Abstract
The extent to which the hypothalamic–pituitary–adrenal axis is activated by short-term and long-term consequences of stress is still open to investigation. This study aimed to determine (i) the correlation between plasma corticosterone and exploratory behavior exhibited by rats subjected to the elevated plus maze (EPM) following different periods of social isolation, (ii) the effects of the corticosterone synthesis blocker, metyrapone, on the behavioral consequences of isolation, and (iii) whether corticosterone produces its effects through an action on the anterior cingulate cortex, area 1 (Cg1). Rats were subjected to 30-min, 2-h, 24-h, or 7-day isolation periods before EPM exposure and plasma corticosterone assessments. Isolation for longer periods of time produced greater anxiogenic-like effects on the EPM. However, stretched attend posture (SAP) and plasma corticosterone concentrations were increased significantly after 30 min of isolation. Among all of the behavioral categories measured in the EPM, only SAP positively correlated with plasma corticosterone. Metyrapone injected prior to the 24 h isolation period reversed the anxiogenic effects of isolation. Moreover, corticosterone injected into the Cg1 produced a selective increase in SAP. These findings indicate that risk assessment behavior induced by the action of corticosterone on Cg1 neurons initiates a cascade of defensive responses during exposure to stressors.
Introduction
The stress reaction can be activated by emergency or threatening situations and is usually accompanied by fear and anxiety. Together with behavioral and autonomic changes, acute stress also elicits activation of the hypothalamic–pituitary–adrenal (HPA) axis, resulting in the release of adrenocorticotropic hormone (ACTH), which leads to the secretion of glucocorticoids (cortisol in humans and corticosterone in rodents) by the adrenal glands (Andersen et al. Citation2004). Once in the circulation the corticosterone modulates emotional behavior by acting on two types of receptors, mineralocorticoid (MRs) and glucocorticoid (GRs), which are ligand-driven transcription factors that function in genomic control (De Kloet et al. Citation2005). Corticosteroid actions can also be mediated through rapid, non-genomic effects on neuronal membranes, promoting vigilance, alertness, and risk appraisal (Mikics et al. Citation2005; Joëls and Baram Citation2009; Lightman and Conway-Campbell Citation2010). Despite the unquestionable involvement of glucocorticoids in the modulation of emotional behavior, the specific roles of corticosterone in the control of each behavioral component of the defense reaction are still open to investigation (Mikics et al. Citation2005; Hlavacova and Jezova Citation2008).
The elevated plus maze (EPM), validated by Pellow et al. (Citation1985), is one of the most widely used animal tests for the study of anxiety. It is based on the measure of behavioral categories that reflect the conflict resulting from the natural tendency of rodents to explore unknown environments and avoiding potentially dangerous situations (Albrechet-Souza and Brandão Citation2010). Its use involves aversive, innate, proximal and distal mechanisms so the aversive environment represented by the open arms of the maze can act as a stimulus that activates the system of fear and keeps the organism away from the danger (Deakin and Graeff Citation1991). Some studies have suggested a relationship between plasma corticosterone concentrations and exploratory behaviors in the EPM (Rodgers et al. Citation1999; Mikics et al. Citation2005; Albrechet-Souza et al. Citation2007). Indeed, this hormonal response appears to be related to danger detection and, accordingly, it has been associated with behaviors classified as risk assessment, which include stretched attend and approach postures (Rodgers et al. Citation1999; Albrechet-Souza et al. Citation2007; Amaral et al. Citation2010). Factor analyses have shown that these behaviors loaded in a specific factor apart from the traditional categories measured in the EPM, indicating a distinct ethological meaning (Anseloni and Brandão Citation1997; Albrechet-Souza et al. Citation2008). Indeed, risk assessment behaviors are supposed to be involved in the acquisition of environmental information in potentially dangerous situations that, ultimately, optimize the most adaptive behavioral strategies (Blanchard et al. Citation1993; Anseloni and Brandão Citation1997; Albrechet-Souza et al. Citation2007).
Evidence for a time-dependent relationship between HPA axis activation and fear/anxiety states has been repeatedly reported in the literature (Calvo et al. Citation1998; Mikics et al. Citation2005; Joëls and Baram Citation2009). In this sense, different periods of isolation can distinctly affect endocrine and autonomic responses in rodents and their behavioral activity (Kim and Kirkpatrick Citation1996). Social isolation may thus be considered a relevant source of stress and can be used as a non-pharmacological method of inducing relevant changes in behavioral and physiological profiles in rodents (Wright et al. Citation1991; Motta et al. Citation1992; Maisonnette et al. Citation1993; Hall et al. Citation1998; Willner and Mitchell Citation2002; Tomazini et al. Citation2006). Accordingly, isolated rats exhibit increased defensive responses when faced with new situations that normally induce minimal emotional alterations (Hatch et al. Citation1965; Maisonnette et al. Citation1993; Martijena et al. Citation1997; Wiedenmayer Citation2004; Adamec et al. Citation2005; Stam Citation2007).
Recent research has emphasized the role of the anterior cingulate cortex in emotion and cognition, especially in the processing of negative emotional stimuli (Figueiredo et al. Citation2003; Mobbs et al. Citation2007; Etkin et al. Citation2010). Both MRs and GRs have been identified in this brain area (Meaney et al. Citation1985; Diorio et al. Citation1993; Cullinan et al. Citation1995; Herman et al. Citation2005; Cerqueira et al. Citation2007). Moreover, a recent immunohistochemical study showed that the anterior cingulate cortex, area 1 (Cg1), is recruited in aversive situations and plays a key role in exploratory behavior in rats subjected to the EPM (Albrechet-Souza et al. Citation2009). In this study, intra-Cg1 injections of benzodiazepine midazolam produced behavioral effects that were similar to the effects of this compound injected systemically.
This study sought to determine the extent to which the HPA axis is activated by different periods of social isolation and whether plasma corticosterone concentrations correlate with exploratory categories exhibited by rats subjected to the EPM. To further assess the relationship between HPA activation and specific aspects of the defensive reaction, the effects of the corticosterone synthesis inhibitor metyrapone administered prior to social isolation were also evaluated. Finally, to test the hypothesis that corticosterone evokes risk assessment behavior by actions in the Cg1, corticosterone was locally administered into the Cg1 in rats subjected to the EPM to examine the role of this medial prefrontal cortex (mPFC) area in the actions of corticosterone during stressful conditions.
Materials and methods
Subjects
One hundred and fifty-nine male Wistar rats, weighing 280–300 g, from the animal house of the University of São Paulo, campus Ribeirão Preto, were housed in groups of four per cage (40 × 33 × 17 cm) for at least 72 h under a 12/12 h light/dark cycle (lights on at 07:00 h) at 23 ± 1°C and given free access to food and water. Care was taken to minimize the effects of handling stress such as keeping the rats for at least three days in the sector animal house before the experiments began, and the same experimenter (F. M. C. V. Reis) was the only person responsible for cleaning the cages, weighing and transporting the rats to the experimental room. All experiments were conducted in the morning between 09:00 and 11:00 h. The experiments reported in this article were performed in accordance with the recommendations of the Brazilian Society of Neuroscience and Behavior and complied with the United States National Institutes of Health Guide for Care and Use of Laboratory Animals. The procedures were approved by the Committee for Animal Care and Use, University of São Paulo (no. 06.1.123.8.53.9).
Apparatus
The EPM consisted of two open arms (50 × 10 cm) and two closed arms (50 × 10 × 50 cm) that extended from a common central platform (10 × 10 cm). The apparatus, constructed of wood, was configured such that the arms of the same type were opposite to each other and the entire apparatus was elevated to 50 cm from the floor (Pellow et al. Citation1985). A raised edge, made of transparent Plexiglas (1 cm) on the open arms, provided additional grip for the rats. The apparatus was located inside a room with ambient noise (50 dB). Animal behavior was recorded by a video camera (Everfocus, São Paulo, Brazil) positioned above the maze, with the signal relayed to a monitor in another room via a closed circuit television camera. Luminosity at the level of the open arms was 30 lux. The rats were placed individually in the center of the maze facing a closed arm and allowed 5 min of free exploration. Behaviors recorded onto digital video disks were subsequently scored by an experienced observer who was blind to the treatment conditions, using Observer ethological analysis software (Noldus, Amsterdam, The Netherlands). An arm entry or exit was defined as all four paws entering into or out of a section, respectively. This software allowed the measurement of the number of entries into and time spent on each part of the maze, and the frequencies of the following complementary behavioral categories: (1) end-arm exploration (EAE; i.e. number of times the rat reached the end of an open arm), (2) head dipping (i.e. dipping the head below the level of the maze floor), and (3) stretched attend posture (SAP; i.e. when the rat stretches to its full length with the forepaws, keeping its hind paws in the same place, and turns back to the anterior position; Anseloni and Brandão Citation1997; Albrechet-Souza et al. Citation2009).
Drugs
All drugs were freshly prepared on the day of testing. The corticosterone synthesis blocker metyrapone (2-methyl-1, 2-di-3-pyridyl-1-propanone; Sigma-Aldrich, São Paulo, Brazil) was dissolved in 0.9% saline that contained 5% Tween 80. The drug was administered intraperitoneally at a dose of 30 mg/kg in a volume of 1 ml/kg. This dose has been shown to significantly reduce plasma corticosterone concentrations within 20 min without inducing signs of sedation (Mikics et al. Citation2005; Albrechet-Souza et al. Citation2007). Corticosterone (Sigma-Aldrich) was first dissolved in 100% ethanol and subsequently diluted in saline. The final ethanol concentration was 2%. Bilateral infusions of corticosterone (5 ng/0.5 μl per side) or an equivalent volume of vehicle (2% ethanol in saline) was administered locally into the Cg1, 5 min prior to testing (Experiment 2). The dose and time of testing were based on previously published studies (Albrechet-Souza et al. Citation2009; Chauveau et al. Citation2009; Quirarte et al. Citation2009; Roozendaal et al. Citation2009).
Experiment 1: Effects of social isolation on exploratory behavior in rats subjected to the EPM and plasma corticosterone concentrations
This experiment evaluated the effects of the time course of social isolation on exploratory behavior and plasma corticosterone concentrations in rats subjected to the EPM. The analyses of the behavioral and endocrine effects produced by different periods of isolation were performed on 66 rats randomly distributed into five groups: 0 min (control group, n = 14), 30 min (n = 13), 2 h (n = 12), 24 h (n = 13), and 7 days (n = 14). Rats subjected to social isolation were singly housed in Plexiglas cages (30.5 × 19 × 13.5 cm). During the isolation period, rats had free access to food and water and had auditory and olfactory contact with other rats. At the end of the isolation period, rats were subjected to the EPM for 5 min. Immediately after the EPM exposure, they were euthanized by decapitation without anesthesia and trunk blood was collected in heparinized tubes. Thirty-six rats were subjected to the same periods of isolation (n = 7–8 per group) but not exposed to the EPM. Blood samples were immediately collected after the isolation period.
Based on our experimental findings and previous data that showed a relationship between SAP and plasma corticosterone concentrations (Rodgers et al. Citation1999; Mikics et al. Citation2005; Albrechet-Souza et al. Citation2007), an additional experiment was conducted to evaluate whether corticosterone blockade and, consequently, metyrapone-induced SAP inhibition during the earlier phases of isolation affect the later phases of stress response. The 24 h period of isolation was chosen because it has been associated with prominent anxiogenic-like effects (Maisonnette et al. Citation1993; Calvo et al. Citation1998). Thirty-four rats were randomly divided into four groups: grouped rats that received either vehicle (n = 8) or metyrapone (30 mg/kg, intraperitoneally, n = 9), and isolated rats that also received vehicle (n = 8) or metyrapone (n = 9). After 20 min, they were subjected to a 24 h isolation period, immediately followed by 5 min EPM exposure.
Corticosterone assay
Blood samples were collected in the morning between 09:00 and 11:00 h in heparinized plastic tubes and centrifuged at 1200g at 4°C for 15 min. Plasma was separated and frozen at − 20°C until the assay. All samples were measured in the same assay to avoid interassay variation. Radioimmunoassay for corticosterone required plasma extraction using ethanol. The antibody and standard were provided by Sigma (St. Louis, MO, USA), and the 3H-labeled hormone was obtained from Amersham (Pittsburgh, PA, USA). The lower limit for detection was 2 ng/ml, and the intra-assay coefficient of variation was 5%.
Experiment 2: Behavioral effects of corticosterone locally injected into the Cg1
This experiment examined whether Cg1 is a target area for the rapid effects of corticosterone on exploratory behavior of rats in the EPM. Rats with guide cannulae directed to the Cg1 received an intraperitoneal pretreatment with metyrapone and were allocated to two different groups: rats that received bilateral intra-Cg1 injections of 0.5 μl of vehicle (2% ethanol in saline, n = 8) or corticosterone (5 ng/0.5 μl, n = 11). Five minutes later, they were subjected to the EPM for 5 min. Metyrapone was administered to prevent an eventual synthesis and release of endogenous corticosterone during the EPM test that could confound effects produced by intra-Cg1 injections of corticosterone. Behavioral testing was started 20 min after metyrapone injections.
Surgery
Six days before the experiment, rats were anesthetized with intraperitoneal ketamine/xylazine at a dose of 100/7.5 mg/kg, in a volume of 1/0.3 ml/kg, respectively, and fixed in a stereotaxic apparatus (David Kopf Instruments, Tujunga, CA, USA). The upper incisor bar was set 3.3 mm below the interaural line so that the skull was horizontal between bregma and lambda. After scalp anesthesia with 2% lidocaine, the skull was surgically exposed and stainless steel guide cannulae (length, 10 mm; outer diameter, 0.6 mm; inner diameter, 0.4 mm) were bilaterally implanted to target the Cg1. The coordinates for cannula implantation were the following: anterior/posterior, +2.2 mm; lateral, 0.9 mm from the median suture; ventral, − 2.0 mm from the skull surface (Paxinos and Watson Citation2007). Cannulae were fixed to the skull by means of acrylic resin and two stainless steel screws. At the end of the surgery, each guide cannula was sealed with a stainless steel wire to prevent obstruction and rats received an intramuscular injection of penicillin-G benzathine (Pentabiotic, 600,000 IU in a 0.2 ml volume; Fort Dodge, Campinas, SP, Brazil) and a subcutaneous injection of the anti-inflammatory analgesic Banamine (2.5 mg/kg flunixin meglumine in a volume of 0.2 ml). After surgery, rats were placed again in their home cages in groups of four.
Infusion procedure
Intracerebral infusions were delivered via an infusion pump (Harvard Apparatus, Holliston, MA, USA) at a rate of 0.5 μl/min. The volume of injection was chosen based on a previous study (Albrechet-Souza et al. Citation2009) and literature data have shown that the diffusion extent of this volume is on average 1 mm (Myers Citation1966). A fine dental needle (outer diameter, 0.3 mm) was introduced through each guide cannula attached by polyethylene tubing to a 5 μl Hamilton syringe (Reno, NV, USA). The injection needle extended 0.5 mm below the ventral tip of the implanted guide cannula, and bilateral infusions were conducted simultaneously. The displacement of an air bubble inside the polyethylene tubing (PE-10; Becton-Dickinson, Franklin Lakes, NJ, USA) that connected the syringe to the injection needle was used to monitor the microinjections. The injection needles were left in place for 1 min after the end of the infusion period to allow for diffusion.
Histology
Upon conclusion of the experiments, rats were deeply anesthetized with intraperitoneal ketamine/xylazine at a dose of 300/30 mg/kg in a volume of 3/1.5 ml/kg, respectively, and perfused transcardially with 0.9% saline followed by buffered 10% formalin. Brains were removed from the skulls, maintained in formalin solution for 2 h, and cryoprotected in 30% sucrose for 72 h. Serial 60 μm coronal brain sections were cut using a cryostat ( − 19°C), mounted on gelatin-coated slides, and stained with Cresyl Violet (5%; Sigma-Aldrich) to localize the positions of the microinjection sites according to the atlas of Paxinos and Watson (Citation2007). Microinjection sites were evaluated by microscopic examination. Four rats were deemed to have inappropriate cannula placements.
Statistical analysis
The software used for all statistical analyses was STATISTICA version 6.0. Data are expressed as mean ± SEM. In Experiment 1, behavioral data were analyzed by one-way analysis of variance (ANOVA), and hormonal data were analyzed by two-way ANOVA, with isolation period (0 min, 30 min, 2 h, 24 h, and 7 days) and EPM exposure (exposed and non-exposed) as the factors. Relationships between plasma corticosterone concentrations and behavioral categories were examined by Spearman's rank-order correlation. Behavioral data obtained with metyrapone administered prior to the 24-h isolation period were analyzed by two-way ANOVA, with treatment (vehicle and metyrapone) and housing condition (grouped and isolated) as the factors. Newman–Keuls post-hoc comparisons were performed if significant overall F-values were obtained in the ANOVAs. In Experiment 2, data were analyzed by Student's t-test. In all experiments, values of p < 0.05 were considered statistically significant.
Results
Experiment 1: Effects of social isolation on exploratory behavior in rats subjected to the EPM and plasma corticosterone concentrations
The effects of different periods of social isolation on exploratory behavior in rats subjected to the EPM are illustrated in . One-way ANOVA revealed a significant effect on the number of open arm entries and percentage of time spent on these arms (F4, 61 = 4.90 and 8.47, respectively, p < 0.001 in both cases). No significant effects were detected on the number of closed arm entries (F4, 61 = 0.98, p>0.05). The post-hoc analysis revealed that rats subjected to 24 h and 7 days of isolation showed a reduction in the number of open arm entries compared with the control group (0 min). Moreover, rats subjected to 2 h, 24 h and 7 days of social isolation exhibited a decrease in the percentage of time spent on the open arms of the maze. With regard to the effects of different periods of isolation on complementary behavioral parameters, the one-way ANOVA revealed a significant effect on the frequency of EAE, head dipping and SAP (F4, 61 = 4.58, 9.15, and 3.03, respectively; p < 0.05 in all cases). The post-hoc analysis indicated that rats subjected to 30 min of isolation exhibited an increased frequency of SAP, whereas rats subjected to 2 h, 24 h, and 7 days of isolation exhibited a reduction in the frequency of head dipping. Rats isolated for 24 h and 7 days also showed a reduction in the exploration of the open ends of the EPM.
Figure 1. Effects of different periods of social isolation (0 min, 30 min, 2 h, 24 h and 7 days) on exploratory behavior of rats subjected to the EPM. Control rats (0 min) were kept in groups of four before being subjected to the test. Data are expressed as mean ± SEM. (A) Number of entries into the open arms of the maze; (B) number of entries into the closed arms of the maze; (C) percentage of time spent on the open arms in relation to total; (D) number of EAE; (E) number of head dips; (F) number of SAP. *p < 0.05, different from control group (0 min; one-way ANOVA followed by Newman-Keuls post-hoc test; n = 12–14 per group).
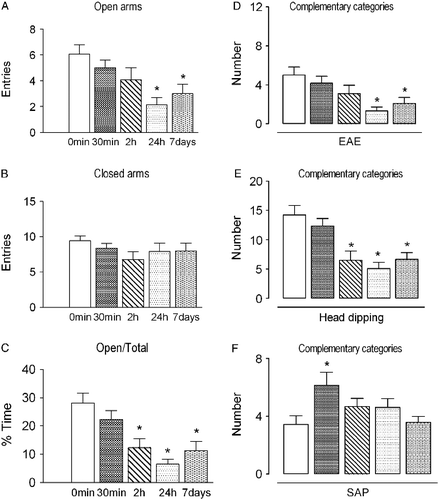
The effects of different periods of isolation in rats subjected or not to the EPM on corticosterone secretion are shown in . Two-way ANOVA revealed significant effects of isolation period (F4, 92 = 10.45, p < 0.001) and EPM exposure (F1, 92 = 14.35, p < 0.001) on plasma corticosterone concentrations. No significant isolation period × EPM exposure interaction was found (F4, 92 = 0.27, p>0.05). The post-hoc analysis showed a significant increase in plasma corticosterone concentrations in all groups subjected to the EPM compared with the respective non-exposed group. Moreover, a significant increase in plasma corticosterone was observed in both groups, in rats subjected to isolation for 30 min compared with the corresponding control group (0 min).
Figure 2. Plasma corticosterone concentrations (expressed as ng/ml) measured in rats subjected to different periods of social isolation (0 min, 30 min, 2 h, 24 h and 7 days) and exposed to or not to the EPM. Control rats (0 min) were kept in groups of four rats. Data are expressed as mean ± SEM. *p < 0.05, different from the respective control group (0 min); #p < 0.05, different from the group isolated for the same period and not exposed to the EPM (two-way ANOVA followed by Newman–Keuls post-hoc test (EPM exposed, n = 12–14 per group; EPM non-exposed, n = 7–8 per group).
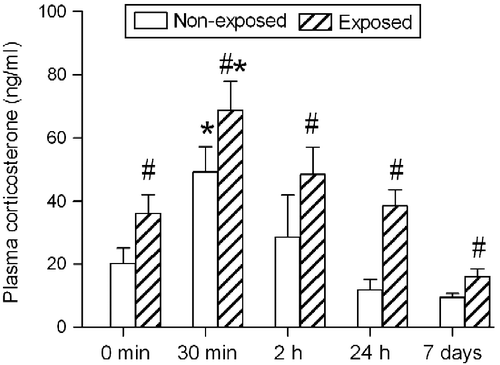
Spearman's test showed a significant positive correlation between plasma corticosterone concentrations and frequencies of SAP including all time points of isolation (r = 0.4, p < 0.01; ). Other behavioral categories did not present significant correlations with corticosterone (p>0.05 in all cases).
Figure 3. Spearman's correlation coefficient between plasma corticosterone concentration and frequency of SAP in rats subjected to different periods of social isolation (0 min, 30 min, 2 h, 24 h and 7 days) and exposed to the EPM. The correlation analysis included all time points of isolation, n = 66. Correlation is significant, p < 0.01.
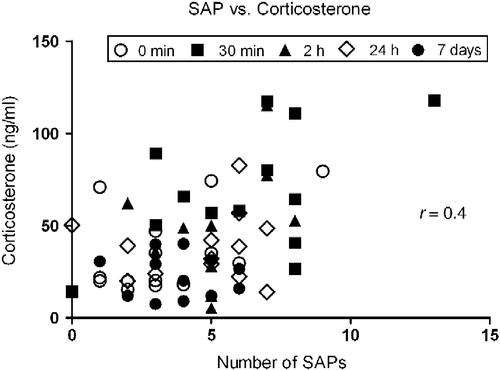
The effects of metyrapone administered intraperitoneally prior to 24-h social isolation on exploratory behavior in rats subjected to the EPM are shown in . Two-way ANOVA revealed significant effects of housing condition (grouped vs. isolated) on the number of entries into the open arms and percentage of time spent on these arms (F1, 30 = 8.70 and 3.93, respectively; p < 0.05 in both cases). No significant effect of treatment was found on the number of open arm entries or percentage of time spent on these arms (F1, 30 = 2.56 and 1.48, respectively; p>0.05 in both cases). A significant interaction was found between housing condition × treatment in the number of open arm entries and percentage of time spent on these arms (F1, 30 = 4.09 and 7.41, respectively; p < 0.05 in both cases). Regarding the number of closed arm entries, no significant effects of housing condition or treatment were found, with no housing condition × treatment interaction (F1, 30 = 0.47, 0.04 and 0.09, respectively; p>0.05 in all cases). The post-hoc analysis showed that the group injected with vehicle and isolated for 24 h exhibited a decrease in activity on the open arms of the maze compared with other groups.
Table I. Effects of metyrapone treatment before 24 h social isolation on exploratory behavior of rats subjected to the EPM.
Two-way ANOVA revealed significant effects of housing condition and an interaction between housing condition × treatment on the frequency of head dipping (F1, 30 = 11.29 and 11.68, respectively; p < 0.01 in both cases) but no effect of treatment (F1, 30 = 1.75, p>0.05). The post-hoc analysis showed a significant decrease in the frequency of head dipping in rats isolated for 24 h and treated with vehicle compared with other groups. With regard to the frequency of EAE, two-way ANOVA revealed no significant effects of housing condition or treatment and no interaction between these factors (F1, 30 = 1.46, 3.16, and 1.58, respectively; p>0.05 in all cases). However, Student's t-test applied to vehicle/grouped vs. vehicle/isolated groups, revealed a significant difference in this category (t = 2.56, p < 0.03). With respect to the frequency of SAP, no significant effects of housing condition or treatment were found, with no significant interaction between these factors (F1, 30 = 2.24, 2.73, and 0.41, respectively; p>0.05 in all cases).
Experiment 2: Behavioral effects of corticosterone locally injected into the Cg1
A diagrammatic representation of the vehicle and corticosterone injection sites and a photomicrograph that shows a representative bilateral injection site in the Cg1 are shown in . The effects of intra-Cg1 vehicle or corticosterone administration on exploratory behavior of rats subjected to the EPM are illustrated in . Student's t-test revealed no significant effects on the frequency of open arm entries, percentage of time that rats spent on the open arms, or number of closed arm entries (t = 1.25, 0.90, and 0.70, respectively, p>0.05 in all cases). With respect to the effects on the complementary behavioral categories, Student's t-test revealed that rats treated with intra-Cg1 corticosterone exhibited a significant increase in SAP frequency (t = 2.85, p < 0.01) but no significant effects on EAE and head dipping (t = 1.44 and 1.75, respectively, p>0.05 in both cases).
Figure 4. Photomicrograph of a coronal rat brain section showing representative sites and location of bilateral injection into the Cg1, based on the rat brain atlas of Paxinos and Watson (Citation2007). The number of points in the figure is less than the total number of rats used in the study because of overlapping injection sites. The positions of the representations relative to bregma are in millimeters. Scale bar = 1 mm. cc, corpus callosum.
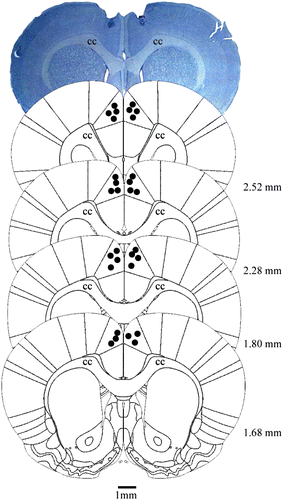
Figure 5. Effects of intra-Cg1 injection of vehicle or corticosterone (5 ng/0.5 μl) on exploratory behavior of rats subjected to the EPM. Data are expressed as mean ± SEM. (A) Number of entries into the open arms of the maze; (B) number of entries into the closed arms of the maze; (C) percentage of time spent on the open arms in relation to total; (D) number of EAE; (E) number of head dips; (F) number of SAP. *p < 0.05, different from the vehicle group (Student's t-test; n = 8–11 per group).
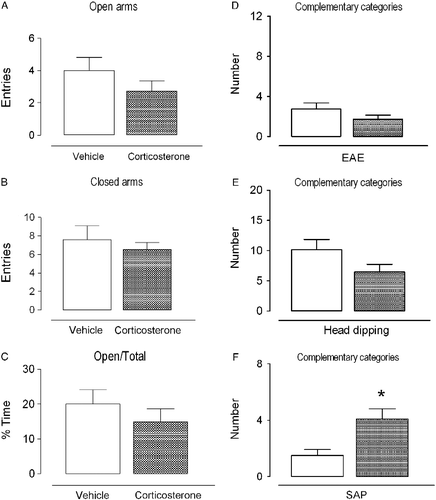
Discussion
In this study, behavioral data from Experiment 1 replicated previous results. Rats subjected to social isolation displayed an increased aversion to the open arms of the EPM as a function of the isolation period, indicating that this procedure induces rapid activation of the neural substrates responsible for aversive states (Motta et al. Citation1992; Maisonnette et al. Citation1993). Thus, rats submitted to longer periods of isolation exhibited greater preference for the closed arms and, consequently, less activity was exhibited in the open areas of the maze. As expected, this decrease in open arm activity was accompanied by reduced exploration of the extremities and frequency of head dipping, a behavioral profile indicative of anxiety-like states (Anseloni and Brandão Citation1997; Rodgers et al. Citation1999; Albrechet-Souza et al. Citation2007).
Hormonal data also support previous studies demonstrating that plasma corticosterone reaches its peak approximately 20 min after the beginning of the aversive stimulus (Hennessy and Levine Citation1979; File et al. Citation1994; Genaro et al. Citation2004; Albrechet-Souza et al. Citation2007). In this study, the highest plasma corticosterone concentrations were found after 30 min of social isolation. Interestingly, even a short period of 5 min exposure to the EPM was able to produce an increase in plasma corticosterone compared with rats not subjected to the test, corroborating previous studies showing that EPM exposure is able to activate the HPA axis (File et al. Citation1994; Calvo et al. Citation1998; Rodgers et al. Citation1999; Mikics et al. Citation2005; Albrechet-Souza et al. Citation2007). The fact that rats were killed just 5 min after the start of EPM exposure obviously prevented further higher increases in plasma corticosterone concentrations. Moreover, as attested by the lack of interaction between periods of isolation and EPM exposure, social isolation, even for longer periods, did not induce a further sensitization of corticosterone secretion in rats subsequently submitted to the EPM.
Besides, 30-min isolation induced a specific increase in the frequency of SAP. This behavioral category has been defined as risk assessment behavior and appears to have a different meaning from the traditional categories measured in the EPM, which may be related to the acquisition of environmental information in potentially dangerous situations (Blanchard and Blanchard Citation1989; Blanchard et al. Citation1993; Cole and Rodgers Citation1993; Anseloni and Brandão Citation1997; Albrechet-Souza et al. Citation2007). Moreover, an increase in SAP occurred concomitantly with increased plasma corticosterone concentrations. Corroborating these results, a previous study has shown that the frequency of SAP is sensitive to treatment with midazolam and metyrapone, compounds that decrease plasma corticosterone concentrations by distinct means (Albrechet-Souza et al. Citation2007). These findings are consistent with the hypothesis that plasma corticosterone concentrations are positively correlated with risk assessment behaviors (Rodgers et al. Citation1999; Mikics et al. Citation2005; CitationAmaral et al. 2010; Lightman and Conway-Campbell Citation2010).
The gradual increase in the length of social isolation was accompanied by the development of an anxiety-like profile, with no late changes in HPA axis reactivity. Thus, activation of this system appears to occur immediately after the detection of an aversive situation and not to be involved in the maintenance of the defensive response (Hennessy and Levine Citation1979; Rodgers et al. Citation1999). After the initial period of reaction to the stressor, activation of feedback mechanisms might occur, reducing the production of ACTH by the pituitary and consequently decreasing plasma corticosterone concentrations in blood (Garcia et al. Citation2000; Rodrigues et al. Citation2009).
Rats treated with vehicle and isolated for 24 h exhibited an anxiety-like profile reflected by a decrease in open arm activity and head dipping movements. This behavioral pattern, however, was prevented by pretreatment with the corticosterone synthesis inhibitor metyrapone, indicating the influence of HPA axis mobilization during the earlier phases of the isolation period on the subsequent reactivity to aversive situations. Moreover, metyrapone did not produce behavioral changes in grouped rats, indicating that increases in plasma corticosterone concentrations, during the beginning of the isolation period, interacts with their defensive behavioral profile in response to aversive stimuli presented later. Similar results have been reported with the use of other stressors, supporting the possibility of behavioral changes mediated through GR mechanisms (Calvo et al. Citation1998). Altogether, these results indicate that while increases in risk assessment behaviors soon after stress onset appear to be associated with the rapid membrane effects of corticosterone, its prolonged effects, reflected by an anxiety-like profile, seem to be mediated by the binding of cytoplasmic MRs and GRs (Cullinan et al. Citation1995; Calvo et al. Citation1998; Calvo and Volosin Citation2001; Pryce Citation2008), which are widely distributed in areas related to the modulation and expression of emotional behaviors, such as paraventricular nucleus of the hypothalamus, amygdaloid complex, hippocampus and mPFC (Graeff Citation1981, Citation1994; Gray and McNaughton Citation2000; Pacak and Palkovitz Citation2001; Duvarci and Paré Citation2007; Albrechet-Souza et al. Citation2008, Citation2009). Thus, immediate corticosterone release in response to a stressful situation appears to play a facilitatory role in the occurrence of fear responses to subsequent threatening situations (Martijena et al. Citation1997; Calvo et al. Citation1998; Mitra et al. Citation2009).
In Experiment 2, bilateral infusions of corticosterone into the Cg1 increased SAP frequency without affecting other behavioral categories exhibited by rats subjected to the EPM. Notably, a previous study has shown that the benzodiazepine midazolam injected intraperitoneally decreased Fos activation in the Cg1 of rats subjected to the EPM, and local administration of this compound produced less selective behavioral effects, reducing measures of risk assessment behaviors and open arms avoidance in rats subjected to the EPM (Albrechet-Souza et al. Citation2009). Indeed, Cg1, as part of the mPFC, which also includes the anterior cingulate cortex, area 2, and prelimbic and infralimbic cortices (Singewald Citation2007), has been found to participate in emotional and cognitive modulation related to the processing of negative stimuli (Mobbs et al. Citation2007; Etkin et al. Citation2010; Shackman et al. Citation2011).
The mPFC is considered a strategic center associated with other limbic regions involved in several aspects of the stress response, including learning and memory, decision-making and emotional and hormonal responses (Cerqueira et al. Citation2007; Joëls and Baram Citation2009; Barsegyan et al. Citation2010; Kim et al. Citation2011). Besides expressing both MRs and GRs (Meaney et al. Citation1985; Chao et al. Citation1989; Patel et al. Citation2000; Brown et al. Citation2005), this area has also indirect anatomical connections with the paraventricular nucleus of the hypothalamus via bed nucleus of the stria terminalis, which points to its involvement in the mediation of endocrine responses related to stress (Diorio et al. Citation1993; Sullivan and Gratton Citation2002; Radley et al. Citation2009). Additionally, findings from several electrophysiological and behavioral studies also demonstrated reciprocal connections between the mPFC and basolateral amygdala that may regulate fear and memory (Brinley-Reed et al. Citation1995; McDonald et al. Citation1996; File et al. Citation1998; McDonald Citation1998; Quirk et al. Citation2003; Likhtik et al. Citation2005; Roozendaal et al. Citation2009; Sotres-Bayon and Quirk Citation2010).
Results from Experiment 2 further confirm the rapid, non-genomic, corticosterone effects associated with the onset of the stress response as indicated by Experiment 1. In this respect, the present data point to the Cg1 as an important area for this corticosterone action leading to risk assessment behaviors induced by isolation and other anxiogenic conditions, which may be the starting point for the defense reactions organized at subcortical levels (Rodgers et al. Citation1999; Mikics et al. Citation2005; Joëls et al. Citation2007; Lightman and Conway-Campbell Citation2010; Musazzi et al. Citation2010). Moreover, several studies have suggested that the mechanisms underlying these rapid non-genomic effects may involve glutamatergic neurotransmission (Karst et al. Citation2002; Joëls et al. Citation2007; Krugers et al. Citation2010). This phenomenon has already been observed in the frontal cortex and hippocampal CA1 pyramidal cells in rats (Moghaddam Citation1993; Karst et al. Citation2005; Musazzi et al. Citation2010).
Conclusions
Behavioral defense reactions follow a monotonic function with regard to the period of social isolation. Corticosterone reached the highest plasma concentration 30 min after the beginning of social isolation and then returned to basal concentrations between 2 and 24 h. This hormonal response positively correlated with risk assessment behaviors displayed by rats subjected to the EPM. Interestingly, metyrapone, a corticosterone synthesis blocker, administered before a 24-h isolation period counteracted the anxiogenic-like effects caused by this stressful stimulus, characterized by low exploration of the EPM open arms. Altogether, these results indicate that HPA axis activation that occurs at the beginning of the stressful condition appears to be an initial step in an integrated hormonal-cognitive-behavior response when the organism evaluates the threat associated with the aversive environment, and thereafter triggers the subsequent cascade of defense reactions. Infusions of corticosterone into the Cg1 replicated the initial adaptive physiological–behavioral response to the 30 min isolation period, indicating that this area is critically involved in the cognitive strategies (risk assessment) adopted by rodents to cope with stressful situations. This important association between endocrine and cognitive systems allows information gathering in potentially threatening situations, leading the animal to execute the most appropriate and adaptive behavioral strategies.
Acknowledgements
This work was supported by FAPESP (Proc. No 11/00041-3). F. M. C. V. Reis and L. Albrechet-Souza are recipients of scholarships from CAPES and CNPq, respectively. M. L. Brandão (Proc. No 300074/2009-3) is a research fellow from CNPq.
Declaration of Interest: The authors report no conflicts of interest. The authors alone are responsible for the content and writing of the paper.
References
- Adamec RE, Blundell J, Burton P. 2005. Neural circuit changes mediating lasting brain and behavioral response to predator stress. Neurosci Biobehav Rev. 29:1225–1241.
- Albrechet-Souza L, Brandão ML. 2010. Hormonal and cognitive factors associated with the exploratory behavior of rats submitted to repeated sessions of the elevated plus-maze. Psychol Neurosci. 3:43–52.
- Albrechet-Souza L, Carvalho MC, Franci CR, Brandão ML. 2007. Increases in plasma corticosterone and stretched-attend postures in rats naive and previously exposed to the elevated plus-maze are sensitive to the anxiolytic-like effects of midazolam. Horm Behav. 52:267–273.
- Albrechet-Souza L, Borelli KG, Brandão ML. 2008. Activity of the medial prefrontal cortex and amygdala underlies one-trial tolerance of rats in the elevated plus-maze. J Neurosci Methods. 169:109–118.
- Albrechet-Souza L, Borelli KG, Carvalho MC, Brandão ML. 2009. The anterior cingulate cortex is a target structure for the anxiolytic-like effects of benzodiazepines assessed by repeated exposure to the elevated plus-maze and Fos immunoreactivity. Neuroscience. 164:387–397.
- Amaral VC, Santos Gomes K, Nunes-De-Souza RL. 2010. Increased corticosterone levels in mice subjected to the rat exposure test. Horm Behav. 57:128–133.
- Andersen ML, Bignotto M, Machado RB, Tufik S. 2004. Different stress modalities result in distinct steroid hormone responses by male rats. Braz J Med Biol Res. 37:791–797.
- Anseloni VCZ, Brandão ML. 1997. Ethopharmacological analysis of behaviour of rats using variations of the elevated plus-maze. Behav Pharmacol. 8:533–540.
- Barsegyan A, Mackenzieb STM, Kuroseb BD, McGaughb JL, Roozendaal B. 2010. Glucocorticoids in the prefrontal cortex enhance memory consolidation and impair working memory by a common neural mechanism. Proc Natl Acad Sci USA. 107:16655–16660.
- Blanchard RJ, Blanchard DC. 1989. Antipredator defensive behaviors in a visible burrow system. J Comp Psychol. 103:70–82.
- Blanchard RJ, Yudko EB, Rodgers RJ, Blanchard DC. 1993. Defense system psychopharmacology: An ethological approach to the pharmacology of fear and anxiety. Behav Brain Res. 58:155–165.
- Brinley-Reed M, Mascagni F, McDonald AJ. 1995. Synaptology of prefrontal projections to the basolateral amygdala: An electron microscopic study in the rat. Neurosci Lett. 202:45–48.
- Brown SM, Henning S, Wellma CL. 2005. Mild, short-term stress alters dendritic morphology in rat medial prefrontal cortex. Cereb Cortex. 15:1714–1722.
- Calvo N, Volosin M. 2001. Glucocorticoid and mineralocorticoid receptors are involved in the facilitation of anxiety-like response induced by restraint. Neuroendocrinology. 73:261–271.
- Calvo N, Martijena ID, Molina VA, Volosin M. 1998. Metyrapone pretreatment prevents the behavioral and neurochemical sequelae induced by stress. Brain Res. 800:227–235.
- Cerqueira JJ, Taipa R, Uylings HBM, Almeida FXO, Sousa N. 2007. Specific configuration of dendritic degeneration in pyramidal neurons of the medial prefrontal cortex induced by differing corticosteroid regimens. Cereb Cortex. 17:1998–2006.
- Chao HM, Choo PH, McEwen BS. 1989. Glucocorticoid and mineralocorticoid receptor mRNA expression in rat brain. Neuroendocrinology. 50:365–371.
- Chauveau F, Piérard C, Tronche C, Coutan M, Drouet I, Liscia P, Béracoché D. 2009. The hippocampus and prefrontal cortex are differentially involved in serial memory retrieval in non-stress and stress conditions. Neurobiol Learn Mem. 91:447–455.
- Cole JC, Rodgers RJ. 1993. An ethological analysis of of the effects of chlordiazepoxide and bretazenil (Ro 16-6028) in the murine elevated plus-maze. Behav Pharmacol. 4:573–580.
- Cullinan WE, Herman JP, Battaglia DF, Akil H, Watson SJ. 1995. Pattern and time course of immediate early gene expression in rat brain following acute stress. Neuroscience. 64:477–505.
- Deakin JFW, Graeff FG. 1991. 5-HT and mechanisms of defense. J Psychopharmacol. 5:305–315.
- De Kloet ER, Joëls M, Holsboer F. 2005. Stress and the brain: From adaptation to disease. Nat Rev Neurosci. 6:463–475.
- Diorio D, Viau V, Meaney MJ. 1993. The role of the medial prefrontal cortex (cingulate gyrus) in the regulation of hypothalamic–pituitary–adrenal responses to stress. J Neurosci. 13:3839–3847.
- Duvarci S, Paré D. 2007. Glucocorticoids enhance the excitability of principal basolateral amygdala neurons. J Neurosci. 27:4482–4491.
- Etkin A, Egner T, Kalisch R. 2010. Emotional processing in anterior cingulate and medial prefrontal córtex. Trends Cogn Sci. 15:85–93.
- Figueiredo HF, Bruestle A, Bodie B, Dolgas CM, Herman JP. 2003. The medial prefrontal cortex differentially regulates stress-induced c-fos expression in the forebrain depending on type of stressor. Eur J Neurosci. 18:2357–2364.
- File SE, Zangrossi HJr, Sanders FL, Mabbutt PS. 1994. Raised corticosterone in the rat after exposure to the elevated plus-maze. Psychopharmacology (Berl). 113:543–546.
- File SE, Gonzalez LE, Gallant R. 1998. Role of the basolateral nucleus of the amygdala in the formation of a phobia. Neuropsychopharmacology. 19:397–405.
- Garcia A, Marti O, Valles A, Dal-Zotto S, Armario A. 2000. Recovery of the hypothalamic–pituitary–adrenal response to stress: Effect of stress intensity, stress duration and previous stress exposure. Neuroendocrinology. 72:114–125.
- Genaro G, Schmidek WR, Franci CR. 2004. Social condition affects hormone secretion and exploratory behavior in rats. Braz J Med Biol Res. 37:833–840.
- Graeff FG. 1981. Minor tranquilizers and brain defense systems. Braz J Med Biol Res. 14:239–265.
- Graeff FG. 1994. Neuroanatomy and neurotransmitter regulation of defensive behaviors and related emotions in mammals. Braz J Med Biol Res. 27:811–829.
- Gray JA, McNaughton N. 2000. The neuropsychology of anxiety: An enquiry into the functions of the septo-hippocampal system. Oxford: Oxford University Press.
- Hall FS, Wilkinson LS, Humby T, Inglis W, Kendall DA, Marsden CA, Robins TW. 1998. Isolation rearing in rats: Pre- and postsynaptic changes in striatal dopaminergic systems. Pharmacol Biochem Behav. 59:859–872.
- Hatch AM, Wiberg GS, Zawidzka Z, Cann M, Airth JM, Grice HC. 1965. Isolation syndrome in the rat. Toxicol Appl Pharmacol. 7:737–745.
- Hennessy JW, Levine S. 1979. Stress, arousal and the pituitary–adrenal system: A psychoendocrine hypothesis. In: Sprague JM, Epstein AN. editors. Progress in psychobiology and physiological psychology. New York: Academic Press133–178.
- Herman JP, Ostrander MM, Mueller NK, Figueiredo H. 2005. Limbic system mechanisms of stress regulation: Hypothalamo–pituitary–adrenocortical axis. Prog Neuropsychopharmacol Biol Psychiatry. 29:1201–1213.
- Hlavacova N, Jezova D. 2008. Chronic treatment with the mineralocorticoid hormone aldosterone results in increased anxiety-like behavior. Horm Behav. 54:90–97.
- Joëls M, Baram TZ. 2009. The neuro-symphony of stress. Nat Rev Neurosci. 10:459–466.
- Joëls M, Karst H, De Rijk R, De Kloet ER. 2007. The coming out of the brain mineralocorticoid receptor. Trends Neurosci. 31:1–7.
- Karst H, Nair S, Velzing E, Rumpff-van Essen L, Slagter E, Shinnick-Gallagher P, Joëls M. 2002. Glucocorticoids alter calcium conductances and calcium channel subunit expression in basolateral amygdala neurons. Eur J Neurosci. 16:1083–1089.
- Karst H, Berger S, Turiault M, Tronche F, Schutz G, Joëls M. 2005. Mineralocorticoid receptors are indispensable for non-genomic modulation of hippocampal glutamate transmission by corticosterone. Proc Natl Acad Sci USA. 102:19204–19207.
- Kim JW, Kirkpatrick B. 1996. Social isolation in animal models of relevance to neuropsychiatric disorders. Biol Psychiatry. 40:918–922.
- Kim SS, Wang H, Li XY, Chen T, Mercaldo V, Descalzi G, Wu LJ, Zhuo M. 2011. Neurabin in the anterior cingulate cortex regulates anxiety-like behavior in adult mice. Mol Brain. 4:6.
- Krugers HJ, Hoogenraad CC, Groc L. 2010. Stress hormones and AMPA receptor trafficking in synaptic plasticity and memory. Nat Rev Neurosci. 11:675–681.
- Lightman SL, Conway-Campbell BL. 2010. The crucial role of pulsatile activity of the HPA axis for continuous dynamic equilibration. Nat Rev Neurosci. 11:710–718.
- Likhtik E, Pelletier JG, Paz R, Paré D. 2005. Prefrontal control of the amygdala. J Neurosci. 25:7429–7437.
- Maisonnette S, Morato S, Brandão ML. 1993. Role of resocialization and 5-HT1A receptor activation on the anxiogenic effects induced by isolation in the elevated plus-maze test. Physiol Behav. 54:753–758.
- Martijena ID, Calvo N, Volosin M, Molina VA. 1997. Prior exposure to a brief restraint session facilitates the occurrence of fear in response to a conflict situation: Behavioral and neurochemical correlates. Brain Res. 752:136–142.
- McDonald AJ. 1998. Cortical pathways to the mammalian amygdala. Prog Neurobiol. 55:257–332.
- McDonald AJ, Mascagni F, Guo L. 1996. Projections of the medial and lateral prefrontal cortices to the amygdala: A Phaseolus vulgaris leucoagglutinin study in the rat. Neuroscience. 71:55–75.
- Meaney MJ, Aitken DH, Bodnoff SR, Iny LJ, Tatarewicz JE, Sapolsky RM. 1985. Early postnatal handling alters glucocorticoid receptor concentrations in selected brain regions. Behav Neurosci. 99:765–770.
- Mikics E, Barsy B, Barsvari B, Haller J. 2005. Behavioral specificity of non-genomic glucocorticoid effects in rats: Effects on risk assessment in the elevated plus-maze and the open-field. Horm Behav. 48:152–162.
- Mitra R, Ferguson D, Sapolsky RM. 2009. Mineralocorticoid receptor overexpression in basolateral amygdala reduces corticosterone secretion and anxiety. Biol Psychiatry. 66:686–690.
- Myers RD. 1966. Injection of solutions into cerebral tissue: Relation between volume and diffusion. Physiol Behav. 1:171–174.
- Mobbs D, Petrovic P, Marchant JL, Hassabis D, Weiskopf N, Seymour B, Dolan RJ, Frith CD. 2007. When fear is near: Threat imminence elicits prefrontal-periaqueductal gray shifts in humans. Science. 317:1079–1083.
- Moghaddam B. 1993. Stress preferentially increases extraneuronal levels of excitatory amino acids in the prefrontal cortex: Comparison to hippocampus and basal ganglia. J Neurochem. 60:1650–1657.
- Motta V, Maisonette S, Morato S, Castrechini P, Brandão ML. 1992. Effects of blockade of 5-HT2 receptors and activation of 5-HT1A receptors on the exploratory activity of rats in the elevated plus-maze. Psychopharmacology (Berl). 107:135–139.
- Musazzi L, Milanese M, Farisello P, Zappettini S, Tardito D, Barbiero VS, Bonifacino T, Mallei A, Baldelli P, Racagni G, Raiteri M, Benfenati F, Bonanno G, Popoli M. 2010. Acute stress increases depolarization-evoked glutamate release in the rat prefrontal/frontal cortex: The dampening action of antidepressants. PLoS One. 5:e8566.
- Pacak K, Palkovitz M. 2001. Stressor specificity of central neuroendocrine responses: Implications for stress-related disorders. Endocr Rev. 22:502–548.
- Patel PD, Lopez JF, Lyons DM, Burke S, Wallace M, Schatzberg AF. 2000. Glucocorticoid and mineralocorticoid receptor mRNA expression in squirrel monkey brain. J Psychiatr Res. 34:383–392.
- Paxinos G, Watson C. 2007. The rat brain in stereotaxic coordinates. New York: Academic Press.
- Pellow S, Chopin P, File SE, Briley M. 1985. Validation of open:closed arm entries in the elevated plus-maze as a measure of anxiety in the rat. J Neurosci Methods. 14:149–167.
- Pryce CR. 2008. Postnatal ontogeny of expression of the corticosteroid receptor genes in mammalian brains: Inter-species and intra-species differences. Brain Res Rev. 57:596–605.
- Quirarte GL, de la Teja IS, Casillas M, Serafin N, Prado-Alcala RA, Roozendaal B. 2009. Corticosterone infused into the dorsal striatum selectively enhances memory consolidation of cued water-maze training. Learn Mem. 16:586–589.
- Quirk GJ, Likhtik E, Pelletier JG, Paré D. 2003. Stimulation of medial prefrontal cortex decreases the responsiveness of central amygdala output neurons. J Neurosci. 23:8800–8807.
- Radley JJ, Gosselink KL, Sawchenko PE. 2009. A discrete GABAergic relay mediates medial prefrontal cortical inhibition of the neuroendocrine stress response. J Neurosci. 29:7330–7340.
- Rodgers RJ, Haller J, Holmes A, Halasz J, Walton TJ, Brain PF. 1999. Corticosterone response to the plus-maze: High correlation with risk assessment in rats and mice. Physiol Behav. 68:47–53.
- Rodrigues SM, LeDoux JE, Sapolsky RM. 2009. The influence of stress hormones on fear circuitry. Annu Rev Neurosci. 32:289–313.
- Roozendaal B, McReynolds JR, Van der Zee EA, Lee S, McGaugh JL, McIntyre CK. 2009. Glucocorticoid effects on memory consolidation depend on functional interactions between the medial prefrontal cortex and basolateral amygdala. J Neurosci. 29:14299–14308.
- Shackman AJ, Salomons TV, Slagter HA, Fox AS, Winter JJ, Davidson RJ. 2011. The integration of negative affect, pain and cognitive control in the cingulated cortex. Nat Rev Neurosci. 12:154–167.
- Singewald N. 2007. Altered brain activity processing in high-anxiety rodents revealed by challenge paradigms and functional mapping. Neurosci Biobehav Rev. 31:18–40.
- Sotres-Bayon F, Quirk GJ. 2010. Prefrontal control of fear: More than just extinction. Curr Opin Neurobiol. 20:231–235.
- Stam R. 2007. PTSD and stress sensitisation: A tale of brain and body: Part 2. Animal models. Neurosci Biobehav Rev. 31:558–584.
- Sullivan RM, Gratton A. 2002. Prefrontal cortical regulation of hypothalamic–pituitary–adrenal function in the rat and implications for psychopathology: Side matters. Psychoneuroendocrinology. 27:99–114.
- Tomazini FM, Reimer A, Albrechet-Souza L, Brandão ML. 2006. Opposite effects of short- and long-duration isolation on ultrasonic vocalization, startle and prepulse inhibition in rats. J Neurosci Methods. 153:114–120.
- Wiedenmayer CP. 2004. Adaptations or pathologies? Long-term changes in brain and behavior after a single exposure to severe threat. Neurosci Biobehav Rev. 28:1–12.
- Willner P, Mitchell PJ. 2002. The validity of animal models of predisposition to depression. Behav Pharmacol. 13:169–188.
- Wright IK, Ismail H, Upton N, Marsden CA. 1991. Effect of isolation rearing on 5-HT agonist-induced responses in the rat. Psychopharmacology (Berl). 105:259–263.