensive rats. Am J Physiol. 244:H406–H412.
- Yu YH, Blessing WW. 1997. Acute increases in forebrain blood flow during alerting responses in conscious rabbits. Brain Res. 767:1–7.
- Zhang ZQ, Julien C, Barrès C. 1996. Baroreceptor modulation of regional haemodynamic responses to acute stress in rat. J Auton Nerv Syst. 60:23–30.