Abstract
Experimental and clinical evidence has shown that chronic stress plays an important role in the onset and/or exacerbation of symptoms of functional gastrointestinal disorders. Here, we aimed to investigate whether exposure to a chronic and temporally unpredictable psychosocial stressor alters visceral and somatic nociception as well as anxiety-related behaviour. In male C57BL/6J mice, chronic stress was induced by repeated exposure to social defeat (SD, 2 h) and overcrowding (OC, 24 h) during 19 consecutive days. Visceral and somatic nociception was evaluated by colorectal distension and a hot plate, respectively. The social interaction test was used to assess social anxiety. Mice exposed to psychosocial stress developed visceral hyperalgesia and somatic hypoalgesia 24 h following the last stress session. SD/OC mice also exhibited social anxiety-like behaviour. All these changes were also associated with physiological alterations, measured as a decreased faecal pellet output and hypothalamic-pituitary-adrenal (HPA) axis disruption. Taken together, these data confirm that this mouse model of chronic psychosocial stress may be useful for studies on the pathophysiological mechanisms underlying such stress-associated disorders and to further test potential therapies.
Introduction
Visceral hyperalgesia is a poorly understood symptom which is common in functional bowel disorders such as irritable bowel syndrome (IBS). IBS is a frequent and debilitating disorder of the gastrointestinal tract (GIT) characterized by the presence of recurrent chronic abdominal pain and defecation-related symptoms (Spinelli Citation2007). Our understanding of the pathophysiology of IBS remains incomplete despite its large socio-economic burden. It is currently viewed as a disorder of the brain–gut axis, referred to as the bi-directional communication pathways between the central nervous system and the GIT (Jones et al. Citation2006; Mayer and Tillisch Citation2011). Alterations in peripheral and central pathways of the brain–gut axis are likely to underlie visceral hyperalgesia and associated gastrointestinal dysfunction (Mayer et al. Citation2008).
Stress is well known to play a key role in the pathogenesis of IBS by disrupting the brain–gut axis (Mayer Citation2000; Elsenbruch et al. Citation2010; Cryan and O'Mahony Citation2011; O'Malley et al. Citation2011). In fact, epidemiological studies provide evidence for the pathophysiological relevance of stress as a trigger of first onset and/or exacerbation of IBS symptoms in which visceral hypersensitivity is an important hallmark (Drossman et al. Citation2002; Mayer and Tillisch Citation2011). Social stress derived from economic and social pressures in everyday life is one of the main and potent sources of stress in humans (Huhman Citation2006). Indeed, the prominent impact of social stress is further supported by several reports that have highlighted how chronic exposure to psychosocial stressors in the form of sustained, threatening life events can influence the manifestation of IBS symptomatology (Bennett et al. Citation1998; Choung et al. Citation2009; Lackner et al. Citation2010). It should be noted that social stress is also considered to predispose to the pathogenesis of psychiatric disorders including depression and anxiety (McEwen Citation2004; Gros et al. Citation2009). Thus, the conceptualization of IBS as a stress disorder is becoming particularly compelling due to its comorbidity with other chronic stress-related psychiatric disorders (Drossman et al. Citation2002; Elsenbruch et al. Citation2010).
Animal models are essential to better understand the pathophysiology of IBS (Mayer et al. Citation2008). Additionally, due to the increase in the use of genetically modified mice, the need for developing mouse models of disease has intensified (Cryan and Holmes Citation2005). Animal models of stress-induced visceral hypersensitivity have used neonatal maternal separation stress in rats (Coutinho et al. Citation2002; Barreau et al. Citation2004; Gosselin et al. Citation2010; O'Mahony et al. Citation2011) and acute (Schwetz et al. Citation2004) and chronic water avoidance stress in adult animals. Models in rats (Bradesi et al. Citation2005; Yu et al. Citation2010) and in mice (Larsson et al. Citation2009; Larauche et al. Citation2010) have been well established in an attempt to achieve a better understanding of the pathophysiological mechanisms underlying visceral hyperalgesia. Although these models of stress present good face and construct validity, a frequent criticism argues against their predictive validity with regard to efficacy of treatment strategies in humans, which is either unknown or has been disappointing (Holschneider et al. Citation2011). One plausible explanation for this may be that most of the laboratory stressors used to date do not reflect adequately the sources of stress present in the natural habitat and thus fail to resemble the challenges that mammals face within their natural environment (Koolhaas et al. Citation1997; Tamashiro et al. Citation2005). However, animal models of chronic social stress closely represent the type and intensity of stressors experienced by humans and rodents on a daily basis under normal living conditions (Tamashiro et al. Citation2005; Huhman Citation2006). Recently, a report has been published indicating the development of visceral hyperalgesia associated with colonic dysfunction in response to a model of chronic psychosocial stress in rats (Vicario et al. Citation2011).
However, to our knowledge, no studies exist on the effect of unpredictable and intermittent chronic psychosocial stress in adult mice on visceral nociception. Moreover, Reber and colleagues (Citation2006) have shown that this model of chronic psychosocial stress robustly enhances gastrointestinal dysfunction in a mouse model of colitis. Also, we have recently described the ability of this model of chronic stress to induce anxiety- and depression-related behaviours in mice (Finger et al. Citation2011a) indicating that this model of stress may have multiple effects across the brain–gut axis. To this end, the present study extends these findings by aiming to test the hypothesis that a model of chronic and temporally unpredictable intermittent psychosocial stress [social defeat (SD) and overcrowding (OC)] in mice modulates visceral and somatic sensitivity and social anxiety-like behaviour.
Materials and methods
Animals
Adult male C57BL/6J mice (8 weeks of age, experimental mice) and adult male CD1 mice (9–10 weeks of age, used as resident aggressors) were purchased from Harlan (Bicester, UK). All mice were kept under standard controlled conditions (21 ± 1°C, 55 ± 10% humidity), on a 12-h light/dark cycle (lights on at 07:00 h). Food and water were provided ad libitum. Mice were singly housed (stress procedure) or group housed as 3–4 mice per cage in standard mouse cages (33 × 15 × 13 cm) for at least 2 weeks before the start of the experiment.
All animal care and experimental procedures were carried out in accordance with the European Community Council Directive (86/609/EEC) and approved by the Animal Experimentation and Ethics Committee of University College Cork. Every effort was made to minimize the number of animals used and their suffering.
Colorectal distension validation
CRD protocol
The procedure for colorectal distension (CRD) was adapted from previously published protocols by Martinez and colleagues (Arvidsson et al. Citation2006; Martinez and Melgar Citation2008). Mice were lightly anesthetized with isoflurane (2% vapour in oxygen; IsoFlo®, Abbott, UK) and a balloon with a connecting catheter was inserted into the colon, 0.5 cm proximal to the anus. Unrestrained mice were allowed to recover for 10 min before starting the CRD procedure. At the end of the experiments, the balloon was carefully removed and the animals were returned to their home cages.
CRD produces contractions of the abdominal musculature, termed the visceromotor response (VMR). From previous reports in rats and mice, pressure oscillations during the inflation of the intracolonic balloon are closely related to the presence of abdominal muscle contractions associated with CRD and, therefore, intra-balloon pressure changes are a valid method to assess the VMR associated with the CRD (Tammpere et al. Citation2005; Arvidsson et al. Citation2006). Thus, VMR to distension was quantified as pressure changes observed within the colonic distending balloon during the CRD procedure.
The CRD system consisted of a barostat (Distender Series II, G&J Electronics, Toronto, ON, Canada) and a transducer amplifier (LabTrax 4, World Precision Instruments, Sarasota, FL, USA). The barostat was used to control air inflation and pressure during the CRD procedure and Protocol PlusTM software (G&J Electronics, Toronto, ON, Canada) was used to control the barostat. A custom-made balloon (2 cm length × 1 cm inflated diameter) prepared from a polyurethane plastic bag (GMC Medical, Hornslet, Denmark) was tied over a PE60 catheter with 4.0 silk suture. Before securing the balloon to the catheter, several holes were punched in the distal 20 mm of the tubing with a 27-gauge needle to allow the balloon to inflate.
On the day of the experiment, CRD was performed starting at 08:00 h. As above, the mice were lightly anesthetized with isoflurane (2% vapour in oxygen) and a balloon with a connecting catheter was inserted into the colon, 0.5 cm proximal to the anus. The catheter was fixed to the base of the tail with tape to avoid any displacement. The balloon was connected to the barostat system and subsequent pressure changes within the distending balloon, observed in response to a distension paradigm, were monitored and recorded using DataTrax 2 software (World Precision Instruments, Sarasota, FL, USA). Two CRD paradigms were used: (1) ascending phasic distension from 10 to 80 mmHg, consisting of three 20-s pulses at each pressure and 5-min inter-pulse intervals and (2) repeated phasic distension at 55 mmHg, consisting of twelve 20-s pulses and 5-min inter-pulse intervals. Similar CRD protocols have previously been used to assess the VMR to CRD in mice (Arvidsson et al. Citation2006; Martinez and Melgar Citation2008).
The analogue input channels were sampled with individual sampling rates and digital filtering was performed on the signals. The pressure signals were sampled at 50 Hz and a high-pass filter at 1 Hz was used to separate the contraction-induced pressure changes from the low varying pressure generated by the barostat. Data analysis of the recordings was performed as follows. DataTrax2 software was used to quantify the magnitude of the balloon pressure signals. Changes in high-pass-filtered balloon pressure signals were determined for the 5-s period before and after each pulse (baseline activity) and over pulses. However, the first and the last second of each pulse were excluded since they reflect artefact signals produced by the barostat during the inflation and deflation of the balloon and do not originate from the animal. Because of the previously described bi-phasic responses to CRD in mice (Arvidsson et al. Citation2006), all data reported correspond to the first 5-s period of the pulses, which usually covers the majority of the VMR to the distension. In all cases, and to minimize variability due to movement-related artefacts, VMR to CRD were established for each three consecutive pulses as a group. For every mouse, the pain threshold was defined as the pressure of the distending pulse at which the response evoked exceeded the mean baseline activity plus three times the SD (Larsson et al. Citation2009).
Drugs
The muscarinic antagonist, atropine and the μ-opioid receptor agonist, fentanyl (Sigma-Aldrich, Wicklow, Ireland) were dissolved in phosphate-buffered saline (PBS) 1 × solution to the desired concentration and tested in naive mice. These pharmacological studies were important to conduct as they provided a validation of the CRD procedure in our laboratory and were based on previous studies (Arvidsson et al. Citation2006; Martinez and Melgar Citation2008).
The dose of atropine used was based on studies showing that a similar regimen of administration does not influence the CRD-elicited intra-balloon pressure changes (Arvidsson et al. Citation2006; Larauche et al. Citation2010). The dose of fentanyl was chosen based on studies showing that fentanyl completely prevents the hyperalgesic response elicited by repetitive CRD (Larsson et al. Citation2003; Arvidsson et al. Citation2006).
The repeated phasic distension paradigm (12 × 55 mmHg) was used with the first three distensions serving as control. After the third distension, atropine (1 mg/kg), fentanyl (0.05 mg/kg) or vehicle (PBS 1 × , 5 ml/kg) were administered subcutaneously and the response to CRD was assessed for the following nine distensions.
Effects of chronic psychosocial stress on nociception and social avoidance behaviour
Experimental design
Mice were stressed according to an unpredictable mixed schedule of SD and OC sessions over 19 days (control: n = 38; stress: n = 37) as illustrated in . On Day 20, following the last stress session (OC 24 h) mice underwent CRD, a hot plate (HP) test and a social interaction test (SIT). All behavioural tests were carried out in separated cohorts of mice during the light cycle (between 08:00 and 14:00 h). Additionally, 24 h after the last stress session, a separate group of stressed and control mice (without undergoing behavioural testing) was euthanized at the beginning of the dark cycle (20:00 h) to confirm the reliability of this model of chronic stress in mice in terms of chronic stress-induced physiological markers (thymus weight, adrenal weight and plasma corticosterone concentrations).
Figure 1. Timeline of the experimental procedure. A variable chronic social stress (social defeat and overcrowding; SD/OC) paradigm was applied for 19 days. On Day 20, to assess the effect of chronic psychosocial stress on visceral and somatic nociception, mice were tested with CRD (with ascending distension from 10 to 80 mmHg), and the HP test, respectively. To evaluate the impact of psychosocial stress on social interaction or avoidance behaviour, 24 h after the last stress session, mice underwent the Social Interaction Test (SIT) followed by faecal pellets collection. Separate cohorts of mice were used for each behavioural test to avoid additional confounding factors. To study the effect of SD/OC on body weight, mice were weighed on days 1, 3, 8, 10, 15 and 19, before each stress session (indicated by arrows). Additionally, some control and SD/OC mice were killed at the beginning of the dark phase (20:00 h) to determine the effect of psychosocial stress on thymus weight, adrenal weight and plasma corticosterone concentrations.
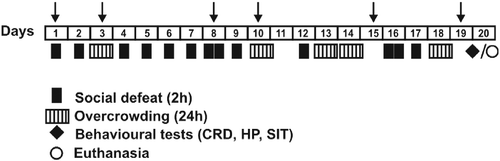
Chronic psychosocial stress: SD and OC
The procedure for SD and OC was performed essentially as described previously (Reber et al. Citation2006; Finger et al. Citation2011a,Citationb). C57BL/6J mice were singly housed and randomly assigned to the control or the stress (SD/OC) group. CD1 mice were used as resident aggressors. Prior to the SD/OC procedure, CD1 mice were exposed over three consecutive days to a test C57BL/6J mouse until the first attack followed by submissive behaviour of the defeated mouse occurred (Beitia et al. Citation2005; Berton et al. Citation2006). CD1 mice with consistent attack latencies ( ≤ 30 s) were selected for the SD procedure. Control mice remained undisturbed except for change of bedding once a week.
Briefly, SD/OC mice were exposed to unpredictable sessions of SD (once or twice a day) and OC (24 or 48 h) over 19 days (see timeline, ). The last stress session was in all the cases of the same type, an OC (24 h) session.
SD sessions consisted of placing SD/OC mice into the home cage of an unfamiliar resident. Thereafter, interaction was allowed and when the first attack of the aggressor and a submissive behaviour of the stress mouse were observed, the mice were separated by a perforated plexiglass wall, allowing visual, olfactory and auditory contact for 2 h. After this period, the separator was removed to follow another attack and defeat. Then, SD/OC mice were returned to their home cage. It is important to note that no SD/OC mouse was ever exposed to the same resident aggressor CD1 mouse over the SD sessions more than once. So, SD/OC mice were exposed to a new resident aggressor CD1 mouse for every SD session. OC sessions consisted of placing 8–10 SD/OC mice in a plastic cage (33 × 15 × 13 cm) for 24 or 48 h, with the same mice housed together over all the OC sessions, with water and food available ad libitum. All SD/OC mice underwent an OC (24 h) session as a last stress (as indicated in ) before performing the subsequent behavioural task.
Colorectal distension
On the day of the experiment, 24 h following the last stress session (), CRD was performed as described above. The ascending phasic distension paradigm (from 10 to 80 mmHg) was used to assess the VMR in a separated cohort of control and SD/OC mice.
Hot plate test
On Day 20, individuals from a separate group of mice that experienced the chronic stress protocol and controls were placed on a HP (Incremental Hot Plate, Stoelting, Ireland) heated to 50°C and the latency to jump was measured. The cut-off time was set at 120 s to avoid tissue damage (Tramullas et al. Citation2010).
Social interaction test
Deficits in social interaction have been one of the most robust manifestations of chronic SD-induced anxiety in rodents (File and Seth Citation2003). Hence, 24 h after the last stress session, a two-trial SIT was used to evaluate social interaction/avoidance behaviour towards an unfamiliar aggressor used during the SD/OC procedure as described previously (Berton et al. Citation2006; Finger et al. Citation2011a,Citationb).
In the first 2.5-min trial (‘no target’), the mouse was placed into a plastic box (39 × 31 × 24 cm) containing an empty wire mesh cage (9.5 × 7.5 × 7.0 cm) apposed to one side and allowed to explore freely. The mouse was then removed and transferred back to its home cage for 1 min. For the second 2.5-min trial (‘target’), the mouse was placed back into the box now containing within the wire mesh cage an unfamiliar aggressor CD1 mouse that had previously been used as resident aggressor over the stress procedure with another SD/OC mouse. The test mouse was allowed to freely explore for another 2.5 min. At the end of the test, both mice were returned to their home cages and the arena and mesh cage were cleaned with 70% ethanol. All testing was carried out between 10:00 and 12:00 h under red light to reduce interference of potential anxiogenic factors with social interaction behaviour. Trials were recorded by a camera mounted on the ceiling above the box and were tracked and evaluated using Ethovision 3.1 (Noldus, Wageningen, Netherlands). The time spent in the ‘interaction zone’ around the wire mesh cage during the first and the second trial was scored and the interaction ratio was calculated as the ratio of the time in the interaction zone in the target condition over the no-target condition (expressed as a percentage).
Faecal pellet output
Twenty-four hours after the last stress session, the colonic motor function in response to chronic psychosocial stress was evaluated by quantifying the faecal pellet output over the 5-min trial SIT.
Corticosterone assay
Mice were taken from their cage and rapidly euthanized by decapitation. No anaesthesia was used to prevent interference with the veracity of the biological samples collected. Trunk blood was collected in EDTA-coated 1.5-ml tubes and placed on ice and centrifuged at 745g for 10 min at 4°C; plasma was collected and stored at − 80°C until assayed. Plasma corticosterone concentrations were measured using commercially available Enzyme Immunoassay Kits (Assay Designs, Inc., Ann Arbor, MI, USA) according to the manufacturer's instructions. The sensitivity of detection was < 26.99 pg/ml and intra- and inter-assay variability were 8.4% and 8.2%, respectively.
Statistical analysis
Data are expressed as mean ± SEM. Changes in response to CRD and body weight were analysed by repeated measures one-way ANOVA (RM-ANOVA) followed by the estimation of parameters. For all other experiments, mean values were compared using an independent sample t-test. The VMR was calculated as the increase in the area under the curve over the distension and in some cases, data represent the cumulative response (expressed as percentage of the control) during the CRD procedure. All tests were carried out at a significance level of p < 0.05.
Results
Effects of atropine and fentanyl on the VMR to CRD
The CRD procedure performed in the present study was adapted from previously published protocols, in order to verify in our laboratory that pressure changes observed in the balloon were not affected by colonic wall motility per se and to detect the effects of analgesic drugs on visceral pain modulation. The muscarinic receptor antagonist, atropine and the μ-opioid receptor agonist, fentanyl were tested on the repeated phasic distension paradigm (12 × 55 mm/Hg) of CRD.
Atropine (1 mg/kg, s.c.) did not affect the VMR compared with the vehicle-treated group (treatment: p = 0.097; distension × treatment: p = 0.963, N = 7; ). Also, as shown in , vehicle-treated mice exhibited a significant increase in the VMR over distensions, while fentanyl (0.05 mg/kg, s.c.) completely prevented the sensitizing response associated with repetitive distensions [treatment: F(1,14) = 11.9; p < 0.01; distension × treatment: F(3,42) = 3.7; p < 0.05, N = 7–10], consistent with visceral analgesia. There were no significant differences in the basal activity over distensions either between groups or within the same experimental group.
Figure 2. Effect of atropine and fentanyl on the VMR to repeated CRD. (A) The muscarinic antagonist atropine did not alter the VMR (expressed as pressure changes in the balloon in response to CRD) and (B) the μ-opioid receptor agonist fentanyl prevented the sensitizing response to repeated CRD (12 × 55 mmHg). After a first group of three distensions, vehicle, atropine (1 mg/kg) or fentanyl (0.05 mg/kg) were administered subcutaneously (indicated by the arrow) and nine additional distensions were performed. The broken lines represent the basal activity. N = 7–10 mice per group. Data are expressed as mean ± SEM. *p < 0.05 and ***p < 0.001 vs. control mice (RM-ANOVA).
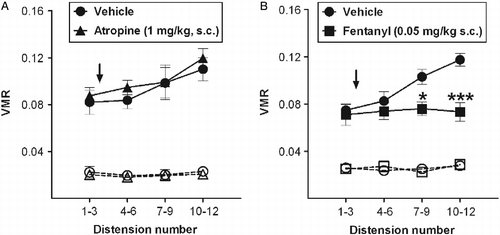
Chronic psychosocial stress (SD/OC)-induced visceral hypersensitivity
The VMR during ascending phasic distension (10–80 mmHg) was recorded 24 h after the last stress session. RM-ANOVA revealed no significant differences in the basal activity (between distensions) of the abdominal musculature over pressure either between groups [F(1,22) = 0.5; p = 0.478, N = 12] or within the same experimental group [F(4,88) = 1.1; p = 0.382]. All mice displayed a progressive and significant increase in the visceral nociceptive responses with increased pressure [F(4,84) = 41.4; p < 0.001]. However, SD/OC mice were significantly more sensitive to CRD compared with control mice [stress: F(1,21) = 43.2; p < 0.001]. In addition, they exhibited significantly greater VMR over pressure [pressure × stress: F(4,84) = 4.9; p < 0.001; , which reached significance at pressures of 25, 40, 65 and 80 mmHg consistent with visceral hyperalgesia. When the cumulative response to CRD was expressed as percentage change in the control group (set as 100%), SD/OC mice exhibited a significant increase in the overall response to CRD (t = 5.5; p < 0.001; ). With regard to the pressure threshold for visceral pain responses, SD/OC mice showed a significantly lower pain threshold compared with control mice (t = 2.8; p < 0.01; ). All these data are consistent with visceral hyperalgesia following chronic psychosocial stress.
Figure 3. Chronic psychosocial stress alters the visceral sensitivity to CRD. (A) Chronic SD/OC induced increased visceral pain responses 24 h following the last stress session. The increase in the VMR (expressed as pressure changes in the balloon in response to CRD) in the stress group was significant for distension pressures of 25, 40, 65 and 80 mmHg, compared with the control group. The broken lines represent the basal activity. (B) Overall response to CRD. Values are expressed as percentage change of control group (set as 100%). SD/OC mice showed a significant increase in the overall response to CRD when compared with the control group. (C) Pain threshold to CRD. SD/OC mice exhibited a significantly lower pain threshold to CRD, compared with the control group. N = 12 mice per group. Data are expressed as mean ± SEM. *p < 0.05, **p < 0.01 and ***p < 0.001 vs. control mice (two-tailed Student's t-test).
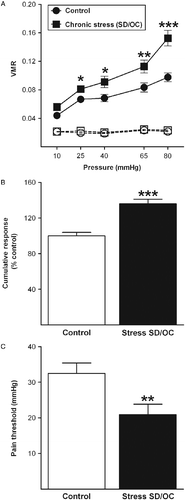
Chronic psychosocial stress (SD/OC)-induced somatic analgesia
To further evaluate whether the chronic psychosocial stress paradigm resulted in a similar sustained somatic pain modulation, the HP test was performed 24 h after the last stress session. This test examines responses to a noxious thermal stimulus mediated by spinal and supraspinal mechanisms (Tramullas et al. Citation2010). The HP test was carried out in a separate cohort of mice to avoid possible interactions between somatic and visceral nociceptive responses. SD/OC mice displayed a significant increase in the latency to jump when compared with control mice (control: 37.2 ± 6.8 vs. stress: 64.6 ± 9.7 s, t = 2.4; p < 0.05, N = 12) indicating somatic hypoalgesia 24 h following the last stress session.
Chronic psychosocial stress (SD/OC)-induced social anxiety-like behaviour
SIT was performed 24 h after the last stress session to determine whether SD/OC alters the motivation for social interaction in mice triggering social avoidance behaviour. SD/OC mice elicited a significant increase in social avoidance behaviours as reflected by a decrease in the interaction ratio when compared with the control group (control: 130.2 ± 10.3% vs. stress: 24.6 ± 7.9%, t = 8.0, p < 0.001, N = 13–14). The interaction ratio was defined as the percentage of time that the mouse was in the interaction zone in the presence versus absence of the ‘social target’ (unfamiliar aggressor CD1 mouse). All mice spent an equivalent amount of time in the interaction zone [in the absence of an unfamiliar aggressive CD1 mouse (‘no target’), t = 0.2, p = 0.841; data not shown], indicating that SD/OC did not affect general exploratory activity. Also, no difference in the total distance moved in the arena was observed between groups (‘no target’: t = 1.3, p = 0.196; ‘target’ t = 1.5, p = 0.128; data not shown), indicating no effect of SD/OC on locomotor activity.
Chronic psychosocial stress (SD/OC)-induced physiological changes
Faecal pellet output
In response to stress, faecal pellet output ia a validated and quantitative parameter indicating colonic motor function. SD/OC mice showed a significantly lower number of faecal pellets (t = 3.8, p < 0.001, N = 13–14) over the 5-min trial of the SIT, 24 h after the last stress session, exhibiting a constipation-like phenotype ().
Figure 4. Chronic stress-induced physiological changes. Mice subjected to chronic SD and OC showed a significant decrease in the number of faecal pellets, 24 h following the last stress session. N = 13–14 mice per group. (B) Daily body weight changes. SD/OC mice showed a significant increase in body weight over days compared with control mice. (C) Body weight gain. SD/OC mice gained significantly more body weight by the end of the SD/OC procedure (calculated as the difference between Day 19 and Day 1). N = 37–38 mice per group. (D,E) Relative thymus and adrenal weights [expressed as tissue weight (mg) per body weight (g)]. SD/OC mice show significantly reduced thymus weight (D) and increased weight of the adrenals (E). (F) Dark-phase plasma corticosterone concentrations. SD/OC mice showed significantly lower levels of plasma corticosterone than the control group. N = 14 mice per group. Data are expressed as mean ± SEM. *p < 0.05 and ***p < 0.001 vs. control mice (two-tailed Student's t-test).
![Figure 4. Chronic stress-induced physiological changes. Mice subjected to chronic SD and OC showed a significant decrease in the number of faecal pellets, 24 h following the last stress session. N = 13–14 mice per group. (B) Daily body weight changes. SD/OC mice showed a significant increase in body weight over days compared with control mice. (C) Body weight gain. SD/OC mice gained significantly more body weight by the end of the SD/OC procedure (calculated as the difference between Day 19 and Day 1). N = 37–38 mice per group. (D,E) Relative thymus and adrenal weights [expressed as tissue weight (mg) per body weight (g)]. SD/OC mice show significantly reduced thymus weight (D) and increased weight of the adrenals (E). (F) Dark-phase plasma corticosterone concentrations. SD/OC mice showed significantly lower levels of plasma corticosterone than the control group. N = 14 mice per group. Data are expressed as mean ± SEM. *p < 0.05 and ***p < 0.001 vs. control mice (two-tailed Student's t-test).](/cms/asset/99a204cb-f0bf-4588-94b2-dfba370fa207/ists_a_622816_f0004_b.gif)
Body weight
Over 19 days, all mice showed a significant increase in body weight [day: F(5,365) = 117.6; p < 0.001]. However, the increase in body weight over days was significantly higher in mice exposed to stress [stress × day: F(5,365) = 21.6; p < 0.001; . As shown in , SD/OC mice had gained significantly more body weight (t = 5.4; p < 0.001, N = 37–38) by the end of the SD/OC procedure (calculated as the difference between Day 19 and Day 1).
Thymus and adrenal weight and plasma corticosterone levels
Twenty-four hours after the last stress session, a separate group of stress and control mice (without experiencing behavioural testing) was euthanized at the beginning of the dark cycle (20:00 h) and the thymus and the adrenal glands were removed and weighed. SD/OC mice showed a significant decrease in thymus weight (t = 7.1; p < 0.001, N = 14; ) and a significant increase in adrenal weight (t = 5.3; p < 0.001, N = 14; ) when compared with control mice. Moreover, plasma corticosterone concentration was significantly decreased (t = 2.5; p < 0.05, N = 14; ) in SD/OC mice compared with the control group.
Discussion
In this study, we demonstrate, for what is to our knowledge the first time, the development of visceral hyperalgesia following chronic psychosocial stress in adult mice. Interestingly, this increase in visceral sensitivity was observed in concert with somatic hypoalgesia in SD/OC mice. Moreover, alterations in nociception following the repeated stressor were associated with enhanced social anxiety-related behaviour, decreased colonic motility, as well as physiological alterations and hypothalamic-pituitary-adrenal (HPA) axis disruption.
It is well known that everyday life stress can induce physiological and psychological disturbances triggering the onset or symptom exacerbation in functional gastrointestinal disorders (FGIDs) such as IBS (Bennett et al. Citation1998; Choung et al. Citation2009; Lackner et al. Citation2010). Thus, animal models of chronic psychosocial stress may better represent the type and intensity of stressors experienced by humans on a daily basis. For this purpose, we conducted the current study by using a model of chronic psychosocial stress based on the combination of two types of stressors, SD and OC. SD is caused by inter-male confrontations to establish a territory and its defence against unfamiliar intruders. OC involves the establishment and maintenance of social hierarchy and competition for resources (space, food and water; Koolhaas et al. Citation1997; Tamashiro et al. Citation2005). Despite it being well recognized that the prevalence of IBS is much higher in women (Ouyang and Wrzos Citation2006), it should be noted that the use of these rodent models of psychosocial stress seems to be ineffective in females, as it is generally difficult to induce high levels of aggression or to obtain a strong dominance relationship in normal females (Martinez et al. Citation1998).
The stressful nature of this model of chronic psychosocial stress was first demonstrated by the ability to modulate visceral and somatic pain perception in mice. Hence, visceral hyperalgesia was observed 24 h following the last stress session, by monitoring the VMR to CRD via recording the abdominal contractions in conscious and unrestrained mice by manometry. Additionally, SD/OC mice showed a lower pain pressure threshold to CRD. Also, the pain threshold in the control group (about 32 mmHg) was consistent with previous reports (Arvidsson et al. Citation2006; Larauche et al. Citation2010).
In our study, visceral hyperalgesia observed in SD/OC mice was related to the combination of SD and OC sessions per se. A non-invasive, surgery-free method to assess visceral sensitivity was performed (Arvidsson et al. Citation2006), which we first validated pharmacologically. The results of the CRD procedure used in the current study were unaffected by contractions originating from the smooth muscle of the colon contributing to the measured balloon pressure changes as the muscarinic receptor antagonist, atropine, which has been extensively used to inhibit colonic motility in mice (Arvidsson et al. Citation2006), rats (Tammpere et al. Citation2005) and humans (O'Brien et al. Citation1997), did not affect the repeated phasic CRD-associated balloon pressure changes. Moreover, the paradigm was sensitive to the analgesic effects of the μ-opioid receptor agonist fentanyl. Fentanyl inhibited visceral sensitization in response to repeated phasic CRD, consistent with previous reports (Larsson et al. Citation2003; Arvidsson et al. Citation2006).
There have been some previous studies investigating the effects of repeated predictable water avoidance stress in mice and these have largely given rise to inconsistent results. Water avoidance stress has been described as inducing visceral hyperalgesia and visceral hypoalgesia (Larauche et al. Citation2010) or as failing to change the perception of visceral pain (Larsson et al. Citation2009). These different observations are considered to be due to differences in housing conditions, gender, surgery associated with electrode implantation and the method used to record the VMR. Consequently, to rule out the impact of single housing in visceral pain, we have also studied a separate cohort of group-housed mice that underwent CRD. The control group (singly housed) did not show alterations in visceral sensitivity when compared with group-housed mice (data not shown).
Differences in visceral pain responses found between our data and those generated using water avoidance stress in mice (Larsson et al. Citation2009; Larauche et al. Citation2010) can be explained in terms of the predictability, type of stressor (psychosocial vs. physiological), severity (combination of two types of stressor against one type) and duration of the stressor (19 days against 10 days), since visceral pain modulation in response to stress is affected by all of these factors (Terman et al. Citation1984). The water avoidance stress paradigm has been demonstrated to generate in animals, especially rats, many of the proposed functional and psychological alterations observed in FGIDs. However, the successful translation of this model to mice has not been consistent with regard to visceral pain. The reasons for this, in addition to those mentioned above, may be related to the inter-species vulnerability to cope with stress, as mice are more resistant to stress than rats (Savignac et al. Citation2011a), at least in relation to visceral pain modulation (Larauche et al. Citation2011). Moreover, the intensity of the stressor (a combination of type and duration) is a key factor that must be taken into consideration for effectiveness in triggering stress-induced visceral hyperalgesia.
Nociception is a complex phenomenon that is undoubtedly modulated by stress (Ossipov et al. Citation2010) and social stimuli have long been known to elicit stress-induced somatic analgesia in mice (Miczek et al. Citation1982). Our findings of concomitant somatic hypoalgesia in chronic SD/OC mice are consistent with previous reports showing that repeated SD stress induced somatic analgesia (Miczek et al. Citation1982). In addition, other stressors have also been used to produce stress-induced somatic analgesia in mice, such as repeated forced swimming, exposure to a natural predator, footshock-witness test, chronic mild stress (swim stress) and chronic exposure to negative psycho-emotional conditions (Butler and Finn Citation2009). Even though stress-induced somatic analgesia has been far more extensively studied, stress has also been associated with somatic hyperalgesia (Imbe et al. Citation2006). However, taking into consideration the severity of the stressor in the modulation of pain sensitivity, it has been proposed that more severe stressors evoke stress-induced somatic analgesia, whereas less severe stressors evoke stress-induced somatic hyperalgesia (Vidal and Jacob Citation1982). Thus, these different observations may be explained in terms of type, severity and duration of the stressor. Even so, our data demonstrate a differential profile for the impact of chronic psychosocial stress on visceral and somatic nociception. This difference suggests the activation of different sensitization mechanisms that are specifically modulating visceral and somatic nociception, rather than a generalized hyperalgesia in response to stress. Similarly, alterations in stress-induced modulation of viscerosomatic sensitivity have been observed in several animal studies. In agreement with our data, visceral hyperalgesia combined with somatic hypoalgesia has been reported in two models of IBS in rats in response to acute (Coutinho et al. Citation2002) and chronic (Bradesi et al. Citation2005) psychological stress.
To further investigate the ability of the SD/OC stressor to induce psychological alterations, the SIT was performed 24 h following the last stress session. SD alters the motivation for social interaction in mice (Berton et al. Citation2006) and such deficits have been associated with anxiety- and depression-related behaviours (File and Seth Citation2003; Berton et al. Citation2006; Savignac et al. Citation2011b). Herein, we further found that alterations in visceral and somatic nociception observed following chronic psychosocial stress were also associated with social anxiety-like behaviour in the form of altered social interaction behaviour. Thus, chronic SD/OC mice exhibited increased social avoidance behaviour along with visceral hyperalgesia. These findings are in agreement with previous reports of anxiety-like behaviour in models of visceral hyperalgesia in rats (Coutinho et al. Citation2002; Bradesi et al. Citation2005; O'Mahony et al. Citation2011). In line with these results, our group has recently highlighted the ability of chronic psychosocial stress to induce a marked increase in anxiety- and depression-related behaviours. SD/OC mice exhibited a significant increase in the time spent in the dark compartment in the light–dark box and in the time of immobility in the forced swim test (Finger et al. Citation2011a). Therefore, the model of chronic intermittent psychosocial stress may be suitable for the study of FGIDs such as IBS, which in many cases is co-morbid with other chronic stress-related psychiatric disorders such as depression and anxiety (Gros et al. Citation2009). Also, future studies are required to investigate the potential long-lasting effects of this model of chronic psychosocial stress on visceral and somatic nociception as well as anxiety- and depression-related behaviours.
In addition to the above alterations induced by chronic stress, the effect of stress on colonic motility was evaluated by the measurement of faecal pellet output. We observed that chronic SD/OC decreased faecal pellet output, a constipation-related sign. Although stress has been more typically related to a diarrhoea phenotype due to enhanced colonic motility (Tache and Bonaz Citation2007) as shown by increased faecal pellet output (Melgar et al. Citation2008; Larsson et al. Citation2009; Larauche et al. Citation2010), based on our current observations chronic stress can also result in a decrease in colonic motility. Interestingly, these data are in agreement with recent studies in mice that underwent early-life stress that also displayed decreased faecal pellet output compared to control animals (Savignac et al. Citation2011a) and in BALB/c mice following a different SD stress protocol (Savignac et al. Citation2011b). It should be noted that the timepoint at which the number of faecal pellets was measured is an important consideration to be taken into account. Clearly, differences in the faecal pellet output as a measurement of colonic motor function emerge when it is performed 1 day or even weeks after the stress cessation compared to immediately after each session of stress (Melgar et al. Citation2008; Larsson et al. Citation2009; Larauche et al. Citation2010) due to adaptations in the colon in response to chronic stress. However, in these models of SD stress, more studies are warranted to address the colonic adaptation and underlying mechanisms following chronic stress, which result in decreased colonic motor function.
It is appropriate to consider whether stress-induced alterations in inflammatory processes may underlie the reduced colonic motility, hence resulting in a decreased defecatory response. Indeed, several models of chronic stress have been shown to induce spontaneous inflammation which persists for a few hours following the stress cessation (Bradesi et al. Citation2005; Reber et al. Citation2007; Vicario et al. Citation2011; Savignac et al. Citation2011c). However, chronic stress can also be associated with the reactivation of latent states of inflammation (Reber et al. Citation2006; Melgar et al. Citation2008). Changes in colon morphology and also reduction in colon length along with increase in colon weight when the colitis is quite severe have been extensively described as markers of gastrointestinal inflammation (Larsson et al. Citation2006; Reber et al. Citation2006; Melgar et al. Citation2008; Larsson et al. Citation2009). However, it should be noted that neither the visceral hyperalgesic effect nor the decrease in faecal pellet output observed in this study in response to chronic psychosocial stress was related to an appearance of spontaneous colitis or mild inflammation of the colon. Previous studies from our group showed no changes in colon length and weight following different chronic psychosocial stress protocols (Savignac et al. Citation2011c) and as expected, in the present study, colon length and colon weight (p < 0.05, data not shown) were found to be unaltered 24 h after the last stress session. Additionally, colon morphology scores in the SD/OC mouse model were normal with no overt structural abnormalities (Tramullas, Finger and Cryan, unpublished data). Such data are consistent with previous reports showing no clinical or histological signs of acute colitis after chronic stress (Larsson et al. Citation2006; Reber et al. Citation2006; Melgar et al. Citation2008; Larsson et al. Citation2009) and with those reports in which visceral hyperalgesia lasted up to 30 days after the end of the stress even when colon damage was absent (Bradesi et al. Citation2005; Vicario et al. Citation2011). Taken together, it is likely that, in the current model of chronic psychosocial stress, stress plays a role as a sensitization factor rather than as an inducer of inflammation per se (Reber et al. Citation2006).
Furthermore, several well-characterized chronic stress-induced physiological markers were evaluated, such as decrease in thymus weight and increase in adrenal weight. In our study, exposure to psychosocial stress triggered severe thymus atrophy and adrenal hypertrophy, indicating chronic stress and activation of the HPA axis, although plasma corticosterone concentration was decreased. This is in agreement with previous data in this model (Reber et al. Citation2006). These authors have shown that the lower levels of corticosterone during the dark phase in SD/OC mice reflected an adrenal insufficiency rather than HPA axis dysfunction. Additionally, we found that SD/OC mice gained more body weight than controls by the end of the SD and OC paradigm. Although different models of chronic stress in mice have been associated with either no changes (Melgar et al. Citation2008; Larsson et al. Citation2009; Larauche et al. Citation2010) or a reduction in body weight (Reber et al. Citation2006; Savignac et al. Citation2011c), recent evidence from models of chronic psychosocial stress indicate metabolic alterations in the direction of body weight gain (Moles et al. Citation2006; Bartolomucci et al. Citation2009) associated with hyperphagia and a positive energy balance.
Overall, our data further validate and expand the utility of the SD/OC paradigm that has been previously described to be a valid and relevant model of chronic psychosocial stress (Reber et al. Citation2006). These authors reported that chronic psychosocial stress increases the severity of dextran sodium sulphate-induced colitis and also impairs colonic regeneration after inflammation. Thus, this model of chronic psychosocial stress was chosen for its intensity and nature to better reflect the stress experienced by humans in their everyday lives and for its ability to recapitulate the psychosocial stress-induced alterations that may be contributory factors for the development of FGIDs (Choung et al. Citation2009; Elsenbruch et al. Citation2010; Lackner et al. Citation2010), such as reactivation of latent states of inflammation (Reber et al. Citation2006) and the development of anxiety- and depression-related behaviours (Finger et al. Citation2011a). From our study, we conclude that the SD/OC paradigm in mice is also poised to be an excellent model of visceral hypersensitivity and social anxiety-like behaviour, indicating that this model of psychosocial stress can have multiple effects on the brain–gut axis dysfunction.
In summary, this study provides the first evidence that chronic intermittent psychosocial stress has a modulatory impact on visceral and somatic nociception in adult mice. Thus, our data can be taken in the context of the development of a disease model of IBS, whereby chronic psychosocial stress in mice mimics some of the main clinical symptoms, such as alterations in visceral nociception and increased anxiety, seen in a large subset of IBS patients. Future studies are required to reveal the underlying mechanisms mediating such responses. Recently, an epigenetic model has been put forward for IBS (Dinan et al. Citation2010) and also chronic stress (Bradesi and Mayer Citation2009) and the role of such mechanisms in stress-induced visceral pain warrants further investigation.
Acknowledgements
The authors would like to thank Dr Stefan Reber and Dr David Slattery (University of Regensburg, Germany) for information and advice on the stress protocol and also Dr Beate Finger for her helpful comments on this paper and assistance in establishing the stress protocol. We thank Dr Marcela Julio-Piper and Mr. Patrick Fitzgerald for their technical assistance.
Declaration of interest: The authors report no conflicts of interest. The authors alone are responsible for the content and writing of the paper.
References
- Arvidsson S, Larsson M, Larsson H, Lindstrom E, Martinez V. 2006. Assessment of visceral pain-related pseudo-affective responses to colorectal distension in mice by intracolonic manometric recordings. J Pain. 7:108–118.
- Barreau F, Cartier C, Ferrier L, Fioramonti J, Bueno L. 2004. Nerve growth factor mediates alterations of colonic sensitivity and mucosal barrier induced by neonatal stress in rats. Gastroenterology. 127:524–534.
- Bartolomucci A, Cabassi A, Govoni P, Ceresini G, Cero C, Berra D, Dadomo H, Franceschini P, Dell'Omo G, Parmigiani S, Palanza P. 2009. Metabolic consequences and vulnerability to diet-induced obesity in male mice under chronic social stress. PLoS One. 4:e4331.
- Beitia G, Garmendia L, Azpiroz A, Vegas O, Brain PF, Arregi A. 2005. Time-dependent behavioral, neurochemical, and immune consequences of repeated experiences of social defeat stress in male mice and the ameliorative effects of fluoxetine. Brain Behav Immun. 19:530–539.
- Bennett EJ, Tennant CC, Piesse C, Badcock CA, Kellow JE. 1998. Level of chronic life stress predicts clinical outcome in irritable bowel syndrome. Gut. 43:256–261.
- Berton O, McClung CA, Dileone RJ, Krishnan V, Renthal W, Russo SJ, Graham D, Tsankova NM, Bolanos CA, Rios M, Monteggia LM, Self DW, Nestler EJ. 2006. Essential role of BDNF in the mesolimbic dopamine pathway in social defeat stress. Science. 311:864–868.
- Bradesi S, Mayer EA. 2009. Experimental models of stress and pain: do they help to develop new therapies?. Dig Dis. 27 Suppl. 1: 55–67.
- Bradesi S, Schwetz I, Ennes HS, Lamy CM, Ohning G, Fanselow M, Pothoulakis C, McRoberts JA, Mayer EA. 2005. Repeated exposure to water avoidance stress in rats: A new model for sustained visceral hyperalgesia. Am J Physiol Gastrointest Liver Physiol. 289:G42–G53.
- Butler RK, Finn DP. 2009. Stress-induced analgesia. Prog Neurobiol. 88:184–202.
- Choung RS, Locke GR3rd, Zinsmeister AR, Schleck CD, Talley NJ. 2009. Psychosocial distress and somatic symptoms in community subjects with irritable bowel syndrome: A psychological component is the rule. Am J Gastroenterol. 104:1772–1779.
- Coutinho SV, Plotsky PM, Sablad M, Miller JC, Zhou H, Bayati AI, McRoberts JA, Mayer EA. 2002. Neonatal maternal separation alters stress-induced responses to viscerosomatic nociceptive stimuli in rat. Am J Physiol Gastrointest Liver Physiol. 282:G307–G316.
- Cryan JF, Holmes A. 2005. The ascent of mouse: Advances in modelling human depression and anxiety. Nat Rev Drug Discov. 4:775–790.
- Cryan JF, O'Mahony SM. 2011. The microbiome-gut-brain axis: From bowel to behavior. Neurogastroenterol Motil. 23:187–192.
- Dinan TG, Cryan J, Shanahan F, Keeling PW, Quigley EM. 2010. IBS: An epigenetic perspective. Nat Rev Gastroenterol Hepatol. 7:465–471.
- Drossman DA, Camilleri M, Mayer EA, Whitehead WE. 2002. AGA technical review on irritable bowel syndrome. Gastroenterology. 123:2108–2131.
- Elsenbruch S, Rosenberger C, Enck P, Forsting M, Schedlowski M, Gizewski ER. 2010. Affective disturbances modulate the neural processing of visceral pain stimuli in irritable bowel syndrome: An fMRI study. Gut. 59:489–495.
- File SE, Seth P. 2003. A review of 25 years of the social interaction test. Eur J Pharmacol. 463:35–53.
- Finger BC, Dinan TG, Cryan JF. High-fat diet selectively protects against the effects of chronic social stress in the mouse. Neuroscience. 2011a; 192:351–360.
- Finger BC, Dinan TG, Cryan JF. The temporal impact of chronic intermittent psychosocial stress on high-fat diet-induced alterations in body weight. Psychoneuroendocrinology. 2011b [Epub ahead of print].
- Gosselin RD, O'Connor RM, Tramullas M, Julio-Pieper M, Dinan TG, Cryan JF. 2010. Riluzole normalizes early-life stress-induced visceral hypersensitivity in rats: Role of spinal glutamate reuptake mechanisms. Gastroenterology. 138:2418–2425.
- Gros DF, Antony MM, McCabe RE, Swinson RP. 2009. Frequency and severity of the symptoms of irritable bowel syndrome across the anxiety disorders and depression. J Anxiety Disord. 23:290–296.
- Holschneider DP, Bradesi S, Mayer EA. 2011. The role of experimental models in developing new treatments for irritable bowel syndrome. Expert Rev Gastroenterol Hepatol. 5:43–57.
- Huhman KL. 2006. Social conflict models: Can they inform us about human psychopathology?. Horm Behav. 50:640–646.
- Imbe H, Iwai-Liao Y, Senba E. 2006. Stress-induced hyperalgesia: Animal models and putative mechanisms. Front Biosci. 11:2179–2192.
- Jones MP, Dilley JB, Drossman D, Crowell MD. 2006. Brain-gut connections in functional GI disorders: Anatomic and physiologic relationships. Neurogastroenterol Motil. 18:91–103.
- Koolhaas JM, De Boer SF, De Rutter AJ, Meerlo P, Sgoifo A. 1997. Social stress in rats and mice. Acta Physiol Scand Suppl. 640:69–72.
- Lackner JM, Brasel AM, Quigley BM, Keefer L, Krasner SS, Powell C, Katz LA, Sitrin MD. 2010. The ties that bind: perceived social support, stress, and IBS in severely affected patients. Neurogastroenterol Motil. 22:893–900.
- Larauche M, Gourcerol G, Million M, Adelson DW, Tache Y. 2010. Repeated psychological stress-induced alterations of visceral sensitivity and colonic motor functions in mice: Influence of surgery and postoperative single housing on visceromotor responses. Stress. 13:343–354.
- Larauche M, Mulak A, Tache Y. Stress and visceral pain: From animal models to clinical therapies. Exp Neurol. 2011 [Epub ahead of print].
- Larsson M, Arvidsson S, Ekman C, Bayati A. 2003. A model for chronic quantitative studies of colorectal sensitivity using balloon distension in conscious mice – effects of opioid receptor agonists. Neurogastroenterol Motil. 15:371–381.
- Larsson MH, Rapp L, Lindstrom E. 2006. Effect of DSS-induced colitis on visceral sensitivity to colorectal distension in mice. Neurogastroenterol Motil. 18:144–152.
- Larsson MH, Miketa A, Martinez V. 2009. Lack of interaction between psychological stress and DSS-induced colitis affecting colonic sensitivity during colorectal distension in mice. Stress. 12:434–444.
- Martinez V, Melgar S. 2008. Lack of colonic-inflammation-induced acute visceral hypersensitivity to colorectal distension in Na(v)1.9 knockout mice. Eur J Pain. 12:934–944.
- Martinez M, Calvo-Torrent A, Pico-Alfonso MA. 1998. Social defeat and subordination as models of social stress in laboratory rodents: A review. Aggressive Behav. 24:241–256.
- Mayer EA. 2000. The neurobiology of stress and gastrointestinal disease. Gut. 47:861–869.
- Mayer EA, Tillisch K. 2011. The brain–gut axis in abdominal pain syndromes. Annu Rev Med. 62:381–396.
- Mayer EA, Bradesi S, Chang L, Spiegel BM, Bueller JA, Naliboff BD. 2008. Functional GI disorders: From animal models to drug development. Gut. 57:384–404.
- McEwen BS. 2004. Protection and damage from acute and chronic stress: Allostasis and allostatic overload and relevance to the pathophysiology of psychiatric disorders. Ann NY Acad Sci. 1032:1–7.
- Melgar S, Engstrom K, Jagervall A, Martinez V. 2008. Psychological stress reactivates dextran sulfate sodium-induced chronic colitis in mice. Stress. 11:348–362.
- Miczek KA, Thompson ML, Shuster L. 1982. Opioid-like analgesia in defeated mice. Science. 215:1520–1522.
- Moles A, Bartolomucci A, Garbugino L, Conti R, Caprioli A, Coccurello R, Rizzi R, Ciani B, D'Amato FR. 2006. Psychosocial stress affects energy balance in mice: Modulation by social status. Psychoneuroendocrinology. 31:623–633.
- O'Brien MD, Camilleri M, Thomforde GM, Wiste JA, Hanson RB, Zinsmeister AR. 1997. Effect of cholecystokinin octapeptide and atropine on human colonic motility, tone, and transit. Dig Dis Sci. 42:26–33.
- O'Mahony SM, Hyland NP, Dinan TG, Cryan JF. 2011. Maternal separation as a model of brain–gut axis dysfunction. Psychopharmacology. 214:71–88.
- O'Malley D, Quigley EM, Dinan TG, Cryan JF. 2011. Do interactions between stress and immune responses lead to symptom exacerbations in irritable bowel syndrome?. Brain Behav Immun. 25:1333–1341.
- Ossipov MH, Dussor GO, Porreca F. 2010. Central modulation of pain. J Clin Invest. 120:3779–3787.
- Ouyang A, Wrzos HF. 2006. Contribution of gender to pathophysiology and clinical presentation of IBS: Should management be different in women?. Am J Gastroenterol. 101:S602–S609.
- Reber SO, Obermeier F, Straub RH, Falk W, Neumann ID. 2006. Chronic intermittent psychosocial stress (social defeat/overcrowding) in mice increases the severity of an acute DSS-induced colitis and impairs regeneration. Endocrinology. 147:4968–4976.
- Reber SO, Birkeneder L, Veenema AH, Obermeier F, Falk W, Straub RH, Neumann ID. 2007. Adrenal insufficiency and colonic inflammation after a novel chronic psycho-social stress paradigm in mice: Implications and mechanisms. Endocrinology. 148:670–682.
- Savignac HM, Dinan TG, Cryan JF. Resistance to early-life stress in mice: Effects of genetic background and stress duration. Front Behav Neurosci. 2011a; 4:5–13.
- Savignac HM, Finger BC, Pizzo RC, O'Leary OF, Dinan TG, Cryan JF. Increased sensitivity to the effects of chronic social defeat stress in an innately anxious mouse strain. Neuroscience. 2011b; 192:524–536.
- Savignac HM, Hyland NP, Dinan TG, Cryan JF. The effects of repeated social interaction stress on behavioural and physiological parameters in a stress-sensitive mouse strain. Behav Brain Res. 2011c; 216:576–584.
- Schwetz I, Bradesi S, McRoberts JA, Sablad M, Miller JC, Zhou H, Ohning G, Mayer EA. 2004. Delayed stress-induced colonic hypersensitivity in male Wistar rats: Role of neurokinin-1 and corticotropin-releasing factor-1 receptors. Am J Physiol Gastrointest Liver Physiol. 286:G683–G691.
- Spinelli A. 2007. Irritable bowel syndrome. Clin Drug Invest. 27:15–33.
- Tache Y, Bonaz B. 2007. Corticotropin-releasing factor receptors and stress-related alterations of gut motor function. J Clin Invest. 117:33–40.
- Tamashiro KL, Nguyen MM, Sakai RR. 2005. Social stress: From rodents to primates. Front Neuroendocrinol. 26:27–40.
- Tammpere A, Brusberg M, Axenborg J, Hirsch I, Larsson H, Lindstrom E. 2005. Evaluation of pseudo-affective responses to noxious colorectal distension in rats by manometric recordings. Pain. 116:220–226.
- Terman GW, Shavit Y, Lewis JW, Cannon JT, Liebeskind JC. 1984. Intrinsic mechanisms of pain inhibition: Activation by stress. Science. 226:1270–1277.
- Tramullas M, Lantero A, Diaz A, Morchon N, Merino D, Villar A, Buscher D, Merino R, Hurle JM, Izpisua-Belmonte JC, Hurle MA. 2010. BAMBI (bone morphogenetic protein and activin membrane-bound inhibitor) reveals the involvement of the transforming growth factor-beta family in pain modulation. J Neurosci. 30:1502–1511.
- Vicario M, Alonso C, Guilarte M, Serra J, Martinez C, Gonzalez-Castro AM, Lobo B, Antolin M, Andreu AL, Garcia-Arumi E, Casellas M, Saperas E, Malagelada JR, Azpiroz F, Santos J. Chronic psychosocial stress induces reversible mitochondrial damage and corticotropin-releasing factor receptor type-1 upregulation in the rat intestine and IBS-like gut dysfunction. Psychoneuroendocrinology. 2011 [Epub ahead of print].
- Vidal C, Jacob J. 1982. Hyperalgesia induced by non-noxious stress in the rat. Neurosci Lett. 32:75–80.
- Yu YB, Yang J, Zuo XL, Gao LJ, Wang P, Li YQ. 2010. Transient receptor potential vanilloid-1 (TRPV1) and ankyrin-1 (TRPA1) participate in visceral hyperalgesia in chronic water avoidance stress rat model. Neurochem Res. 35:797–803.